Jo C. Murrell Bristol Veterinary Specialists, Severn Beach, Bristol, UK There is an ever‐increasing understanding of the signs associated with acute and chronic pain as well as ways in which these phenomena can be measured. There is also now a plethora of validated tools to measure acute and chronic pain although the evidence suggests that they have not been widely adopted in general practice. This is unfortunate because unless pain is quantified it is very difficult to treat it effectively without falling into the trap of over or undertreating pain, with both scenarios having a negative impact on the animal. The various methods available to recognize acute pain are discussed below. Table 47.1 provides a summary of the principal advantages and disadvantages of each. Changes in spontaneous behavior form the cornerstone of methods to recognize acute pain across species. Behavioral changes can be used to detect both the sensory (e.g., how much does it hurt? and where does it hurt?) and the emotional component (e.g., depression, anxiety) of pain. The detection of behavioral changes is facilitated if the normal (pain‐free) behavior of the animal is known, therefore pet owners or carers can play a key role in the detection of acute pain. Similarly, animal care staff such as veterinary nurses or technicians who spend a lot of time with the animal can be pivotal to detecting changes in behavior throughout the day and in response to the administration of analgesic drugs. Formal assessment of behavior can be achieved by using an ethogram (a catalog of behaviors) specifically related to pain in the species being observed, however, careful observation of behavior is also sufficient to detect signs of pain. Table 47.1 Summary of the advantages and disadvantages of different methods used to recognize acute pain. Figure 47.1 In dogs, adoption of a “praying posture” is suggestive of abdominal pain. A. Praying position in a dog diagnosed with acute pancreatitis. B. Praying position in a dog that had undergone surgery for a splenectomy. Source: Dr. Sheilah Robertson, with permission. Figure 47.2 In cats, a hunched or “tucked up” posture is suggestive of abdominal pain. This cat had undergone an elective ovariohysterectomy 6 h earlier. Source: Dr. Sheilah Robertson, with permission. Figure 47.3 This horse is pawing at the ground, a behavior that is related to abdominal pain. Source: Dr. Sheilah Robertson, with permission. An important part of behavioral assessment of pain is interaction with the animal, particularly the application of gentle pressure around any wound or painful area in order to detect secondary hyperalgesia and allodynia. This should be done gently so as not to cause the animal more pain, but it is an essential part of behavioral pain assessment and is included as part of many tools used to quantify pain. Changes in facial expression have received a significant amount of attention as a biomarker for acute pain across species. This has largely stemmed from work in neonates which showed that reliable changes in facial expression could be detected during painful interventions such as heel lancing for blood sample collection [3]. The first work in animals was carried out in mice and led to the development of the Mouse Grimace Scale [4]. The detection of pain using facial expression across species largely relies on recognizing changes in facial action units, which are a set of facial muscle movements which correspond to a displayed emotion. Typically, changes in ear position and eye‐opening are included in the scoring system, with other changes being largely species‐dependent. Details of specific grimace scales used to quantify pain are given later in this chapter. Changes in complex patterns of behavior such as burrowing and nest‐making have been used in rats and mice, respectively, as indicators of pain that may be a more sensitive biomarker of affective state or how the animal is feeling about their pain than traditional nociceptive tests. These tests use the principle that rodents are highly motivated to perform these behaviors in their home cage if given the means to do so (i.e., burrowing and nesting substrate); therefore, quantifying an altered willingness to carry out these behaviors offers a means to probe changes in affective state following pain induction and subsequent administration of analgesic drugs. Spontaneous burrowing behavior in rats is reduced by peripheral nerve injury or inflammatory pain [5], an effect that is reversed by the administration of analgesic drugs, showing the utility of this assay in analgesic drug development. Similar studies have been carried out in mice using burrowing behavior [6]. The complexity of nest‐making has been shown to relate to postoperative pain in mice, although the complexity of nest‐making was not restored by treatment with carprofen [7], suggesting that it may not be a good biomarker for efficacy of analgesic treatment. Advantages of using behavior to assess pain are that it may provide direct insight into how the animal is feeling and therefore how painful the animal is. Disadvantages include that it is time consuming to carry out and requires careful observation of the animal. The presence of an observer may also change the behavior of the animals being observed. Questions have also been raised about the objectivity of behavior as a tool to recognize pain. However, behavior can be an objective means depending on how it is measured. Although behavior can be a reliable tool to assess acute pain, a number of confounding factors can interfere with behavioral pain assessment, especially in the immediate postoperative period during recovery from anesthesia. A full bladder can make animals restless and unwilling to settle and this can confound pain assessment. Similarly, it is difficult to behaviorally assess a fear‐aggressive animal, especially because interaction with such animals is often limited. Sedation is a big confounder of behavioral pain assessment; animals that are sedated can appear very comfortable and settled, but it is important to be aware that sedation does not necessarily equate to adequate analgesia. It is advisable to wait until 30–40 min after the end of anesthesia to assess pain when the patient is more rousable and less sedated. Emergence delirium and dysphoria from the over‐zealous use of opioids during anesthesia can also cause behavioral changes such as agitation and vocalization that can be confused with pain. Changes in physiological parameters such as heart rate, blood pressure, respiratory rate, and cortisol have been widely used as a biomarker for acute pain, particularly in farm animal studies where changes in these parameters have been used to assess pain caused by husbandry procedures such as castration [8,9]. Generally, pain can cause an increase in heart rate, blood pressure, and respiratory rate through stimulation of the sympathetic nervous system due to an interaction between pathways that transmit sensory information and the central autonomic nervous system in the brain. Pain also causes activation of the hypothalamo–pituitary–adrenal axis, which is the neuroendocrine stress pathway. Cortisol is one of the end products of this pathway and therefore has been widely used as a biomarker for stress caused by acute pain in farm animals, particularly pain caused by castration in lambs and calves and tail docking in lambs [10]. Another neuroendocrine biomarker of pain that has been studied is β‐endorphin [11]. Blood biomarkers of pain and distress are considered objective biomarkers of pain. However, a big caveat to their use is that neuroendocrine biomarkers are primarily indicators of stress, and although pain is a stressor, other stressors such as handling to collect a blood sample can cause elevations in their concentrations. In horses, anesthesia alone will cause marked elevations in neuroendocrine biomarkers, so that the capacity for further increases due to pain associated with surgery is limited [12]. Cortisol is also subject to circadian rhythms in secretion and there may be a ceiling effect on cortisol secretion. Heart rate, respiratory rate, and blood pressure, although relatively easy to measure, are also considered to be non‐specific for pain. Blood pressure and heart rate may change intraoperatively due to blood loss or the effects of surgical manipulation of nerves such as the vagus nerve, rather than being specific biomarkers of nociception. Similarly, postoperative changes in these parameters may indicate stress caused by factors other than pain. Heart rate variability assesses beat‐to‐beat variability in the timing between one heartbeat and the next [13]. Heart rate variability is used to investigate the balance between sympathetic and parasympathetic nervous system activity, with a decrease in heart rate variability shown with nociception or pain due to a shift in balance toward sympathetic nervous system activity. Heart rate variability data are generally analyzed using frequency domain measures calculated using fast Fourier transformation, such as high‐ and low‐frequency power and the low‐frequency/high‐frequency ratio [13]. Monitors designed to detect intraoperative nociception have been developed for animals based on heart rate variability data, for example, the Parasympathetic Tone Activity (PTA) monitor (MDoloris Medical Systems, Loos, France) [14]. Production parameters such as average daily weight gain have been used to assess pain following castration in calves [10]; however, they are often too imprecise to detect acute changes in pain after castration. Furthermore, weight gain after castration may be negatively influenced by a decrease in testosterone after removal of the testes [15]. The electroencephalogram (EEG) can be recorded from the surface of the head of animals and has been widely used as a measure of acute pain following surgical interventions such as castration. Fig. 47.4 shows EEG recording in an anesthetized deer. Measurement of the EEG relies on recording the summated electrical activity of populations of neurons together with glial cells (for a review see Murrell and Johnson [16]). These electrical potentials can be recorded from the surface of the head using electrodes connected to amplifiers and an EEG recording system, including an analog‐to‐digital converter. The raw EEG signal is recorded in real time, but this can be difficult to interpret and generates a large amount of data in a short space of time. Commonly, fast Fourier transformation is applied to raw EEG data, off‐line at the end of an experiment, changing the signal from the time domain to the frequency domain to produce a power spectrum. Simple descriptors can be derived from the power spectrum, including the median frequency, spectral edge frequency 95%, and total power. EEG changes during nociception are frequently reported as percentage changes in these descriptors compared with an unstimulated baseline period [17,18]. Commonly a change in the level of synchronization of the EEG is described. Desynchronization is characterized as increased high‐frequency activity and decreased power in the low‐frequency bands of the EEG and is associated with an increased level of arousal due to noxious stimuli. Conversely, synchronization refers to an EEG pattern of high‐amplitude, low‐frequency activity. In human studies, the frequency of EEG activity has been denoted by δ (0–4 Hz), θ (4–8 Hz), α (8–12 Hz), and β (> 12 Hz), and the relative amount of activity in each frequency band is reported. These frequency bands also have functionality associated with them in man, and this method has been adopted by some authors investigating EEG in animals [19]. However, it is important to be aware that the division of the EEG into these frequency bands in animals is completely arbitrary and may be inappropriate in animals as compared to humans. Advantages of using the EEG as a marker of nociception in animals include its objectivity and the fact that signals are being recorded directly from the brain, which can be considered the seat of pain perception. Disadvantages include the need to understand the complex nature of the EEG acquisition equipment and the underlying signal‐processing techniques. It can also be difficult to record high‐quality EEG signals in awake animals while anesthesia may interfere with EEG data acquisition depending on the anesthetic agent used [20]. Many of the studies investigating EEG changes with nociception in anesthetized animals have been carried out using halothane as an inhalant anesthetic agent because it is less depressant to the EEG compared with isoflurane or sevoflurane [20–22]. Figure 47.4 This image shows EEG recording in an anesthetized deer. The needle electrodes are placed subcutaneously and attached to pre‐amplifiers (the metal boxes in the foreground of the picture). Source: Dr. Jo Murrell, with permission. Functional magnetic resonance imaging (fMRI) uses blood oxygen level‐dependent imaging (BOLD) to detect changes in blood flow to different regions of the brain. Hemoglobin is diamagnetic when oxygenated but paramagnetic when deoxygenated. This difference in magnetic properties leads to small differences in the magnetic resonance signal of blood depending on the degree of oxygenation. When areas of the brain are activated, such as during application of a noxious stimulus, blood flow to these areas will usually increase, which can be seen on the fMRI image. There are many studies in humans that have investigated which areas of the brain are activated during application of noxious thermal and mechanical stimuli [23]. These studies have led to identification of the so‐called “pain matrix,” the complex pattern of areas of the brain that are activated when a noxious stimulus is processed [24]. However, it is important to be aware that the pain matrix may not be specific to pain and similar areas of the brain may be activated by loud auditory, startling visual, and salient non‐painful mechanical stimuli [25]. This has led to the suggestion that the pain matrix is in fact a saliency detection system [25]. Although there have been numerous studies elucidating path pathways in rats and mice using fMRI, studies using fMRI to detect pain or noxious stimuli in companion and farm animals are currently nonexistent. This is largely because of difficulties associated with training an animal to lie still in the scanner while a noxious or painful stimulus is delivered. Infrared thermography can be used to measure changes in skin surface temperature before and after a noxious intervention such as castration. It is hypothesized that release of hormones such as epinephrine and norepinephrine associated with noxious stimulation causes peripheral vasoconstriction and therefore a decrease in skin temperature that can be quantified using a thermal imaging camera. Eye temperature has also been used as a biomarker for pain, with a decrease in eye temperature noted after castration without local anesthetic in calves, attributed to sympathetically driven changes in tone in the eye capillary beds [26]. Changes in comb temperature have been measured in poultry and the temperature changes in the head, snout, and vulva have been measured in pigs [27]. Although monitoring of skin surface temperature at different sites is non‐invasive and quick to conduct it also has limitations. Thermal imaging cameras are expensive to purchase and the area which has to be measured must be defined and analyzed in a standardized way. Skin surface temperature is affected by stress and disease; therefore, it is a measure that is non‐specific for pain. Accelerometry has been widely used as a measure of acute pain in calves following castration or dehorning [28,29]. They have also been used as a marker of acute postoperative pain after ovariohysterectomy in dogs [30]. Triaxial accelerometers estimate acceleration along the x, y, and z axes, allowing measurement of position relative to the ground, and the direction and speed of movement. Some accelerometers will allow measurement of time spent standing, lying, and walking as well as giving an accurate step count. Advantages of accelerometry are that it is non‐invasive, accelerometers are relatively inexpensive to purchase, and they can record high‐resolution data over a relatively long period of time. Attaching pedometers is also unlikely to influence the behavior of the animal because they are small and lightweight to wear. Step‐counts can also be used as a surrogate marker for pain, measured using pedometers. Like accelerometers, these are inexpensive to purchase, and can be easily attached and used. Fig. 47.5 shows a pedometer attached to a horse’s forelimb. Detection of chronic pain is challenging across all species and is probably more difficult than the detection of acute pain. Behavioral changes can be very subtle and vary according to the species, pain condition, and individual. Many of the techniques used to assess acute pain are not appropriate for the detection of chronic pain in animals or have not been trialed for this purpose. The reason for this may be that, unlike acute pain where established clinical pain models exist (e.g., castration or tail docking), common clinical chronic pain models are lacking. Exceptions to this may be osteoarthritis, Chiari‐like malformation and syringomyelia in dogs, or laminitis in horses, which are relatively well‐characterized clinical models of chronic pain. A summary of the advantages and disadvantages of the various methods to recognize chronic pain that are discussed below is provided in Table 47.2. Changes in behavior associated with osteoarthritis have been characterized in dogs and cats and there is evidence that these behavioral changes can be detected by owners [31,32], although the detected changes in behavior may not always be attributed to osteoarthritis by the owner. Enomoto et al. [31] have developed a checklist for owners of cats which asks questions about whether their cat can jump up normally, jump down normally, the ability of the cat to climb up and down stairs, run, and chase objects. The role of owners in detecting chronic pain caused by osteoarthritis in cats and dogs is emphasized by the importance of clinical metrology instruments in measuring pain caused by this condition as discussed later in this chapter. Chiari‐like malformation and syringomyelia are common conditions in small and toy breed dogs such as the Cavalier King Charles Spaniel. They commonly co‐exist and are believed to cause clinical signs indicative of neuropathic pain such as allodynia or dysesthesia. Dogs appear to dislike being touched over areas of skin around the neck and may not tolerate a neck collar. Phantom scratching, where dogs appear to scratch the air without making skin contact, sometimes while walking may be suggestive of dysesthesia [33]. Chronic laminitis is a painful and debilitating condition in horses that results in severe pain with a neuropathic component [34]. Characteristic behavioral changes associated with laminitis in horses include lameness, which most commonly affects at least two limbs, leaning back onto the heels to take the weight off the painful toe area, and shifting weight between feet when resting. Figure 47.5 A pedometer attached to the forelimb of a horse to measure step count. Source: Dr. Jo Murrell, with permission. Generally, physiological biomarkers are less helpful in the recognition of chronic pain than they are for the recognition of acute pain. Changes in heart rate, respiratory rate, and blood pressure do not persist in chronic pain states and changes in neuroendocrine biomarkers have largely not been assessed in chronic states. An exception may be heart rate variability which has been shown to return to more normal resting conditions when horses with laminitis were treated with analgesics [35]. Table 47.2 Summary of the advantages and disadvantages of different methods used to recognize chronic pain. Visual observation of gait, or lameness scoring is commonly used to assess lameness in companion animals. Techniques to facilitate visual observation of lameness include choosing a surface for observation that is even and flat, observing the animal at a walk and trot, watching the animal both going away from and coming toward you, and when it moves in a circle. Generally, an animal that is lame on the forelimb will shift weight caudally and lift the head on the lame limb, or “nod” the head down on the sound limb. With hindlimb lameness, weight tends to be shifted cranially with the head and neck lowered and extended to offset weight from the hind end. Numerical rating scales and visual analog scales are used to quantify lameness, although visual observation is recognized to be less sensitive at detecting lameness than force plate or kinematic gait analysis techniques [36]. With force plate gait analysis metal plates are mounted on the floor or walkway to measure ground reaction forces. Force plate gait analysis is viewed as the gold standard for evaluation of lameness with peak vertical force (PVF) (maximal force applied during the stance phase) in the vertical axis the most commonly used variable to assess lameness [37]. The vertical impulse (VI) can be derived by calculating the area under the vertical force curve over time. In general, a lame animal has a lower PVF and VI in the lame limb. Although force plate gait analysis is more sensitive than observation to detect lameness there are limitations to this technique. These include an inability to measure stride or step length, the need for the animal to transverse the force plate at a constant velocity, the need for a long walkway, multiple trials, and the complexity of software and data analysis. Kinematic gait analysis quantifies the positions, velocities, acceleration, and deceleration, and angles of anatomical structures in space. Generally, kinematic gait analysis systems use colored or reflective markers that identify specific anatomical landmarks, and these markers are tracked as the animal moves by a series of cameras. Outputs from kinematic gait analysis include displacement, which is the distance recorded when a marker changes position; angular velocity, which is the speed at which this change occurs; and range of motion, which is calculated from the displacement of a specific joint. Kinematic gait analysis has been applied to dogs, horses, and chickens; in the latter, it was used to quantify gait abnormalities associated with lameness in broiler chickens and identify evolutionary gait differences between broiler chickens and jungle fowl [38]. Limitations of kinematic gait analysis include the potential for skin movement altering marker placement, accuracy, and repeatability of marker placement, as well as the complexity of software and data analysis. In addition to its role in acute pain assessment, accelerometry has been widely used to assess pain associated with osteoarthritis in cats [39] and dogs [40], and to document a treatment effect when animals are administered an analgesic [41]. Advantages of accelerometry for chronic pain are similar to those for acute pain. A number of different tools have been developed to try and measure acute pain across different species. However, where data are available, it shows that adoption of these tools in general practice is low. In a 2021 study in Australia, only 14% of respondents in small animal practices used validated pain scales to quantify pain [42]. This finding is also supported by an older study in the UK where only 14% of small animal general practitioners were using pain assessment tools [43]. Interestingly the percentage of respondents using pain assessment tools was significantly higher (60–80%) among respondents who were members of the Association of Veterinary Anaesthetists [43]. There are a number of excellent reasons to use tools to try and quantify pain across different species. Unless you use a pain assessment tool it is difficult to know what level of pain the animal is experiencing and therefore treat it appropriately with analgesics. It is also difficult to objectively assess the response to analgesic drug administration to determine whether it has been adequate or whether more analgesic drugs are required. Tools also help with the actual assessment of pain, because using a structured instrument to assess pain aids in coming to some sort of conclusion about whether the animal is experiencing pain or not. They also help standardize the process of pain assessment between individuals in a clinical setting so that when more than one person is assessing pain in an individual animal interobserver variability should be reduced.
47
Recognition and Quantification of Acute and Chronic Pain
Introduction
Recognition of acute pain
Changes in behavior
Characteristic changes in behavior associated with different types of pain have been described. Behavioral changes that have been described in dogs with abdominal pain include a hunched appearance and adoption of a praying position where the animal appears to stretch out with the front legs positioned near the ground and the back legs in a more upright position (Fig. 47.1, Video 47.1). Dogs with abdominal pain do not look comfortable and relaxed and often cannot sleep, showing an inability to settle and a tense abdomen when palpated. Cats with abdominal pain also show a tense abdomen on palpation and a hunched appearance (Fig. 47.2). Behavioral changes have also been noted in cats by owners in the three days following surgery for ovariohysterectomy and include decreased playfulness, increased time spent sleeping, and an altered way of moving, suggestive of abdominal pain [1]. Horses with colic may be restless, depressed, show flank watching, weight shifting, kicking of the abdomen, pawing at the ground (Fig. 47.3), and stretching [2].
Method to recognize acute pain
Advantages
Disadvantages
Behavior
Can provide a good insight into how the animal “feels” about their pain
“Gold standard” measure of acute pain
Can be time consuming to collect data
Presence of an observer may influence the behavior of an animal
Risk of confounding factors changing the behavior of an animal
Physiology
Objective means to measure pain
Many biomarkers are related to stress and are not pain‐specific
Some biomarkers are invasive to measure e.g., blood samples for serum cortisol
Electroencephalogram
Direct assessment of brain activity may provide insights into pain perception
Objective means to measure pain
Generates a large amount of data that can be difficult to interpret
Requires knowledge of data acquisition and signal‐processing techniques
Anesthesia can facilitate a high signal‐to‐noise ratio for the data but may also influence the recorded data
Functional magnetic resonance imaging
Direct assessment of brain activity may provide insights into pain perception
Changes in brain activity may not be specific to pain
Currently requires anesthesia for pain studies in animals
Requires access to an appropriate scanner
Infrared thermography
Quick to carry out
Non‐invasive
Skin surface temperature can be affected by stress and is not specific to pain
Accelerometry
Accelerometers are inexpensive to purchase
Easy to use
Placing them on the animal is unlikely to change the behavior of the animal
Generates a large amount of data
Activity may be increased for reasons that are not specific to pain e.g., during administration of potent opioids to horses
Factors confounding behavioral assessment of acute pain
Physiological parameters
Electroencephalogram
Functional magnetic resonance imaging
Infrared thermography
Accelerometry and step‐counts
Recognition of chronic pain
Changes in behavior
Physiological parameters
Method to recognize chronic pain
Advantages
Disadvantages
Behavior
Can provide a good insight into how the animal “feels” about their pain
“Gold standard” measure of chronic pain
Can be time consuming to collect data
Presence of an observer may influence the behavior of an animal
Risk of confounding factors changing the behavior of an animal
Physiology
Objective means to measure pain
Less helpful in the assessment of chronic compared to acute pain
May be invasive
Force plate gait analysis
Objective means to measure pain associated with lameness
Cannot measure step or stride length
More useful when the animal has a single‐limb lameness
Requires an understanding of the complex software required for data analysis
Kinematic gait analysis
Objective means to measure pain associated with lameness
Requires an understanding of the complex software required for data analysis
Markers on the body can shift in position rendering data collection inaccurate
Accelerometry
Accelerometers are inexpensive to purchase
Easy to use
Placing them on the animal is unlikely to change the behavior of the animal
Generates a large amount of data
Activity may be increased for reasons that are not specific to pain e.g., during administration of potent opioids to horses
Visual observation of gait
Force plate gait analysis
Kinematic gait analysis
Accelerometry
Quantification of acute pain
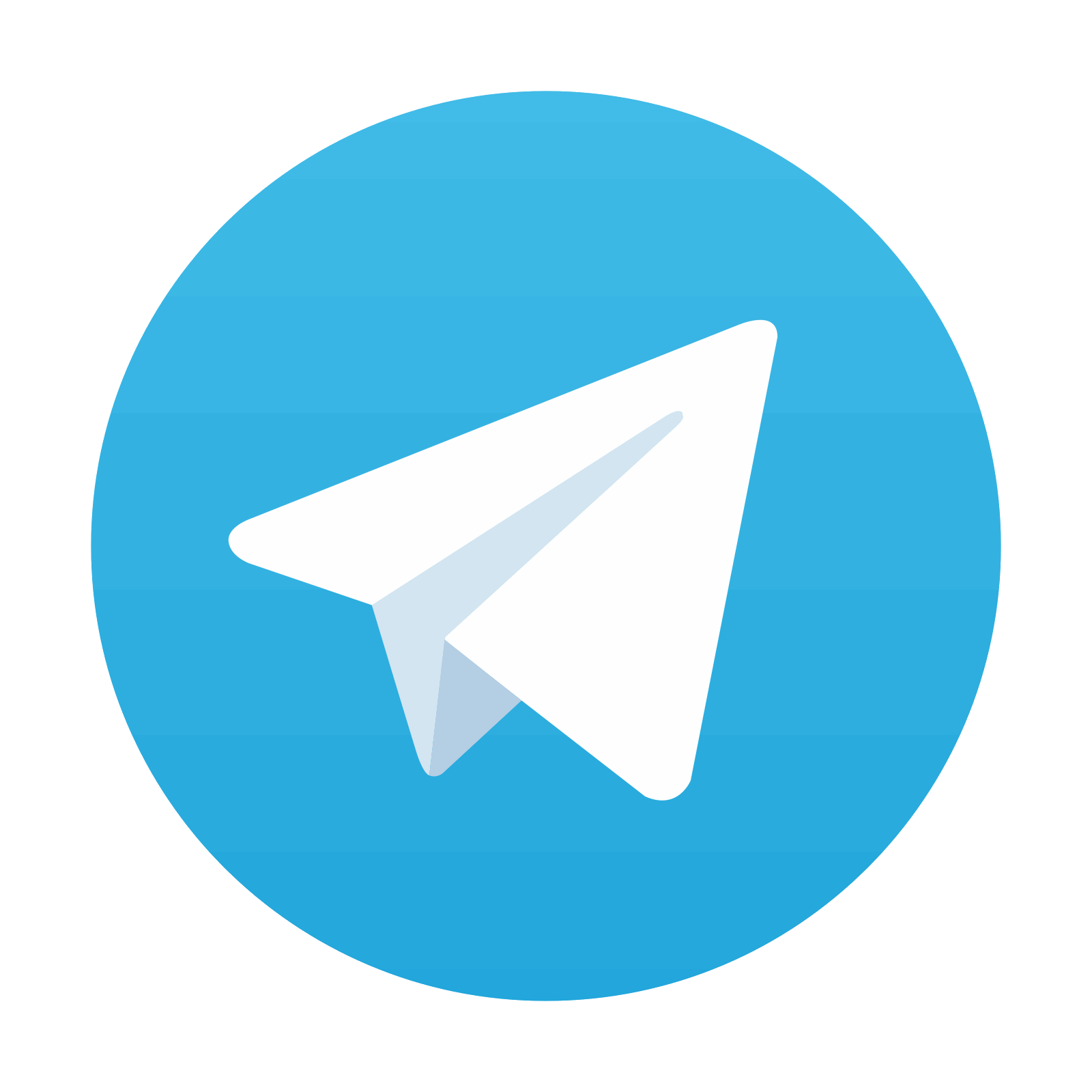
Stay updated, free articles. Join our Telegram channel

Full access? Get Clinical Tree
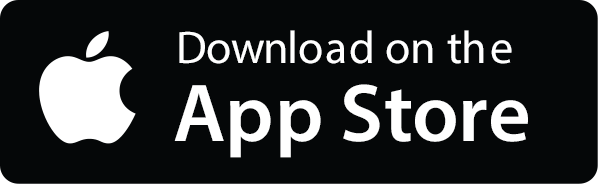
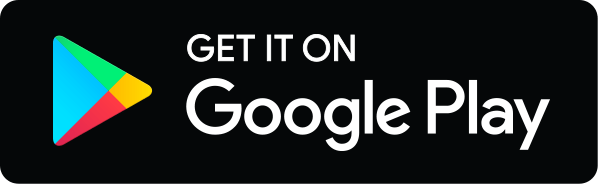