13.1 Introduction
Rabies is a major zoonotic disease, which remains a serious world public health problem. It is one of the most recognizable zoonosis and has been well known for more than 4300 years (Takayama, 2005). While rabies has been controlled throughout most of the developed world, it remains a significant burden in developing countries, causing a large number of animal and human deaths (Wilde and Lumlertdacha, 2011). According to WHO estimates, 55,000 human deaths are reported worldwide and more than 10 million people undergo post-exposure prophylaxis every year. Most human cases occur in the developing countries of Asia and Africa, where canine rabies is endemic (Fu, 1997). In more developed countries, human rabies has dramatically declined over the past 60 years as a direct consequence of routine vaccination of pet animals. However, rabies in wildlife has emerged as a major threat. Despite extensive investigations over more than 100 years, the pathogenetic mechanisms by which infection of street rabies virus (RABV) results in neurological diseases and death in humans are not well understood. Many papers have summarized studies on rabies pathogenesis (Dean et al., 1963; Ritossa and D’Agostino, 1967; Dierks, 1979; Dietzschold et al., 2005, 2008; Hooper, 2005; Jackson, 2006). In this chapter, we briefly describe our current understanding of rabies pathogenesis, the use of RABV as a neuronal tracer and molecular diagnosis for the disease.
RABV has a bullet-shaped structure of about 75 nm×200 nm and this RNA virus contains a negative-stranded RNA genome of about 12 kb, which encodes five proteins in the order of nucleoprotein (N), phosphoprotein (P), matrix protein (M), glycoprotein (G) and the RNA-dependent RNA polymerase (RdRp, also termed L). The N protein encapsulates the newly synthesized RNA, switching the virus from transcription to replication (Lawson et al., 1991). The P protein is important not only for viral transcription and replication but also for interaction with cellular proteins during axoplasmic transport (Lawson et al., 1991). The M protein is located beneath the viral membrane and bridges the nucleocapsid and the lipid bilayer and is involved in viral assembly and budding (Lenard, 1996). The G gene produces a single transmembrane G protein, which is assembled as a trimeric spike (Lawson et al., 1991). This G protein is responsible for the initial binding during infection of susceptible cells (Lawson et al., 1991). The L gene encodes a polymerase for viral RNA transcription and replication.
RABV is a highly neurotropic virus that spreads along neural pathways and invades the central nervous system (CNS), where it causes an acute infection. Most studies on rabies pathogenesis have used experimental animal models infected with laboratory-adapted strains, although the events in these models may not closely mimic the disease under natural conditions (Morimoto et al., 1998, Suja et al., 2011). There are a number of sequential steps that occur after peripheral inoculation of the virus from an animal bite, which is the most common mechanism of transmission (Jackson, 2002, 2006). The steps include replication in peripheral tissues, spread along peripheral nerves and the spinal cord to the brain, dissemination within the CNS, and centrifugal spread from the CNS along nerves to various organs, including the salivary glands (Fig. 13.1).
13.2 Earliest Events at the Site of Exposure
Under natural conditions, humans and animals may experience long and variable incubation periods following a bite exposure, usually lasting for 20 to 90 days (Smith et al., 1991). However, incubation periods shorter than 20 days and longer than 90 days have been reported (Smith et al., 1991). The biologic bases for the variable incubation periods are unclear, but a number of factors may be responsible, including the density of RABV receptors in affected tissues, the degree of innervation in tissues in different anatomical locations, the quantity of virus inoculated and the biological properties of RABV variant (Ray et al., 1995). Many studies have been performed to determine the events during the incubation period and viral spread following the bite exposure. For example, Baer et al. (1968) performed experiments involving amputation of the tail or leg of an animal proximal to the site of inoculation or neurectomy of sciatic nerve. The results clearly demonstrated that RABV moves along peripheral nerves to the CNS in a time-dependent manner. Studies using street RABV supported the idea that the virus remains at or near the site of entry for most of the long incubation period (Baer and Cleary, 1972).
Fig. 13.1. The sequential steps involved in RABV spread from the bite site to the CNS.
There has been speculation that RABV may infect, replicate and subsequently persist in vivo in macrophages (Ray et al., 1995), but the extent of viral replication in macrophages at the site of infection/injection has not yet been clearly demonstrated in animal models. The best experimental example to date was in striped skunks using a Canadian isolate of street RABV by Charlton et al. (1997), where they showed the presence of viral genomic RNA in the inoculated muscle, but not in either spinal ganglia or the spinal cord for up to 62–64 days. Immunohistochemistry supported infection of extrafusal muscle fibres and occasional fibrocytes at the site of inoculation. However, the importance of RABV infecting muscle fibres to gain access to peripheral nervous system and its relevance in pathogenicity is still being debated. Bats produce more superficial bites and muscle infection is likely not important in infection with bat RABV variants (Feder et al., 1997).
13.3 RABV Receptors and Virus Entry
RABV enters the nervous system via a motor neuron through the neuromuscular junction (NMJ), or a sensory nerve through nerve spindles (Murphy et al., 1973). The viral G protein is responsible for the attachment of the virus to target cells (Lafon, 2005). In 1982, the first identified receptor for RABV entry was nicotinic acetylcholine receptor (nAChR) by Lentz and coworkers, who showed that RABV bound to mouse diaphragms at the location of nAChR shortly after the immersion of mouse diaphragms in a suspension of RABV (Lentz et al., 1986; Lentz, 1990). RABV antigen was found to co-localize with the nAChR in infected cultured myotubes from chicken embryos. It is evident from these studies that the distribution of viral antigen at the NMJ corresponds to the distribution of nAChRs. Pre-treatment of myotubes with either the irreversible binding nicotinic cholinergic antagonist α-bungarotoxin or the reversible binding d-tubocurarine reduced virus infection. Also, Tsiang et al. (1986) showed the inhibitory effect on RABV infection on rat myotubes in vitro by the pre-treatment of α-bungarotoxin. These research insights provide enough evidence that the RABV virus binds to nAChRs at the NMJ. Also, it has been established that the viral G protein is involved in attachment and entry into the target cells. Further investigation of RABV G protein binding to the nAChR suggests that both RABV and neurotoxins bind to residues 173–204 of the α1-subunit of the nAChR. Also, the highest-affinity virus-binding residues of the α1-subunit of the nAChR have been mapped to 179–192 (Lentz, 1990). In order to map the viral determinants for attachment and entry, Bracci et al. (1988) raised monoclonal antibodies against a peptide containing residues 190–203 of the RABV G protein. The monoclonal antibody inhibited the binding of the RABV G protein and α-bungarotoxin to the nAChR. These studies have provided strong evidence that RABV binds to nAChRs at the NMJ. Finally, Lentz et al. (1982) showed that the binding of RABV to AChRs, which is present in high concentrations at the NMJ, would localize and concentrate the virus and facilitate subsequent uptake and transfer of virus to peripheral motor nerves.
In addition to nAChR, other molecules have been proposed as RABV receptors. Thoulouze et al. (1998) reported that RABV may also bind to the neural cell adhesion molecule (NCAM), which is present in presynaptic membranes (Lafon, 2005). Incubation of susceptible cells with RABV decreased surface expression of NCAM. RABV infection could be inhibited when the NCAM receptor was blocked with heparan sulfate and by polyclonal or monoclonal antibodies directed against the NCAM receptor (Thoulouze et al., 1998). Soluble NCAM can also neutralize RABV infection. In addition, significantly less RABV antigen was found in various brain regions in NCAM receptor-deficient than in wildtype mice, indicating that viral spread was less efficient without the NCAM receptor. However, lack of NCAM receptor in vivo only mildly delayed the death of mice. Since the NCAM receptor is localized in presynaptic membranes, it is well-positioned for RABV internalization by receptor-mediated endocytosis into vesicles (Lafon, 2005). The low-affinity p75 neurotrophin receptor (p75NTR) has also been suggested to be an RABV receptor (Tuffereau et al., 1998). Most cell lines of non-neuronal cell origin, including BSR cells, are not permissive for street RABV infection. However, BSR cells with stable expression of p75NTR were able to bind soluble RABV G protein. When p75NTR-deficient mice were infected intracerebrally with challenge virus standard, similar clinical features of disease and pathologic changes were observed in the brain as in mice expressing p75NTR (Jackson and Park, 1999). Tuffereau et al. (1998) performed further studies in cultured adult mouse dorsal root ganglion neurons and reported that, although p75NTR is a receptor for soluble RABV G protein in transfected cells of heterologous systems, a RABV G protein interaction is not necessary for RABV infection of primary neurons (Tuffereau et al., 2007). p75NTR is not present at the NMJ and it is mainly present in the dorsal horn of the spinal cord, suggesting that it could be involved in trafficking of RABV by a sensory pathway (Lafon, 2005). It may also play an important role in the retrograde transport of RABV by forming an RABV–p75NTR complex that is transported into the cell in endocytic compartments, possibly following caveolae transcytosis.
Once RABV binds to presumptive receptor(s), virus entry is mediated by endocytosis. Studies performed in chick spinal cord muscle co-cultures show the co-localization of CVS strain of RABV and AChR tracers at the NMJ, which provides evidence that the NMJ is the major site of virus entry into neurons (Lewis et al., 2000). Subsequently, co-localization with endosome tracers indicated that the virus resides in an early endo-some compartment. There is also supporting ultrastructural evidence that RABV particles enter nerve terminals by endocytosis (Iwasaki and Clark, 1975; Charlton et al., 1996). The acidic environment of the endo-some triggers fusion of the viral membrane with the endosome membrane, which allows the viral nucleocapsid to escape into the cytoplasm. However, it has not yet been resolved whether the viral uncoating actually takes place in nerve terminals or in the cell body (perikaryon) after transport in the axon.
Although RABV infection with fixed strains is restricted to a small number of cell types in vivo, fixed viruses can infect a much larger variety of cell types in vitro (Reagan and Wunner, 1985). There is evidence that carbohydrate moieties, phospholipids, highly sialylated gangliosides and other membrane-associated proteins might contribute to the cellular membrane receptor structure for RABV (Superti et al., 1984; Conti et al., 1986).
13.4 RABV Spread
Once RABV enters the nervous system, it is transported in the fibres by centripetal spread within motor and perhaps also sensory axons of peripheral nerves. Colchicine, a microtubule-disrupting agent active for tubulin-containing cytoskeletal structures, is an effective inhibitor of fast axonal transport in the sciatic nerve of rats (Tsiang, 1979). When colchicine was applied locally to the sciatic nerve, propagation of RABV was prevented, which provides strong evidence that RABV spreads from sites of peripheral inoculation to the CNS by retrograde fast axonal transport. Using human dorsal root ganglia neurons Tsiang et al. (1991) showed that viral retrograde transport occurs at a rate of between 50 and 100 mm/day. There is evidence that the RABV P protein interacts with dynein light chain 8 (LC8) (Jacob et al., 2000; Raux et al., 2000). This led to speculation that RABV P protein–dynein interaction may be of fundamental importance in axonal transport of RABV. However, deletions of the dynein light chain-binding region of recombinant RABV demonstrated only minor effects on viral spread after peripheral inoculation (Mebatsion, 2001; Rasalingam et al., 2005). Mazarakis et al. (2001) have demonstrated that RABV G protein-pseudotyped lenti-virus (equine infectious anaemia virus)-based vectors enhance gene transfer to neurons by facilitating retrograde axonal transport. Hence, RABV G protein may play a more important role than the P protein (Mazarakis et al., 2001).
Viral spread within the CNS has been studied extensively in animal models. Once the virus invades the CNS neurons (often in the spinal cord) in rodent models there is rapid dissemination of RABV along neuroanatomical pathways by fast axonal transport. Studies using stereotaxic brain inoculation in rats show the axonal transport of virus in the neuroanatomical structures (Gillet et al., 1986) and it is supported by studies in which the administration of colchicine inhibited virus transport within the CNS (Ceccaldi et al., 1989, 1990). Tsiang et al. (1989) showed the anterograde fast axonal movement of RABV is in the range of 100–400 mm/day in cultured rat dorsal root ganglia neurons. However, it is contradictory to studies performed in rhesus monkeys, which indicate that the spread of RABV occurs exclusively by retrograde axonal transport (Kelly and Strick, 2000). Studies performed with G-deficient recombinant RABV showed limited spread in the brains of mice after intracerebral inoculation (Etessami et al., 2000). Therefore, it is clear that the G protein is necessary for trans-synaptic spread of RABV from one neuron to another. In a skunk model, it has been found that most viral budding occurs on synaptic or adjacent plasma membranes of dendrites, with less prominent budding from the plasma membrane of the perikaryon and occasionally budding freely into the intracellular space (Charlton and Casey, 1979). Also, the presence of virus partially engulfed in the invaginated membrane of an adjacent axon terminal indicates transneuronal dendroaxonal transfer of virus.
Experiments involving the inoculation of CVS in the footpad of mice shows there was early involvement of neurons in the brainstem tegmentum and deep cerebellar nuclei (Jackson and Reimer, 1989). Subsequently, the virus spreads to involve cerebellar Purkinje cells and neurons in the diencephalon, basal ganglia and cerebral cortex. RABV spreads to the hippocampus relatively late after peripheral inoculation (Carbone et al., 1987). RABV predominantly infects pyramidal neurons of the hippocampus, with relative sparing of neurons in the dentate gyrus in adult mice (Jackson and Reimer, 1989). Although the basis of specific cell tropism is still unclear, Gosztonyi and Ludwig (2001) speculated that the cell selectivity can be explained if N-methyl-D-aspartate (NMDA) NR1 receptors are involved as RABV receptors, because RABV spreads only by retrograde (not by anterograde) fast axonal transport. Therefore, the virus cannot infect dentate granule cells by the perforant path and mossy fibres from CA3 that predominantly have α-amino-3-hydroxy-5-methyl-4-isoxazole propionate (AMPA) and kainate receptors rather than NMDA receptors. In experimentally infected skunks, it has been observed that skunk RABV is highly neurotropic and infects Bergmann glia in the cerebellum more prominently than Purkinje cells (Jackson et al., 2000). Initial infection was present in the lumbar spinal cord and transit to the brain occurred via a variety of long ascending and descending fibre tracts, including rubrospinal, corticospinal, spinothalamic, spino-olivary, vestibulospinal/spinovestibular, reticulospinal/spinoreticular, cerebellospinal/spinocerebellar and dorsal column pathways (Charlton et al., 1996).
13.5 RABV as an Advanced Tracer for Neural Circuits
RABV acts as a highly effective trans-neuronal tracer (Ugolini, 1995, 2008; Kelly and Strick, 2000; Callaway, 2008; Lyon, 2012). As noted above, the viral G protein serves as the ligand necessary for infection and transneuronal spread (Conzelmann et al., 1990; Gaudin et al., 1993; Etessami et al., 2000). And, because the G protein receptor appears to be localized to presynaptic nerve terminals (Lafon, 2005), injections of RABV directly into the brain infect neurons through axon terminals and RABV is transported in a retrograde direction to replicate in the soma, then invade postsynaptic terminals in order to spread retrogradely through additional neurons (Kelly and Strick, 2000; Callaway, 2008; Ugolini, 2008; Lyon, 2012).
Unlike traditional non-viral retrograde neuronal tracers, neurons are very well labelled by RABV, allowing for detailed morphological reconstruction following immunohistochemical staining for the RABV N protein (Nassi et al., 2006). However, in order to extract meaningful data, the spread of the virus needs to be prevented from infecting the entire CNS. One approach for controlling RABV spread is to limit the survival time after injection. The CVS strain, for example, can be limited to two synapses using a survival time of 72 hours (Callaway, 2008). This approach has been used to identify several previously unknown di-synaptic pathways in monkeys (Nassi et al., 2006; Nassi and Callaway, 2009; Lyon et al., 2010; Lyon and Rabideau, 2012). Therefore, controlled use of fixed strains of RABV can be an effective tool.
The spread of RABV can also be restricted by altering the genetic expression of the G protein (Etessami et al., 2000). Wickersham and colleagues (2007a) removed the G gene from the SAD-B19 vaccine strain and replaced it with the gene for enhanced GFP (Fig. 13.2). Moreover, during production of this G-deleted (ΔG) RABV (SADΔGGFP), the G protein was supplied so that it could be incorporated into the viral envelope (but not into the genome). Thus, the virus acts as a monosynaptic retrograde tracer, infecting axon terminals at the site of brain injection, transporting retrogradely to the soma where it replicates and fills the soma and dendrites completely with GFP (Nassi and Callaway, 2007; Wickersham et al., 2007a; Connolly et al., 2012), but is unable to spread to presynaptically connected neurons because the G gene is missing (Fig. 13.2B). Variants of this virus are now used to deliver many different genes, from red or blue fluorescent proteins, to genetically encoded Ca2+ indicators for imaging cell activity, to channel-rhodopsin-2 for light activation, and many others (Osakada et al., 2011).
An additional modification to the SADΔG-GFP was made to enable highly specific, genetically targeted infection of a single neuron. Wickersham and colleagues (2007a) replaced the G protein in the membrane envelope with the envelope A protein (EnvA) from the avian sarcoma and leukosis virus, a process known as pseudotyping (Fig. 13.2A). EnvA limits infection to cells that express the TVA receptor, a protein found in birds but not mammals (Barnard et al., 2006). As developed by Wickersham et al. (2007b) for the resulting pseudotyped virus, EnvA-SADΔG-GFP, to work as a ‘single-cell tracer’ three genes must be delivered to the target neuron prior to delivering the virus (Fig. 13.2C–F): (1) the gene for TVA, so that the virus can enter the soma; (2) the gene for RABV G protein, to allow the virus spread to presynaptically connected neurons; and (3) the gene for a marker protein such as mCherry for cell identification. Following a 24-hour period to allow for expression of these three proteins, the EnvA-SADΔG-GFP virus is injected near the transfected neuron. The transfected neuron, which is already expressing mCherry (Fig. 13.2E), will become infected and then over the next day begin to express GFP (Fig. 13.2F); after a few additional days presynaptically connected neurons from all over the brain will also become infected and express GFP as well (Fig. 13.2D, F). The virus will not spread beyond these directly connected presynaptic cells since the gene for RABV G protein was not delivered to these cells. In vivo, this technique can label inputs originating throughout the brain providing unprecedented global detail of the circuits involving a single neuron (Marshel et al., 2010; Rancz et al., 2011).
13.6 Spread from the CNS
The viral centrifugal spread from the CNS to peripheral sites including salivary glands along neuronal routes is essential for efficient transmission of RABV among its natural hosts. Anatomically, salivary glands receive parasympathetic innervation by the facial (via the submandibular ganglion or Langley’s ganglion in some animals) and glossopharyngeal (via the optic ganglion) nerves, sympathetic innervation via the superior (or cranial) cervical ganglion and afferent (sensory) innervation (Emmelin, 1967). Experimental studies using street RABV in dogs and foxes involving unilateral excision of a portion of the lingual nerve and the cranial cervical ganglion resulted in very low viral titres in denervated salivary glands compared with contralateral salivary glands (Dean et al., 1963). Also, it is evident from the studies by Charlton et al. (1983) that the widespread infection of salivary gland epithelial cells is a result of viral spread along multiple terminal axons rather than spread between epithelial cells. Experimental rabies infection of non-nervous tissues in skunks and foxes by Balachandran and Charlton (1994) shows the presence of RABV antigen in the apical region of mucous acinar cells. Also, the ultrastructural studies show the presence of viral proteins in tissues at variable proportion, and also suggested that the viral titres in salivary glands may be higher than in CNS tissues.
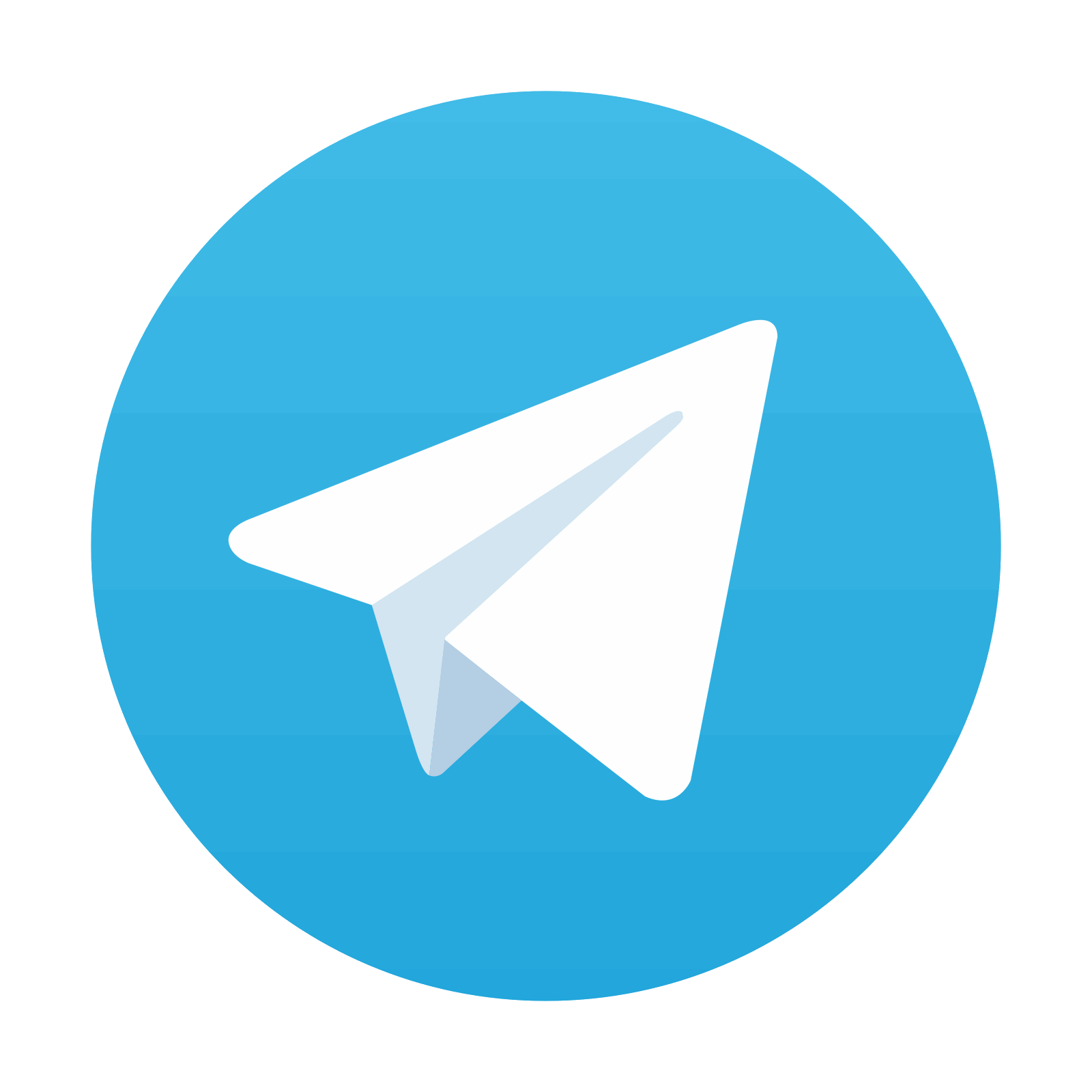
Stay updated, free articles. Join our Telegram channel

Full access? Get Clinical Tree
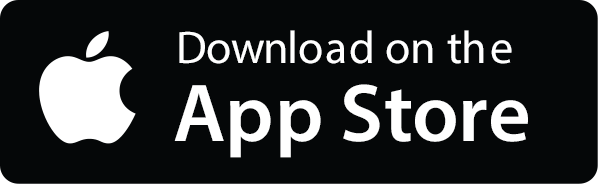
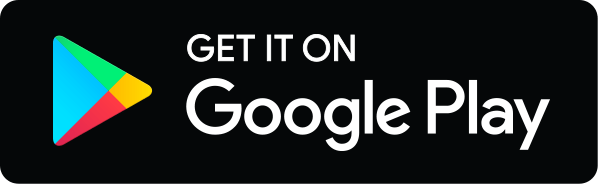