CHAPTER 4 Although protozoa are single-celled organisms, they are not necessarily primitive. They have much greater complexity than other unicellular life-forms, such as bacteria. Like multicellular animals and plants, their DNA is mostly packaged into chromosomes within a nucleus. They have many subcellular structures similar to those in metazoan cells but, in addition, each protozoan species possesses specialised organelles that enable it to live and function as an independent organism within its own ecological niche. Most protozoa are free-living although many coexist with animal hosts. This chapter focuses on those that are potentially pathogenic. Many others are benign (‘commensals’) or even beneficial to their host, e.g. the symbionts that digest cellulose in the rumen or caecum of herbivores. Practical tip box 4.1 There is a great diversity of pathogenic protozoa. Those of greatest economic, welfare or zoonotic significance are covered below, but many others are encountered less frequently or are yet to be recognised as pathogens. A simplified classification chart (see Figure 4.1) makes a useful framework for understanding veterinary protozoology as it reflects differences in structure, behaviour and life-cycle, which, in turn, often determine disease processes. Figure 4.1 The major groups of protozoan parasites of veterinary importance. One of the most obvious features separating the major protozoan groups is the way they move, as summarised in Table 4.1. Table 4.1 Some important characteristics of major protozoan groups Ciliates (see Figure 4.2) can be recognised by the thousands of tiny hairs (‘cilia’) covering the body surface. To propel the organism along, these bend in coordinated ripples (like Mexican waves round a sports stadium). Figure 4.2 Balantidium trophozoite. Redrawn after Cheng, 1986 with permission of Elsevier. Amoebae are amorphous jelly-like blobs (see Figure 4.3) that progress by pushing out a finger-like protrusion (‘pseudopodium’) which enlarges as cytoplasm flows into it. Amoebae need a suitable substrate for active movement. Figure 4.3 Entamoeba trophozoite. Redrawn after Jeanne Robertson from Roberts and Janovy Jr, 1996 with permission of McGraw-Hill Education. The flagellates, in contrast, are strong swimmers employing one or more long contractile fibres (‘flagellae’ – see Figure 4.4) that flex in a whiplike fashion. They are well suited for life in blood or other body fluids. Figure 4.4 Giardia trophozoite. Redrawn after William Ober from Roberts and Janovy Jr., 1996 with permission of McGraw-Hill Education. The intracellular life-cycle forms of apicomplexans are immobile but the forms that leave one host cell to find another, such as the Toxoplasma merozoites illustrated in Figure 4.5, have sleek, crescent-shaped bodies that appear to glide along a spiral trajectory. This effect is achieved by intracellular contractile microfilaments that induce subtle alterations along the body surface. These propel the organism along an excreted slime trail in a manner analogous to the progress of a snail. Once a suitable host cell is located, penetration is achieved by means of a specialised structure, the ‘apical complex’. Figure 4.5 Schizont: SEM (with artificial colouring) showing merozoites (pink) within host cells (blue). Reproduced with permission of D.J. Ferguson. Protozoa feed mainly on particulate material. The cell membrane indents and folds slowly over, thereby entrapping a small quantity of food and drawing it into the cell. This process is known as pinocytosis or phagocytosis, depending on the size of the particle (in ascending order). In ciliates, food particles are directed by the action of cilia towards the base of a funnel-like structure (the ‘cytostome’). When sufficient food has accumulated in this, a vacuole forms which is engulfed into the cytoplasm. Many parasitic protozoa are also able to absorb liquid nutrients and in some cases this may be their main source of nourishment. The feeding stage in a protozoan’s life-cycle is called a ‘trophozoite’. For many species this is the only form that exists, but others have a succession of stages with different functions, appearances and, of course, names. Trophozoites adapted to a parasitic existence are often vulnerable to unfavourable conditions in the external environment. Those of many species, therefore, secrete a protective wall around themselves to form a resistant ‘cyst’ prior to leaving their host. Passage from host to host can take place in many ways, ranging from passive transfer of trophozoites or cysts (by faecal-oral transfer, for example) to transmission of various life-cycle stages by arthropod vectors or the ingestion by carnivores of organisms within intermediate hosts. Extra information box 4.1 All protozoa reproduce asexually (i.e. by mitosis), although many are able to exchange genetic material within the species. Ciliates, for example, have both a macro- and a micronucleus (see Figure 4.2) and occasionally come together (‘conjugate’) to switch micronuclei. The apicomplexans have complex life-cycles, which, in addition to asexual divisions, also include a sexual phase during which male and female gametes are formed by meiotic division. In some genera, the fertilised cell generates a protective wall to become an ‘oocyst’ (see Figure 4.6). Figure 4.6 Eimeria oocysts: unsporulated (left); sporulated (right). Based on Roberts and Janovy Jr, 1996. Reproduced with permission of McGraw-Hill Education. Asexual reproduction can occur in different ways depending on species and life-cycle phase: The ciliates are generally harmless commensals. They are easily recognised by the hairs (cilia) covering the body, the funnel-shaped depression leading to the mouth (cytostome) and the kidney-shaped macronucleus (see Figure 4.2). The small micronucleus is less obvious. Balantidium and similar genera occur in the lumen of the large intestine of pigs, cattle, primates and some other animals. The trophozoites are about 80 μm long and may become invasive if the intestinal mucosa is damaged by another pathological process (see Figure 4.7). This process can lead to ulceration and dysentery in pigs and primates (including humans). Figure 4.7 Balantidium trophozoites on eroded surface of large intestine. Infections spread by excretion of cysts in host faeces. The cysts are slightly smaller than the corresponding trophozoite. They are thick-walled, but this does not obscure the macronucleus which provides a useful diagnostic feature (see Figure 4.8). Human and canine infections are usually derived from pigs. Figure 4.8 Balantidium cyst: note macronucleus in upper part of cyst. Amoebic infections are often caused by opportunistic free-living aquatic species that take advantage of chance encounters with susceptible hosts (such as humans in a contaminated swimming pool). One species, Entamoeba histolytica, is a true parasite transmitted by the faecal-oral route. It provokes a disease called amoebic dysentery in primates and can pass from humans to dogs and cats. It occurs more commonly in warmer climates. Faecal smears from infected patients may contain motile amoebae (see Figure 4.3) or small (10 μm) cysts containing four nuclei (see Figure 4.9). Autopsy will reveal deep ulcers in the large intestine and abscesses in the liver. Figure 4.9 Entamoeba cyst. Redrawn after Jeanne Robertson from Roberts and Janovy Jr, 1996 with permission of McGraw-Hill Education. The flagellates are those protozoa propelled by one or more whiplike contractile fibres. Most are commensals or symbionts although some are dangerous pathogens. It is convenient to divide the latter into the haemoflagellates (see Section 4.5.1) that live within body tissues, particularly blood and lymph, and those that do not penetrate into the tissues but remain within the lumen of the alimentary tract, uterus or prepuce (see Section 4.5.2). The haemoflagellates include two major genera: Trypanosoma and Leishmania, both of which are of greatest importance in tropical and subtropical climates. Both genera have indirect life-histories that include a succession of morphologically different life-cycle stages. There is little purpose in describing those that occur in the insect vector as they currently have little diagnostic application, but the two forms found in the mammalian host are: Figure 4.10 A Trypanosoma trypomastigote surrounded by red blood cells. Figure 4.11 A Leishmania amastigote (arrowed) in the cytoplasm of a host cell. Redrawn after IPZ, S. Ehrat from Deplazes et al., 2013 with permission of Enke Verlag. Trypanosomes are responsible for serious disease in humans (sleeping sickness in Africa and Chagas disease in parts of Latin America). They also impose severe constraints on agricultural production throughout large areas of sub-Saharan Africa, inflicting death and chronic disease on both food-providing ruminants and the draught animals needed to cultivate crops and to carry produce to market. Trypomastigotes of Trypanosoma are mostly around 20 μm in length, although some species are longer and some shorter. A single flagellum originates near the hind end of the organism from a dark-staining basal body (‘kinetosome’). It meanders initially along the length of the body and is held in place by the ‘undulating membrane’ which gives the trypanosomes their characteristic appearance (see Figure 4.10). The remainder of the flagellum lies free and protrudes anteriorly. Trypanosomes swim with a corkscrew movement. Most trypanosomes have indirect life-cycles using insect vectors which become infected while taking a blood meal from a mammalian host. After an initial period of multiplication in the insect gut, the life-cycle proceeds in one of two ways, depending on species: There are, however, two trypanosome species of veterinary importance that do not multiply within a vector. The first of these, T. evansi, relies on mechanical transfer of infected blood from host to host by tabanid flies, stable flies or vampire bats. If any of these are disturbed while feeding, they will seek a new animal to satisfy their appetite. Trypanosomes transported in this way will die if not quickly transferred to a new host. Another species, T. equiperdum, does not use a vector at all but is transmitted directly from host to host during coitus. It is therefore a venereal parasitosis. After entering a new host, trypanosomes multiply in the skin, causing a transitory inflammatory swelling before entering the blood-stream. In most Trypanosoma species, the trypomastigotes stay in the blood to replicate by binary fission. Those of T. cruzi, however, do not divide but instead enter various tissues of the body and proliferate as intracellular amastigotes before reentering the circulation as a new generation of trypomastigotes. The distribution of the various trypanosome-associated diseases is largely dependent on the presence and abundance of potential vectors. Different species of tsetse fly, for example, have different feeding preferences and are restricted to either riverine, savannah or forest habitats in sub-Saharan Africa. The reduviid bugs that carry T. cruzi are found in parts of Latin America living inside buildings. They emerge from their hiding places at night to take blood meals. Help box 4.1 Host factors can also influence the incidence and severity of disease. Some indigenous breeds of African cattle, for example, are less vulnerable to the effects of infection than imported European cattle. Trypanotolerant wild animals can remain parasitaemic for prolonged periods without showing signs of disease, thereby acting as reservoirs of infection. T. brucei infects antelope as well as cattle, horses and donkeys in Africa, while T. cruzi infects armadillos and possums as well as humans in South America. Susceptible animals that survive the acute phase of infection are able to mount an effective immune defence but this gives only temporary protection. African trypanosomes are able to evade host immune responses by varying the surface antigens on their glycoprotein coat (by a number of processes including trans-splicing RNA). This they can do repeatedly, thereby outmanoeuvring each successive immune attack. The result of this see-saw battle between host and parasite is a relapsing parasitaemia (see Figure 4.12). Figure 4.12 Diagrammatic representation of the relapsing parasitaemia associated with trypanosomosis. Each coloured curve depicts the number of trypanosomes expressing a particular surface antigen. Host antibodies generated in response to each antigen are represented by ‘Y’-shaped symbols in the corresponding colour. The pathogenicity of trypanosomosis varies with host species and breed, and with parasite species and strain. Infections are usually chronic with fever and malaise marking each parasitaemic episode. Lymph nodes and spleen are swollen due to plasma cell hyperplasia, which in turn leads to high nonspecific IgM blood levels and eventually to lymphoid exhaustion. Immunity to other pathogens is thereby compromised. Animals also develop a haemolytic anaemia and there is progressive weight-loss. Fertility and work capacity are reduced. T. cruzi infections are generally asymptomatic but considerable tissue damage can accrue in humans as a result of intracellular parasite replication in heart, oesophageal and intestinal muscles. T. congolense, T. brucei and T. vivax are all major pathogens of cattle in sub-Saharan Africa, although they affect other domesticated animals and wild reservoir hosts as well (see Table 4.2). T. congolense and T. vivax are responsible for both acute and longer-term disease while T. brucei tends to be more chronic. There are several subspecies of T. brucei, some of which are more important as human pathogens while others are primarily of veterinary significance. The clinical picture associated with T. brucei infection is complicated by the fact that it can invade extravascular tissues such as myocardium and CNS. Table 4.2 Some important Trypanosoma species T. evansi is spread mechanically in North Africa and Asia by biting flies and, in Latin America, also by vampire bats. The associated disease is called ‘surra’ and occurs mainly in cattle, buffalo, pigs, horses and camels, but T. evansi can infect many other animals as well. The venereally transmitted T. equiperdum replicates in subcutaneous and other tissues provoking transitory urticarial plaques and oedema of the genitalia and adjacent tissues. Progressive loss of condition and neurological complications may lead to a fatal outcome. Donkeys are often asymptomatic. This condition is known as ‘dourine’ and occurs widely but has been eradicated in North America, most European and some other countries. Leishmania species are closely related to the trypanosomes. Only one morphological form, the amastigote, occurs in mammalian hosts. This is found inside phagocytic cells such as macrophages, monocytes and polymorphonuclear neutrophils. The amastigote is oval with a rudimentary flagellum that does not protrude beyond the body (see Figure 4.11). Leishmania is responsible for serious disease in humans, dogs and wild animals (see Figure 4.13). About 30 morphologically indistinguishable species have been recorded, mainly in the Mediterranean region and parts of Asia, South America and Africa. The European species is L. infantum, but this comprises several distinct strains (‘zymodemes’). Each species of Leishmania uses a specific phlebotomine sandfly vector. Infected host cells are ingested when the fly takes a blood meal. The organism multiplies in the insect gut and migrates to the proboscis where it waits to be inoculated into a new host. Transmission can also take place if the fly is crushed onto abraded skin. Successive cycles of replication follow in the mammalian host as amastigotes multiply within and then destroy their host cells before seeking others to parasitize. Disease risk is largely determined by the distribution of the sand-fly vectors: Phlebotomus spp. in the Old World and Lutzomyia spp. in the Americas. In Europe, canine leishmaniosis is particularly prevalent along the Mediterranean coast, with some inland foci of transmission. Many infected dogs are asymptomatic. These provide an unsuspected source for onward transmission to other dogs or humans. There are also reservoirs of infection in some wild animals, especially rodents. Leishmaniosis is not endemic in northern Europe, although isolated cases do occur. This happens as local dogs have no opportunity to develop immunity and are therefore highly susceptible to infection if taken south on vacation. Such animals are a potential danger to their owners and in-contact pets at home as mechanical transmission can take place in the absence of sandflies if infected material is rubbed into a skin abrasion (see Section 9.3.2). A well-documented outbreak of leishmaniosis, caused by a particular strain of L. infantum, occurred recently in hunt kennels in New York State. Infected fox-hounds plus a few dogs of other breeds have since been found in other parts of the USA. Circumstantial evidence suggests that, with no sandflies in the region, direct transmission by close contact may have been responsible in this instance. Infection can also be passed from dog to dog by blood transfusion. Dogs are the main reservoir for human infection in the Mediterranean basin and parts of South America. Wild animals play a significant role in many rural environments, although human-to-human transmission via sandfly bites predominates in some regions. Leishmaniosis is a slowly progressing and relapsing disease. Young dogs are most vulnerable. The first clinical signs may not become evident until months or years after infection. The events taking place during this long incubation period are complex and lead to two main clinical presentations in dogs: cutaneous and visceral (see Section 9.2.3). The following processes contribute to these outcomes: Although the flagellates covered in this section do not invade host tissues, they are nevertheless very damaging in their different ways. Giardia is a common cause of diarrhoea, with an estimated two million human cases occurring annually in the USA, for example. This parasite is also found in wild and domesticated mammals. The trichomonads are a group of parasites responsible for diseases ranging from infertility in cattle and digestive upsets in cats to feeding problems in birds. Spironucleus is a common cause of mortality in game bird poults, while Histomonas is responsible for ‘blackhead’ in turkeys. All reproduce by binary fission and have simple, direct life-cycles. Giardia and Spironucleus encyst to enhance their chances of survival outside the host, but the trichomonads are transmitted as naked trophozoites. Histomonas is unique in that it uses a nematode worm to transport itself from host to host. Giardia may be found in the upper small intestine of a wide range of mammalian hosts (see Figure 4.14). Although it can provoke acute, intermittent or chronic diarrhoea of varying severity, many infections are asymptomatic. There are several species but that of greatest importance in veterinary and human medicine is G. duodenalis (also known as G. intestinalis or G. lamblia). Accumulating evidence suggests that G. duodenalis is not a single species but a series of morphologically identical forms with differing biological attributes that can only be differentiated at a molecular level. Figure 4.13 Cutaneous leishmaniosis: an advanced case showing ulceration on the nose and general alopecia. Reproduced with permission of L.H. Kramer. Figure 4.14 Giardia in small intestine: trophozoites (circled) can be seen between villi, some adhering to the epithelium. Reproduced with permission of AHVLA, Carmarthen © Crown copyright 2013. Giardia trophozoites are 15–20 μm long and pear-shaped with four pairs of flagellae (see Figure 4.4). They are bilaterally symmetrical with a separate nucleus on either side of the body (see Figure 4.15). They also have a large anterior disc used for attachment to the intestinal wall (see Figure 4.16). Figure 4.15 Giardia trophozoites growing in a culture medium. Figure 4.16 SEM of Giardia trophozoite showing attachment disc and flagellae. Reproduced with permission of R.C.A. Thompson. Because the epithelial cells lining intestinal villi are continually being shed (as part of a normal physiological process), Giardia trophozoites have to relocate at frequent intervals. Heavy infections may be associated with a flattening of the intestinal mucosa (villous and crypt atrophy), leading to decreased production of digestive enzymes, malabsorption of nutrients, enhanced gut motility and consequently weight loss. Trophozoites finding themselves in the lower small intestine form resistant cysts (see Figure 4.17). These are ovoid, measure 10 μm in diameter and have four nuclei. They are passed in the faeces of infected animals at irregular intervals, commencing a few days after infection. They are immediately infective and can be transmitted to a new host by faecal-oral transfer or via faecal contamination of drinking water. Figure 4.17 Giardia cyst. Redrawn after William Ober from Roberts and Janovy Jr., 1996 with permission of McGraw-Hill Education. After the cyst is swallowed, the released organism immediately splits into four individuals, thereby enhancing its chances of establishing in its new host. Serious disease can be initiated by ingestion of just a few cysts, which is why polluted water supplies can be a potent source of infection. Giardia infections may be abbreviated by host immune responses or they can persist for many months. Prevalence rates up to 15% have been recorded in European and North American dogs and cats, with the highest figures coming from urban areas and in younger animals. Such statistics, however, tell only part of the story, as the percentage of the host population carrying the various G. duodenalis genotypes (called ‘assemblages’) varies considerably from place to place. This is of veterinary public health significance as not all genotypes occurring in pet animals are potentially zoonotic (see Table 4.3). Table 4.3 Major Giardia duodenalis genotypes * These are proposed species names not yet in common usage at the time of writing. Two assemblages, A and B, have a very broad host range and these are the ones that pose a zoonotic hazard. There are well-documented cases of human epidemics being caused by the contamination of rivers or reservoirs with Giardia cysts originating from wildlife (e.g. beavers) or grazing livestock. There is also the possibility of direct faecal-oral transmission from pets to humans, or vice versa. Other assemblages are host specific and so household dogs infected with C or D, or cats with F, are unlikely to expose their owner to any risk. The part played by pets in the epidemiology of human giardiosis and the reverse role of humans in initiating canine/feline disease is still ill-defined and is the subject of on-going investigation. Many trichomonad species are commensals in the large intestine but some are pathogenic. Genus names within the group often reflect the number of forward-pointing flagellae. A feline example has five such flagellae and is therefore called Pentatrichomonas. An exception is Trichomonas gallinae which has four anterior flagellae. T. gallinae causes a serious and often fatal disease in pigeons and wild birds (e.g. finches) characterised by necrotic nodules in the oesophagus and crop. The most important trichomonad in veterinary practice is Tritrichomonas foetus, which causes reproductive problems in cattle and digestive disorders (typhlocolitis) in cats. T. foetus is a venereally transmitted trichomonad that lives in the uterus of cows and the preputial cavity of bulls. It occurs worldwide, although it has become rare or absent in countries where artificial insemination is widely employed. This pear-shaped protozoan is 10–25 μm long. It has three anterior flagellae and another projecting backwards which is bound by an undulating membrane for most of its length (see Figure 4.18). When seen in vaginal or preputial washings, the organisms swim with jerky movements. The body is supported by an obvious longitudinal rod (‘axostyle’). Figure 4.18 Tritrichomonas foetus. Redrawn from William Ober from Wenrich and Emmerson, 1933 in Roberts and Janovy Jr, 1996 with permission of McGraw-Hill Education. Chronically infected bulls show no clinical signs. In cows, infection may induce early abortion or may manifest as an irregular oestrous cycle or infertility. Uterine discharge, endometritis, pyometra and other reproductive dysfunctions may also occur. This flagellate was until recently called Hexamita. It resides in the lumen and crypts of the upper small intestine of turkeys and game birds. It causes infectious catarrhal enteritis in poults but older birds are often symptomless carriers. Spironucleus is small (less than 10 μm) and when seen in mucosa scrapings moves characteristically fast and straight. It is bilaterally symmetrical with a nucleus and three flagellae on each side of a narrow body. A further two flagellae trail behind (see Figure 4.19). Figure 4.19 Spironucleus. Redrawn from Perdue et al., 2008 with permission of the American Association for Laboratory Animal Science. Histomonas infects turkeys, game birds and free-range chickens. The associated disease, infectious entero-hepatitis, is known colloquially as ‘blackhead’ as the head and wattle sometimes become cyanotic (blue, due to deficient blood supply). It is seen mostly in young turkeys and there can be a high mortality rate. Histomonas is irregularly round or oval, measuring between 6 and 20 μm (see Figure 4.20). A single flagellum is present on histomonads in the caeca, but not on those in the liver. Figure 4.20 Histomonas meleagridis. Redrawn after Cheng, 1986 with permission of Elsevier. Infection can take place by simple faecal-oral transfer within a contaminated area. Wider transmission, however, is an intricate process which starts when an intestinal histomonad invades the tissues of another parasite co-inhabiting the caeca of the avian host. This is the nematode worm, Heterakis (see Section 7.1.3). The protozoan travels to the ovary of the worm and is incorporated into an egg which eventually passes into the environment with the bird’s droppings. The worm eggs are tough and long-living. If an egg is subsequently ingested by an earthworm, the nematode larva contained within hatches and remains inside its newly adopted transport host. Thus, the histomonad is now inside a nematode larva which, in turn, is inside an earthworm (see Figure 4.21). If and when the earthworm is caught and eaten by another bird, two parasitic transmission cycles (Heterakis and Histomonas) are completed. Figure 4.21 Transmission of Histomonas: the histomonad (yellow) is inside the nematode larva (blue), which is inside the earthworm (red), which has been swallowed by the bird. On arrival in a new host, histomonads invade the caecal wall and multiply, producing ulcers. They travel by way of the hepatic portal system to the liver where they continue to reproduce, forming necrotic lesions throughout the parenchyma. In histological section, the parasites do not stain readily and appear as pale, amorphous ‘ghosts’ (see Figure 4.22). It is not known if these histomonads return to the intestine or if their presence in the liver is accidental and a biological dead-end. Figure 4.22 Histomonads in the liver: the parasites appear as pale pink ‘ghosts’. The coccidia are a very large group containing many parasites of considerable importance to veterinary and human medicine. They may be divided into those genera that produce tissue cysts in intermediate hosts (discussed in Section 4.7) and those that do not. Some, such as Isospora, do not fall conveniently into either category but seem to be intermediary as they do not form tissue cysts seen by light microscopy, yet molecular evidence suggests genetic affinity with those that do. Coccidian life-cycles form a chain of increasing complexity ranging from the ‘simple’ pattern displayed by Eimeria, which undergoes sexual and asexual development in the same host, via Sarcocystis, which alternates between final and intermediate hosts, to Toxoplasma and Neospora, which can cycle indefinitely in intermediate host populations but retain the genetic advantages of sexual replication when opportunity arises (see Table 4.4). Table 4.4 Examples of coccidian life-cycles 1FH = final host; IH = intermediate host; 2 the life-cycle of Besnoitia is still poorly understood. The dominant genus amongst the ‘simple’ coccidia (i.e. those that do not form tissue cysts) is Eimeria. This is of greatest significance in the poultry industry but it also causes disease in ruminants and some other animals. Isospora is of relatively minor importance but can be detrimental to the health of piglets, dogs and cats. Another genus, Cryptosporidium, which until recently was considered an atypical ‘simple’ coccidian, is now known to be a very distant relative. As it differs fundamentally in some aspects of its biology, it will be considered separately (in Section 4.9). The genus Eimeria is widespread in nature with multiple species occurring in virtually every vertebrate host. With a few exceptions, all Eimeria species are host specific. Most inhabit the epithelial lining or deeper tissues of the alimentary tract, although a few are found in other locations such as bile ducts or renal tubules. Many are relatively harmless but others are serious pathogens. In broad outline, the Eimeria life-cycle involves three stages of replication (see Figure 4.23): Figure 4.23 Overview of Eimeria life-cycle (details in text). During sporogony, the fertilised cell divides into four pairs of daughter organisms (sporozoites). Each pair of sporozoites is held within an enclosing wall (the ‘sporocyst’). Thus, an Eimeria oocyst contains four sporocysts each containing two sporozoites (see Figure 4.6). The oocyst is now capable of infecting a new host and is said to be ‘sporulated’. More detail has to be added to the above overview of the Eimeria life-cycle to provide an adequate foundation for understanding the epidemiology, pathogenesis, diagnosis and control of coccidiosis (see Figure 4.24): Figure 4.24 Life-cycle of a typical Eimeria species: a – sporozoites from swallowed oocyst invade intestinal cell; b – schizogony; c – merozoites released; d – gametogony; e – oocyst formation and release; f – sporulation (details in text which uses same lettering as shown above). Based on Soulsby, 1982 with permission of Wolters Kluwer Health – Lippincott, Williams & Wilkins. Help box 4.2
Protozoa (single-celled parasites)
4.1 Introduction
4.2 Key concepts
4.2.1 Classification
4.2.2 Locomotion
Locomotion by:
Intracellular stages?
Ciliates
Cilia
No
Amoebae
Pseudopodia
No
Flagellates
Flagellae
Some spp.
Apicomplexa
Gliding
Yes
4.2.3 Nutrition
4.2.4 Transmission
4.2.5 Reproduction
4.3 Ciliates
4.4 Amoebae
4.5 Flagellates
4.5.1 Haemoflagellates
Trypanosoma
Life-cycle
Epidemiology
Pathogenesis
Important trypanosomes
Tsetse-transmitted species
Host
Zoonotic?
Vector
Transmission
Distribution
More information
T. congolense
Wide host range
▼
Tsetse fly
Salivarian
Sub-Saharan Africa
T. brucei
Wide host range, varies with subspecies
▼▼▼ (some subspecies cause sleeping sickness)
Tsetse fly
Salivarian
Sub-Saharan Africa
2.2.5 8.2.3
T. vivax
Wide host range
Tsetse fly
Salivarian
Africa, South America
T. evansi
Wide host range
▼
Biting flies, vampire bats
Mechanical
North Africa, Asia, Latin America
T. equiperdum
Horse
None
Venereal
Mediterranean area, South Africa, South America
9.1.4
T. cruzi
Armadillo, possum, human
▼▼▼ (Chagas disease)
Reduviid bug
Stercorarian
Latin America
2.2.4 9.3.2
Trypanosoma evansi
Trypanosoma equiperdum
Leishmania
Life-cycle
Epidemiology
Pathogenesis
4.5.2 Other flagellates
Giardia
Epidemiology
Assemblage
Species name*
Host specificity
Hosts
A, B
A – G. duodenalis B – G. enterica
Broad
Human, dogs, cats, livestock and wildlife
C, D
G. canis
Narrow
Dogs
E
G. bovis
Narrow
Livestock
F
G. cati
Narrow
Cats
G
G. simondi
Narrow
Rats
Trichomonads
Bovine trichomonosis
Spironucleus
Histomonas
4.6 Coccidia
4.6.1 General characteristics
Genus:
Tissue cyst forming?
Life-cycle1
Transmission
Eimeria
No
One host only
Horizontal
Isospora
No
Some species: FH →FH only Other species: FH→FH ↔ (IH optional)
Horizontal
Sarcocystis/ Besnoitia2
Yes
Alternating FH ↔ IH
Horizontal
Neospora/ Toxoplasma
Yes
IH ↔ IH (↔ FH optional)
Horizontal and vertical
4.6.2 Eimeria
Life-cycle
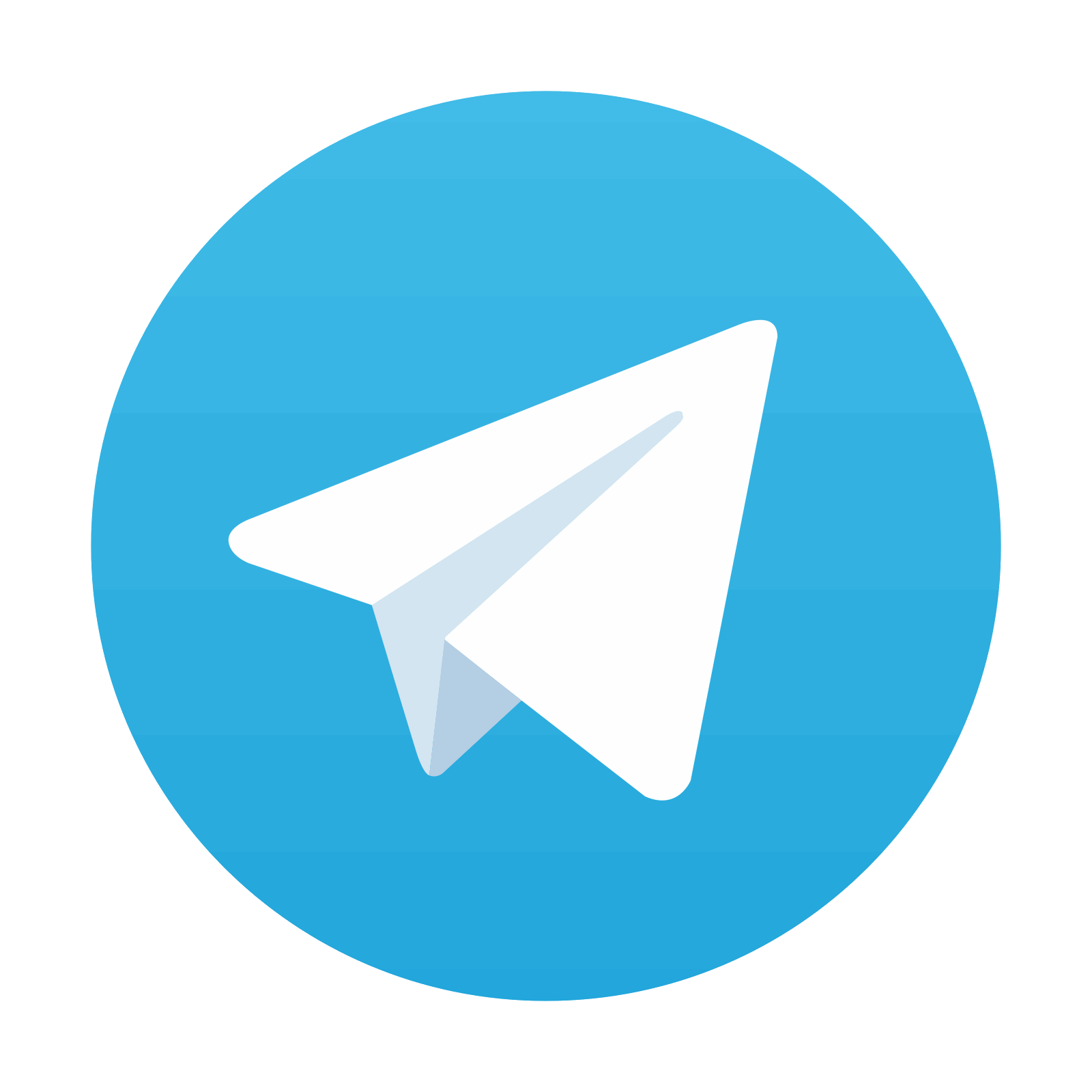
Stay updated, free articles. Join our Telegram channel

Full access? Get Clinical Tree
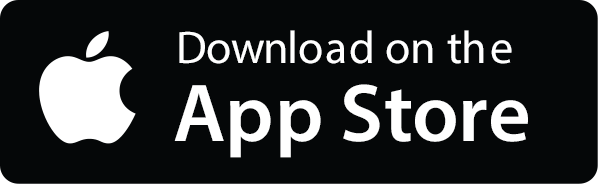
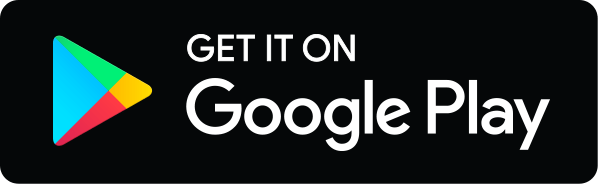