Seth Bleakley CARE Surgery Center, Phoenix, AZ, USA External coaptation is defined as the use of bandages, splints, casts, or other materials to provide support, compression, and sometimes analgesia. Indications for use include fractures and wounds. In fractures, the goal of external coaptation may be In cases of primary fixation, the goal of external coaptation is to prevent displacement of fractured bone so as to increase chances of secondary bone healing with close to anatomical alignment as well as to reduce discomfort from swelling and moving fracture fragments. In cases of ancillary support of a surgical repair, the goal of external coaptation is to reduce forces on the repair that could be detrimental to construct stability and implant integrity, as well as manage swelling and pain. In cases of temporary fixation, the goal is to improve alignment and reduce pain from moving fracture fragments and swelling until definitive treatment can be pursued. With regard to wound management, external coaptation is used to prevent contamination, aid hemostasis, reduce desiccation of tissues, absorb exudate, reduce swelling, aid thermoregulation, maintain wound contact layers, and promote moist wound healing. Wound management is discussed briefly in Chapter 18 and is described in detail in separate texts, the most recent one being: Textbook of Wound Management (edited by Dr. Nicole Buote). The purpose of this chapter is to describe mechanical principles of external coaptation for orthopedic indications. The Hippocratic phrase “first, do no harm” applies to decision‐making with external coaptation. One of the major disadvantages of external coaptation in small animal practice is morbidity, which can take the form of sores, ischemic injury,1 muscle atrophy, flexor tendon laxity/weakness,2 joint stiffness, decreased ligament strength,2 cartilage loss,3 and gait miseducation. Figure 40.1 shows examples of abrasions and ischemic injuries associated with external coaptation in dogs. In a study of nine dogs and two cats that suffered from ischemic injury secondary to bandaging, five animals required skin grafts, three required digit amputations, and two required limb amputations.1 Avoiding such morbidity with external coaptation requires appropriate case selection, padding, tension, protection from the elements, and patient and owner compliance. A secondary goal of this chapter is to help the veterinary practitioner avoid bandage morbidity through an understanding of the mechanical principles of external coaptation. When a transverse force is applied to a fixed longitudinal structure, the resultant reaction is known as bending moment and is directly proportional to the product of the force and the distance from the pivot. An unconstrained longitudinal structure, such as a limb, will require application of at least three forces, one opposing the other two, to create bending moment. A simplistic example of this is bending or breaking a pencil (Figure 40.2). Three forces are required, two in opposition to a central force. Less force is required if the distance between them is increased. It is challenging to bend and break a pencil if it is held with fingers close together, or in other words, more force is required as distance decreases. In the context of external coaptation, forces are applied by the immobilized limb against the soft or rigid materials applied to constrain the joint from flexion. Forces involved are a factor of the patient’s weight, the ground reactive force, and the position of the applied materials. Figure 40.3 demonstrates this in the canine hindlimb. Without muscle resistance, weight bearing will lead to extension of the paw, flexion of the tarsus, flexion of the stifle, and flexion of the hip (Figure 40.3a). Subsequently, the paw will displace cranially, the tarsus caudally, the stifle cranially, and the hip caudally. In the context of external coaptation, immobilization of a limb will involve application of corrective forces (CFs) in three locations. In the hindlimb, this would involve caudal forces on the paw and the tibia, and a cranial force on the tarsus (Figure 40.3b). Clinical relevance of this principle is clear when examining bandage sores (Figure 40.1), which, in the hindlimb, are typically associated with the digits, the caudal tarsus, and the cranial tibia. Careful padding of these locations is essential to prevent morbidity, but the length of the construct also becomes relevant due to the aforementioned formula for bending moment. A shorter bandage will decrease the distance between the CFs, and as ground reaction force is constant, the force to resist bending moment will be subsequently greater (Figure 40.3c). This, coupled with decreased surface area (which is inversely proportional to pressure), will lead to a higher incidence of sores and ischemic injury. This has been demonstrated experimentally. In a prospective study of 13 dogs, Iodence et al. demonstrated through pressure mapping with sensors underneath casts applied to the pelvic limbs that a short cast significantly increased pressure on the calcaneus and proximal tibia (Figures 40.4, 40.5, 40.6, 40.7, and 40.8). The principle of the three‐point corrective system will guide clinical application of external coaptation by creating awareness of the effect of construct length and location. Generally speaking, the longer the bandage, splint, or cast, the more effectively it will resist limb movement and lower the chance of bandage sores and ischemic injury. In practicality, external coaptation should include the paw and extend as proximally as possible – to the level of the stifle in the hindlimb and to the level of the elbow or above in the forelimb. Attention should be paid to the location of subsequent CFs. For example, padding should be adequate around the paw, caudal calcaneus, and proximal tibia in the hindlimb and around the paw, carpus, and proximal antebrachium/elbow in the forelimb, to correspond with CFs as demonstrated in Figures 40.3, 40.4, 40.5, 40.6, 40.7, and 40.8. Rigid materials should be trimmed or adapted in these locations to mitigate pressure and abrasions, especially over bony prominences. Thoughtful application will help ensure morbidity of external coaptation does not become a bigger problem than the presenting complaint. Area moment of inertia is a cross‐sectional property used to predict the resistance of an object to bending and deflection. A basic understanding of area moment of inertia aids clinical decision‐making with external coaptation by helping ensure adequate strength and rigidity while balancing padding and weight (Figure 40.9). This formula becomes relevant in the context of ensuring mechanical adequacy of a cast. A small increase in cast thickness through application of additional material will lead to a large increase in resistance to bending (specifically, the increase in cast thickness to the power of 4). There is also relevance in application of cast padding. Adequate cast padding is desired to prevent sores, but excessive padding can increase motion within the cast (reducing immobilizing function), as well as affect the rigidity of the cast. With a fixed volume of cast material, a wider cast diameter will decrease the thickness and, therefore, resistance to bending of the cast. Thus, additional rolls of cast material may be needed in casts with wider diameters to ensure mechanical adequacy. Figure 40.1 Examples of abrasions and ischemic injuries associated with and secondary to external coaptation in dogs. Source: © Seth Bleakley. Figure 40.2 The principle of bending moment can be demonstrated simplistically by bending/breaking a pencil. Three forces are required and are represented by the finger icons. Increasing the distance between the forces as in part (b) makes it easier to bend/break the pencil when compared to the more narrowly distributed forces demonstrated in part (a). This formula (AMI of a cuboid) has relevance to splinting. If a splint is applied caudally or cranially, resistance to bending will be proportional to the thinnest section of the splint to the power of 3. This would be akin to bending a ruler (Figure 40.10) when it is flat; intuitively not very rigid, as h becomes the thickness of the ruler while b remains the length. If a splint is applied laterally or medially, h becomes the width of the splint, and area moment of inertia is increased to width of the splint to the power of 3. Similarly, bending a ruler turned on its side is intuitively challenging; explained by the width of the ruler becoming h in the formula while b remains the length. Therefore, splints will be much stronger and more resistant to bending when applied laterally or medially. In the context of external coaptation, pressure is directly proportional to bandage tension and inversely proportional to the radius of the limb and the bandage width.1 Figure 40.3 Schematic of the canine hindlimb demonstrating the principle of the three‐point corrective system. (a) demonstrates the direction of joint movement during flexion of the unconstrained limb in response to ground reaction force; (b) demonstrates application of three corrective forces (CFs) to resist flexion, such as in a long cast; (c) demonstrates how a short cast would alter the proximal CF, decreasing the distance. As ground reaction force is constant, the force to resist bending moment will be subsequently greater. This, coupled with decreased surface area (which is inversely proportional to pressure), will lead to a higher incidence of sores and ischemic injury. Figure 40.4 Schematic diagram that depicts the concept of the three‐point corrective system for a tall cast (a) and short cast (b) applied to a pelvic limb of a dog. The force acting at the calcaneus is the corrective reaction force (CRF) for the tall (CRFa) and short (CRFb) casts. For the tall cast, the two opposing CFs are acting at the points where the cast terminates on the cranial surface of the proximal portion of the tibia (CF2a) and digits (CF1a). For the short cast, the two opposing CFs are acting at the points where the cast terminates on the cranial surface of the distal portion of the tibia (CF2b) and digits (CF1b). For both cast configurations, the forces of both CFs are expected to equal the force applied at the CRF location. The extended lever arm associated with the tall cast configuration results in lower pressures at CF2a and CRFa, compared with the pressures at the corresponding locations (CF2b and CRFb) with the short cast configuration. Source: Iodence et al.4 published with permission from Dr. Felix Duerr. Figure 40.5 Photographs of a pressure sensor, application of the pressure sensors to a pelvic limb of a dog, and outputs from the sensor. (a) Photographs of the pressure sensor (top) and a magnified view of the six sensor columns (bottom). (b) Photograph of three sensor columns positioned (starting proximally at the tibial tuberosity) over the cranial aspect of the pelvic limb of a dog. (c) Photograph of one sensor column positioned over the calcaneus. Notice that the two other sensor columns in the photograph are positioned outside the area to be evaluated (i.e., outside the calcaneus). (d) Photograph of the screen overlay for the pressure‐mapping software. Notice that the investigator is applying direct pressure to the area to be evaluated to record its location on the screen overlay for subsequent use in data analysis. (e) Photograph of the final positioning of the sensors, cast padding, and fiberglass cast on a pelvic limb. The sensor cuff is affixed to the exterior of the cast over the tarsus. The sensor wires attach directly to the pressure‐mapping system. (f) Photographs of the screen overlays of three recorded pressure measurements. Notice that areas of lower pressure appear dark blue, whereas areas of higher pressure appear yellow or green. Source: Iodence et al.4 with permission from American Veterinary Medical Association. Figure 40.6 Photographs of procedures for the application of a short cast configuration. (a) Photograph of the longitudinal transection of a short cast on the lateral and medial aspects of the limb by use of a battery‐operated oscillating saw. (b) Photograph of a short cast on the pelvic limb. Notice that the two halves of the cast have been aligned, fully compressed, and taped together circumferentially. (c) Photograph of the final positioning of a short cast with the sensors, cast padding, and short cast in place. The sensors and padding were not removed to ensure there was no change in sensor placement. The sensor cuff is affixed to the exterior of the cast over the tarsus. The sensor wires attach directly to the pressure‐mapping system. Source: Iodence et al.4 with permission from American Veterinary Medical Association. Figure 40.7 Mean and 95% confidence intervals for the mean pressure (MP) and peak pressure (PP) (a) and mean force (MF) and peak force (PF) (b) of a tall cast configuration (dark gray bars) and a short cast configuration (light gray bars) at the level of the calcaneus for 13 dogs. *Within a variable, the value for the short cast configuration differs significantly (P ≤ 0.05) from the value for the tall cast configuration. Source: Iodence et al.4 published with permission from Dr. Felix Duerr. Figure 40.8 Mean and 95% confidence intervals for the MP and PP (a) and MF and PF (b) of a tall cast configuration (dark gray bars) and a short cast configuration (light gray bars) at the level of the proximal edge of each cast configuration for 13 dogs. See Figure 40.7 for remainder of key. Source: Iodence et al.4 published with permission from Dr. Felix Duerr.
40
Principles of External Coaptation
Introduction
Mechanical Principles
Three‐Point Corrective System
Area Moment of Inertia and Rigid Materials
Pressure
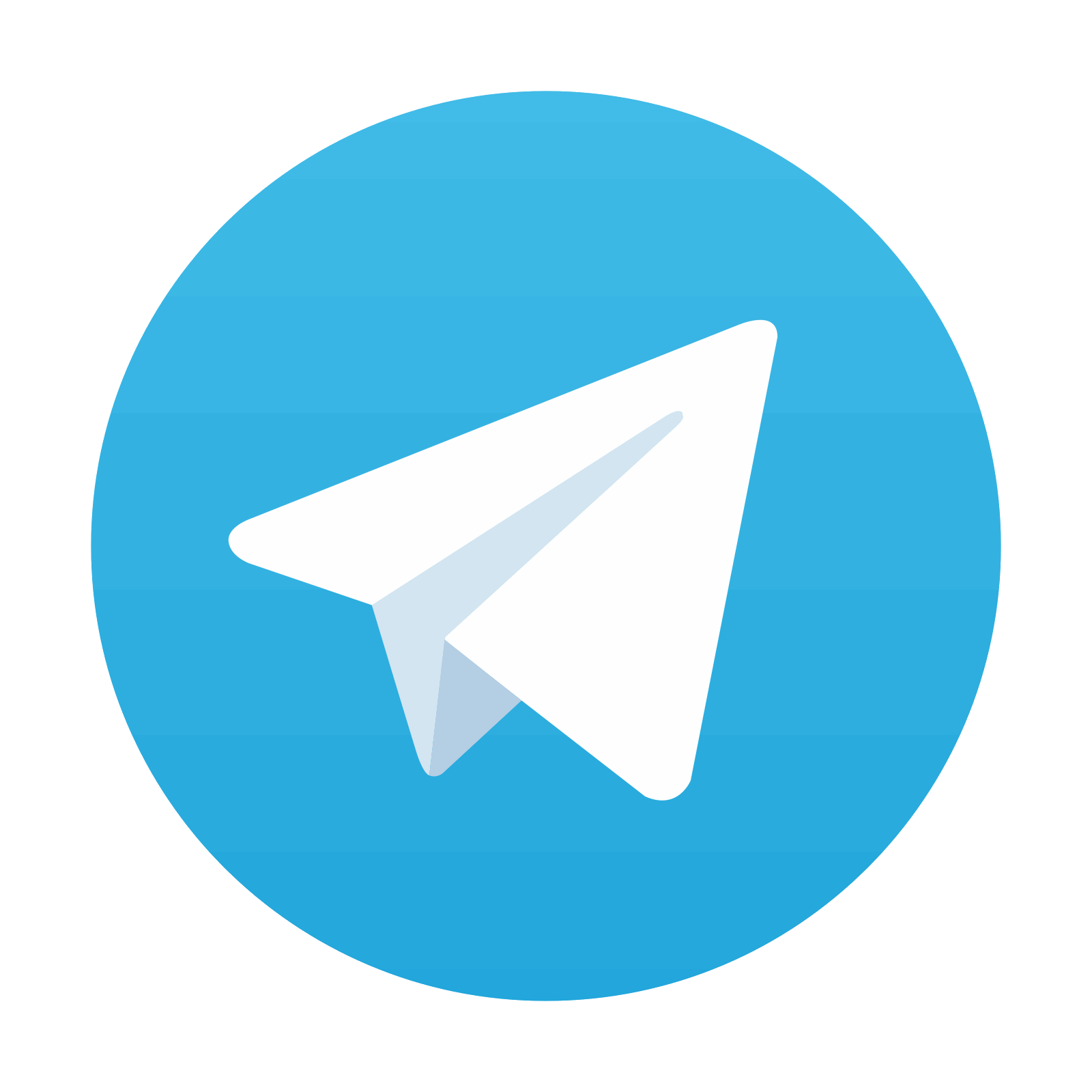
Stay updated, free articles. Join our Telegram channel

Full access? Get Clinical Tree
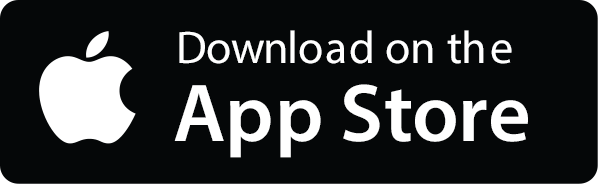
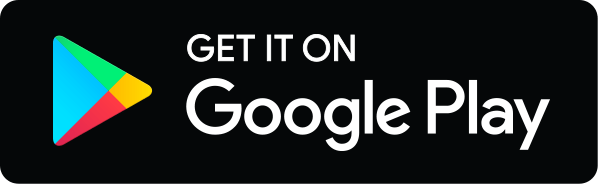