Table 6-4 Integration of Population Pharmacodynamic (PD) and Pharmacokinetic (PK) Data and Its Role in the Design of Dosing Regimens
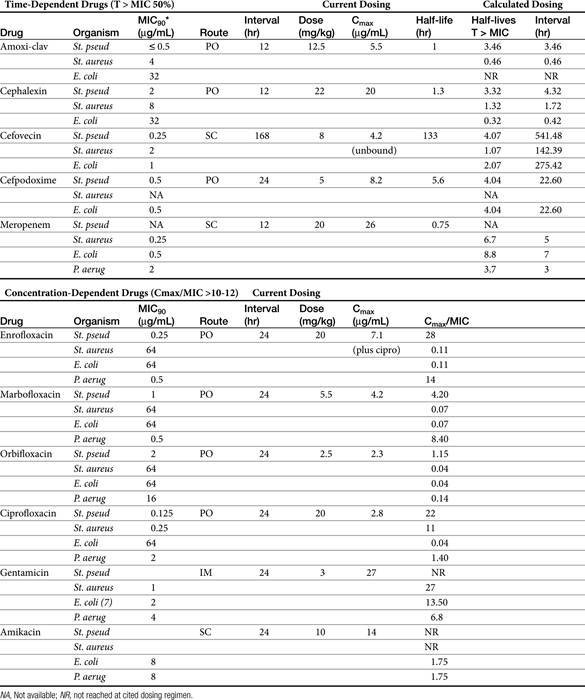
6 Principles of Antimicrobial Therapy∗
Judicious Antimicrobial Use
“Even experienced practitioners may not realize that giving a patient antibiotics affects not just that patient, but also their environment, and all the other people that come into contact with that environment.” Dancer’s1 statement, intended as a warning to practitioners of human medicine, emphasizes the importance of judicious antimicrobial therapy. It is understood that the goal of antimicrobial therapy is successful treatment of infection. However, the less judicious the approach taken to achieve that goal, the more likely a path to future failure is paved. The goal of antimicrobial therapy must be further modified to include avoidance of resistance, a goal that is not necessarily accomplished with successful resolution of infection. Although it might be tempting to consider that human and veterinary medicine are differentially affected by antimicrobial resistance, in reality both are inexorably linked, and what affects one will affect the other. As early as 1998, the National Foundation for Infectious Diseases estimated the cost of antibiotic-resistant bacteria to be as high as $4.5 billion annually and that they are responsible for more than 19,000 (human) deaths per year.2 The impact is evident globally, nationally, in the community setting, in the hospital environment, and within the hospital, particularly with regard to at-risk patients (e.g., critical care).3 Any antimicrobial used to treat a patient ultimately must be excreted into the environment; the impact of this is just now being addressed scientifically.
Empirical antimicrobial selection may become an approach of the past. As medical communities struggle to assess impact, causes, and means of avoidance, inappropriate antimicrobial use clearly is a consistent contributing factor to antimicrobial resistance. Inappropriate use includes both excessive and unnecessary use, as well as inappropriate dosing regimens. In the United States alone, approximately 350 million pounds of antibiotics are consumed annually in human medicine.2 The numbers in veterinary medicine are less clear, but 20 million pounds were consumed by food animals during the same period.2,4 Approximately 40% of human consumption of antimicrobials is considered unnecessary.2 That we do not have a similar statistic regarding therapeutic antimicrobial use in veterinary patients reflects, perhaps, a somewhat cavalier attitude regarding antimicrobial stewardship both on the part of the manufacturers and the users. The veterinary profession has been intensely scrutinized by the medical community regarding its use of antimicrobial products in animals. This sometimes scientific and frequently emotional focus began with use in food animals but is shifting to companion animals.5 Guardabassie6 has described the role of the family pet as a reservoir of potentially resistant zoonotic organisms. Resistant strains of Staphyloccocus intermedius, Campylobacter, Salmonella, and Escherichia coli were cited as possible zoonotic concerns. At least 1% of annual salmonellosis cases in humans are assumed to be associated with companion animals.6 Approximately 6% of Campylobacter jejuni infections in humans (children) are transmitted from pets.6,7 Methicillin-resistant Staphyloccoccus aureus (MRSA) has been isolated in family members and pets in the same household8–10; methicillin-resistant Staphylococcus intermedius∗ (MRSIG) has been reported in human patients11; and, perhaps disconcertingly, MRSA of animal origin (not previously identified in humans) has been identified in animals, albeit food animals (pigs).12 In dogs E. coli strains are phylogenetically similar to pathogenic strains causing infection in humans; more than 15% of canine fecal deposits in the environment contain E. coli strains related to virulent human strains.6 The concern regarding E. coli relates, in part, to its ability to develop resistance in the presence of antibiotic concentrations considered therapeutic.13,14 Extraintestinal pathogenic E. coli (ExPEC, the “other” bad E. coli) appears to easily colonize the gastrointestinal tract, potentially displacing commensals and eventually emerging as infectious organisms in other body tissues, particularly in the urinary tract.15 Further, E. coli is able to share mechanisms of resistance with other enteric pathogenic coliforms such as Salmonella.13 Evidence exists for the transfer of resistance between E. coli and Salmonella and subsequent transfer of these organisms between animals (pet and farm animals) and humans16
The pernicious advent of resistance over decades of antimicrobial use reveals that, despite their safety to the patient, antimicrobials are not innocuous drugs.17 As in human medicine, antibiotic stewardship (i.e., judicious antimicrobial use) should become the focus for reducing resistance in veterinary medicine.18 Prudent veterinarians and veterinary practices will implement decision-making processes (antimicrobial use paradigms) that minimize the temptation to use antimicrobials as alternative therapies. Designing a dosing regimen on the basis of cost and convenience, rather than on pharmacodynamics and pharmacokinetics, must become a paradigm of the past. Antimicrobial stewardship begins by recognizing the problems and issues and successfully implementing procedures that reasonably minimize the impact of antimicrobial use in the patient while not forfeiting the likelihood of therapeutic success. It is with this appproach this chapter emphasizes the rational basis for decision making in the selection of the proper antimicrobials.
Definitions and Goals
The terms antibiotic, antibacterial, and antimicrobial are often used interchangeably, despite their different meanings. Antibiotics are natural chemicals (e.g., penicillin) produced by organisms intended to suppress other organisms (generally, but not exclusively, bacteria), whereas antimicrobial refers to any compound, whether natural, synthetic, or a combination thereof, that suppresses microbial growth. Antibacterials target bacteria, antifungals target fungi, and so forth. The term microbes usually refers to bacterial organisms but also includes fungal and other (nonviral) organisms. Bacteria can be further categorized on the basis of their Gram-staining characteristics and their morphology (Box 6-1). This classification is helpful when matching bug to drug. In addition to staining characteristics, bacteria can be defined as aerobic—that is, those that generate energy (ATP) by aerobic respiration of oxygen. Some aerobes have minimal capacity to generate energy in the absence of oxygen and can be referred to as obligate aerobes. Although Pseudomonas aeruginosa might be classified as an obligate aerobe, it has the capacity to function, as with many other aerobes, as a facultative anaerobe. Facultative anaerobes prefer an oxygen-rich environment but are quite capable of switching to fermentation in the absence of oxygen. An example environment in which P. aeruginosa is able to survive in reduced oxygen environments (in humans) is cystic fibrosis. Other examples of facultative anaerobes include the families Enterobacteriaceae (e.g., E. coli, Klebsiella pneumoniae, Proteus mirabilis), Vibrionaceae (e.g., Vibrio, Aeromonas), and Pasteurellaceae (Pasteurella, Haemophilus). In contrast, obligate anaerobes cannot tolerate the presence of oxygen more than a few seconds. Examples include members of the family Bacteroides. Clostridia sp. is an example of an anaerobic organism whose oxygen toleration ranges from moderately tolerant (e.g., Clostridia tetani) to highly tolerant (e.g. Clostridia perfringens). Microaerophilic bacteria lie somewhere between aerobic and obligate anaerobes, in that they require low concentrations of oxygen to survive. Examples include Helicobacter and Borrelia spp.19 When collecting a culture sample for such organisms, extreme care must be taken to prevent its exposure to oxygen. Aerotolerant organisms are not affected by either the presence or the absence of oxygen.
Box 6-1 Bergey’s Classification of Medically Relevant Bacteria
(From Krieg NR, Staley JT, Hedlund B et al: Bergey’s manual of systematic bacteriology, ed 2, Volume 4: The Bacteroidetes, Spirochaetes, Tenericutes (Mollicutes), Acidobacteria, Fibrobacteres, Fusobacteria, Dictyoglomi, Gemmatimonadetes, Lentisphaerae, Verrucomicrobia, Chlamydiae, and Planctomycetes, New York, 2010, Springer.)
The goal of antimicrobial therapy is to achieve sufficient concentrations of an appropriate drug at the site of infection such that the infecting organism is killed, while simultaneously avoiding side effects of the drug in the patient. In today’s age of emerging resistance, the goal must be modified to include the avoidance of antimicrobial resistance. Therapeutic decisions concerning antimicrobial therapy for the infected patient are among the most challenging (Figure 6-1). Unlike most other drug therapies, antimicrobial therapy must take into account microbe, drug, and patient factors (i.e., the chemotherapeutic triangle), many of which confound successful therapy to the point of causing failure (Figure 6-2). Antimicrobial therapy is most likely to be successful when the target (and thus spectrum of antimicrobial activity) is known such that pharmacodynamics (PD) of the infecting organism can be integrated with the pharmacokinetics (PK) of the drug in the patient.
Identifying the Need for Antimicrobial Therapy
The first decision to be made regarding antimicrobial therapy is determining the need to treat (see Figure 6-1). The decision includes confirming, to the extent possible, the existence of infection; identification of the cause of the infection bacteria (or fungal, etc), the need for treatment of the infection; and, if treatment is deemed necessary, whether antimicrobials should be part of the therapy. This first decision is probably given the least consideration yet may be the most important if resistance is to be avoided. It also may be the most difficult to make. The presence of infections frequently cannot be confirmed for a variety of reasons, such as the lack of (infection-) specific clinical signs, location in an inaccessible site, and costs associated with accurate diagnosis. Infection is supported, but not necessarily confirmed, by clinical signs or laboratory tests indicating fever, inflammation, and organ dysfunction or structural changes detected by imaging techniques such as radiology, ultrasound, and magnetic resonance imaging. Culture may support, but does not necessarily confirm, infection. Newer detection methods based on molecular diagnostic techniques (e.g., polymerase chain reaction) may ultimately prove to be important tools in the rapid bedside diagnosis of infectious diseases, including multidrug-resistant bacteria.20 However, simply documenting the presence of these microbes may not be a sufficient indication of cause and effect. These methods may not discriminate infection (reproducing, pathogenic organisms) and colonization (the presence, growth, and multiplication of the organism without observable clinical symptoms or immune reaction), or pathogens from normal microflora. An exception can be made if cytology reveals organisms phagocytized by white blood cells, but the absence of phagocytosis does not eliminate infection.
Internal structures and organs (e.g., bone, heart, kidneys, the lower respiratory tract) are normally sterile. Sterility may be maintained, in part, by secretions, which constantly clean or clear the site. In addition to bulk flow, secretions may contribute to sterility by the presence of endogenous antimicrobial compounds (e.g., tears, saliva, respiratory tract secretions, gastrointestinal acidity). However, in contrast, external (skin and conjunctiva of the eye) and internal (linings of the respiratory, digestive, and urogenital systems) surfaces are characterized by normal microflora. Normal flora may be further defined according to their contribution to host health or well-being. Most normal flora are commensals that appear to neither harm nor help the host. Some commensals, however, are also opportunistic in that they may become pathogenic, particularly if host health is impaired. A pathogen is a microbe that is associated with and capable of causing host damage.21 Pathogens often reflect the normal flora of infected sites, with E. coli, P. aeruginosa, K. pneumoniae, and S. aureus being common examples of opportunistic normal flora that can become pathogenic (Table 6-1). Mutualistic organisms help maintain microbial balance through host–microbe interactions. They provide beneficial effects such as producing acids that lower pH and blocking colonization by more dangerous microbes. Antibiotics secreted by mutualistic organisms help maintain the composition of aerobic and anaerobic commensal bacteria, resulting in a population that is most appropriate for host health and preventing colonization by pathogenic organisms. Opportunistic organisms may not originate from normal microflora but rather may be acquired from the environment (e.g., Aspergillosis, fungal organisms). Nosocomial organisms cause infections as a result of medical treatment, usually in a hospital or clinic setting. As such, a nosocomial infection is defined as one that arises 48 hours after hospital admission. Disruption of the environment, such as might occur with the use of antimicrobials that alter the anaerobic population, will also disrupt the balance of normal microflora, increasing the risk of infection (see the section on antimicrobial resistance). Not only will death of normal flora leave a void that can be filled in with more hardy and potentially pathogenic microbes, but the constant exposure of the microbes to antibiotics leads to ongoing development of mechanisms such that the microbes resist environmental drugs. Therefore the organisms are primed for resistance. Narrowing the spectrum of the chosen antimicrobial will help limit, although probably not prevent, the development of resistance.
Table 6-1 Normal Flora and Clinically Significant Infections by Organ System (Dogs and Cats)
Organ or Site | Organism | Comment |
---|---|---|
Blood | ||
Staphylococcus intermedius (D: 25%-35%)∗, †, ‡ | ||
Streptococcus spp. (D: 18%-21%)† | ||
Enterobacter cloacae (D: 3%-8%, C: 7%)† | ||
Escherichia coli (D: 35%-45%∗; D: 18%-71% & C: 14%)† | ||
Klebsiella pneumoniae (D: 25%-35%∗: C: 14%)† | ||
Proteus (D: 14%)† | ||
Pseudomonas aeruginosa (D: 10%-20%) | ||
Salmonella (D: 11%-13%; C: 29%)† | ||
Obligate anaerobes (D: 10%-20%) | ||
Endocarditis† | Staphylococcus intermedius (D: 6%-33%) | |
Streptococcus spp. (D: 12%-26%) | ||
Escherichia coli (D: 6%-30%) | ||
Erysipelothrix rhusiopathiae (D: 19%) | ||
Corynebacterium spp. (D: 19%) | ||
Respiratory | ||
Upper | Staphylococcus intermedius (D: 30%-35%)§, ║, ¶ | Have been isolated from nasal swabs, tonsillar and pharyngeal swabs, or tracheal and lung swabs |
Streptococcus spp. (15%-27%)§, ║, ¶ | ||
Corynebacterium spp, §, ║, ¶ | ||
Escherichia coli§ (15%-29%)§, ║ | ||
Klebsiella pneumoniae (D: 10%-15%)║, ¶ | ||
Moraxella§, ¶ | ||
Neisseria§, ║ | ||
Pasteurella multocida (D: 15%-34%; C: >50%)§, ║ | ||
Proteus (C: <10%)§, ║ | ||
Pseudomonas§ (6%-34%)§, ║ | ||
Bacteroides║ | ||
Clostridium spp. § | ||
Fusobacterium║ | ||
Rhinitis, sinusitis | Escherichia coli | |
Pasteurella multocida | ||
Proteus | ||
Pseudomonas spp. | ||
Tracheobronchitis∗ | Bordetella | |
Lower | ||
Staphylococcus intermedius (D: 10%-15%) | Normal bronchi and lungs sterile distal to first bronchial division | |
Escherichia coli (D: 30%-40%; C: 15%-20%) | ||
Bordetella (D: 10%-15%) | ||
Enterococcus | ||
Klebsiella pneumoniae (D: 15%-20%; C: <10%) | ||
Pasteurella multocida (C: >50%) | ||
Pseudomonas | ||
Proteus mirabilis (D: <10%) | ||
Pleuritis | Actinomyces, Bacteroides, Corynebacterium, Fusobacterium, Nocardia, Pasteurella, Staphylococcus, Streptococcus | |
Gastrointestinal | ||
Oral cavity | Beta-hemolytic Streptococcus | Isolates from healthy dogs§ |
Staphylococcus epidermidis§ | ||
Acinetobacter§ | ||
Escherichia coli§ | ||
Moraxella§ | ||
Neisseria§ | ||
Pasteurella§ | ||
Proteus§ | ||
Pseudomonas§ | ||
Obligate anaerobes (80%-90%) | ||
Small intestine | Escherichia coli, Klebsiella,¶ | Enteropathogenic bacteria in the stomach or small intestine associated with enterotoxin¶ or mucosal invasion |
Enterobacteriaceae§ | Campylobacter fetus¶ | |
Moraxella | ||
Neisseria | ||
Proteus spp. | ||
Pseudomonas spp. | ||
Salmonella typhimurium§, ¶ | ||
Shigella§ | ||
Vibrio cholerae¶ | ||
Vibrio parahaemolyticus§ | ||
Yersinia enterocolitica¶ | ||
Clostridium perfringens (type A) ¶ | ||
Bacillus§, ¶ | ||
Large intestine | Enterobacteriaceae∗ | ∗Normal microflora; anaerobic make up 90% of microflora |
Enterobacteriaceae§ | Anaerobes | |
Peritonitis | ||
Hepatobiliary | ||
Enterobacteriaceae | ||
Escherichia coli | ||
Enterobacter | ||
Klebsiella | ||
Genital | ||
Staphylococcus intermedius (D: 15%-25%)§ | Normal microflora of distal urethra and prepuce§ | |
Acinetobacter§ | ||
Escherichia coli (30%-35%)§ | ||
Klebsiella§ | ||
Moraxella,§ Haemophilus§ | ||
Pasteurella multocida (10%-25%)§ | ||
Proteus sp.§ | ||
Pseudomonas aeruginosa (<10%) | ||
Obligate anaerobes (C: 10%-25%) | ||
Mycoplasma spp.§ | ||
Ureaplasma spp.§ | ||
Staphylococcus intermedius (D: 15%-25%)§ | Normal microflora of canine vagina§ | |
Staphylococcus epidermidis§ | ||
Streptococcus canis, S. faecalis, S. viridans, S. zooepidemicus§ | ||
Corynebacterium§ | ||
Acinetobacter§ | ||
Citrobacter§ | ||
Enterobacter§ | ||
Enterococcus§ | ||
Escherichia coli (30%-35%)§ | ||
Haemophilus§ | ||
Klebsiella§ | ||
Micrococcus§ | ||
Moraxella,§ Neisseria§ | ||
Pasteurella multocida (15%-25%) | ||
Proteus§ | ||
Pseudomonas aeruginosa (<10%)§ | ||
Obligate anaerobes (C: 10%-25%) | ||
Mycoplasma§ | ||
Ureaplasma§ | ||
Urinary Tract | ||
Staphylococcus intermedius (D: <10%) | ||
Enterococcus faecalis (D: <10%) | ||
Escherichia coli (40%-50%) | ||
Klebsiella pneumoniae (10%-15%) | ||
Pasteurella multocida (C: 10%-15%) | ||
Proteus mirabilis (10%-15%) | ||
Pseudomonas aeruginosa (C: <10%) | ||
Central Nervous System | ||
Brucella | ||
Pasteurella | ||
Ocular | ||
Conjunctiva | Staphylococcus intermedius,§, ¶ S. albus¶ | Cultured from the conjunctival sac of clinically normal dogs or cats§¶ |
Beta-hemolytic Streptococcus (C: 15%-25%)§, ¶ | ||
Corynebacterium§, ¶ | ||
Escherichia coli¶ | ||
Moraxella§ | ||
Neisseria§ | ||
Pasteurella multocida (C: 10%-20%) | ||
Pseudomonas§ | ||
Proteus | ||
Bacillus§, ¶ | ||
Chlamydia psittaci (C: 50%-75%) | ||
Mycoplasma¶ | ||
Eye | Leptospira | |
Brucella canis | ||
Clostridium tetani | ||
Mycobacterium bovis | ||
Otitis externa | Staphylococcus intermedius (D: 25%-30%) | |
Escherichia coli (D: 10%-20%) | ||
Proteus mirabilis (D: 20%-25%) | ||
Pseudomonas aeruginosa (D: 15%-25%) | ||
Skin | Staphylococcus intermedius (D: 60%-70%) | |
Escherichia coli (20%-30%) | ||
Pasteurella multocida (C: >50%) | ||
Proteus mirabilis (<10%) | ||
Pseudomonas aeruginosa (D: <10%) | ||
Wounds, abscesses | Staphylococcus intermedius (D: 25%-50%) | |
Escherichia coli (D: 20%-30%; C: 10%-20%) | ||
Pasteurella multocida (C: 30%-40%) | ||
Proteus mirabilis (D: 10%-20%; C: <10%) | ||
Pseudomonas aeruginosa (D: 10%-20%) | ||
Obligate anaerobes (25%-35%) | ||
Musculoskeletal | ||
Osteomyelitis | Staphylococcus intermedius (D: 40%-50%) | |
Staphylococcus aureus | ||
Escherichia coli (D: 10%-20%) | ||
Enterococcus faecalis (D: 10%-20%) | ||
Proteus mirabilis (10%-20%) |
∗∗Organisms that are cultured from clinically healthy animals may be difficult to distinguish from those that cause infection.
∗ Numbers in parentheses refer to probable percentages of infections in this tissue that are caused by the organism, as cited by Aucoin (1993). Unless noted otherwise, the percentages refer to both dogs and cats (D = dog; C = cat). Note that the probable percentage is likely to vary geographically and may be biased toward patients referred to a specialty service.
† Numbers in parentheses refer to probable percentages of infection in this tissue that are caused by the organism, as cited by Greene (1990).
‡ Number in parenthesis reflects the range of percent cited by both Aucoin (1993) and Greene (1990).
§ ¶ For each tissue, the symbol is defined in the Comment column.
Identifying the Target Organism
Empirical Antimicrobial Therapy
After it has been determined that infection does exist and warrants medical management with antimicrobial drugs, identification of the target is the second critical decision to be made. Antimicrobial selection is probably most often made empirically—that is, on the basis of assumptions regarding the infecting organism and its susceptibility to drugs. These assumptions are based on historic data that identify organisms most commonly associated with infections of various body systems (see Table 6-1).22 However, older data may not have discriminated between commensals and pathogens — indeed even today such discrimination often is not possible — which complicates the accuracy of prediction. More problematic, as resistance has emerged, the risk of incorrectly identifying the susceptibility pattern of an infecting microbe has increased. Thus the clinician should carefully balance the risk of therapeutic failure, including recurrence of infection with a resistant microbe, with the cost associated with more accurate diagnostic procedures.
The utility of Gram staining in the selection of an antimicrobial should not be overlooked as a means to narrow the spectrum of the chosen antimicrobial. Gram stain characteristics differ on account of differences in the layers penetrated by the Gram (purple) stain. The cell wall is many layers thicker in gram-positive organisms than in gram-negative ones thus rendering them more susceptible to some drugs that target the cell wall; further, the gram-positive isolates do not have an external lipopolysaccharide (LPS) covering that is present in gram-negative organisms (Figure 6-3). Whereas the LPS layer is the source of endotoxin responsible for the morbidity and mortality associated with many gram-negative infections, just as this external covering precludes stain movement into the cell wall, it also serves as a barrier to drug movement into the organism (see Figure 6-3).23 Movement, particularly of water-soluble drugs, is generally restricted to outer membrane proteins that span the breadth of the covering (porins); however, changes in porin size and efflux pumps are mechanisms by which gram-negative organisms overcome drug movement through porins.
In addition to Gram staining, determining the source of infection may help identify the microbe because some organisms are more likely than others to infect certain body systems. For example, genitourinary tracts are often infected with gram-negative aerobes, whereas abdominal infections generally are caused by gram-negative aerobes initially, followed by anaerobes after several days (see Table 6-1).24,25 Skin is most commonly infected with Staphylococcus pseudintermedius (to be referred to as S. intermedius group, or SIG), abscesses with anaerobes and Pasteurella spp., and the urinary tract with E. coli. Indeed, E. coli is one of the more common pathogens, infecting many tissues. One study of 674 E. coli isolates collected from dogs found the vast majority (n=424) associated with urinary tract infections (UTIs) (n=424); however, 61 were also collected from skin, respiratory tract (52), ear (43), female (42) and male (25) reproductive tracts, and other organ systems (23). However, although E. coli may indeed be the most common isolate associated with UTIs, it does not necessarily represent the majority of UTIs. In a study by the author, only 50% of UTIs were caused by E. coli, with the remaining 50% caused by Staphylococcus spp., Enterococcus spp., Proteus, and others. For critical patients, organisms generally represent the normal flora of the alimentary canal or a nosocomial organism.26 Granulocytopenic or otherwise immunoincompetent patients also are more likely to be infected by aerobic gram-negative organisms.
Even if the organism is correctly identified, the greater risk of failure associated with empirical treatment lies in the inability to correctly predict susceptibility patterns. This is not a new concern: As early as 1996, a study of critical-care patients revealed that empirical selection of antimicrobials was incorrect, on the basis of cultures collected before antimicrobials were started, in nearly 45% of patients.27 Further, isolates of four organisms collected between 1998 and 2000 (P. aeuriginosa, P. mirabilis, E. coli, Staphylococcus spp.) widely considered to be susceptible to enrofloxacin (which had been approved for approximately 10 years) were characterized by a higher than expected incidence of resistance (28% for E. coli).28 More recently, a high level of resistance was ascribed to drugs used empirically to treat otitis interna29 and pyothorax.30 Finally, our laboratory has demonstrated that more than 40% to 60% of E. coli associated with UTIs in dogs are characterized by resistance to first- and second-choice drugs (amoxicillin/clavulanic acid, cephalexin, potentiated sulfonamides, and enrofloxacin).31 These differences may be regional but the absence of a robust surveillance program for dogs and cats limits empirical antimicrobial selection. These studies suggest culture and susceptibility (C&S) testing will become increasingly important.
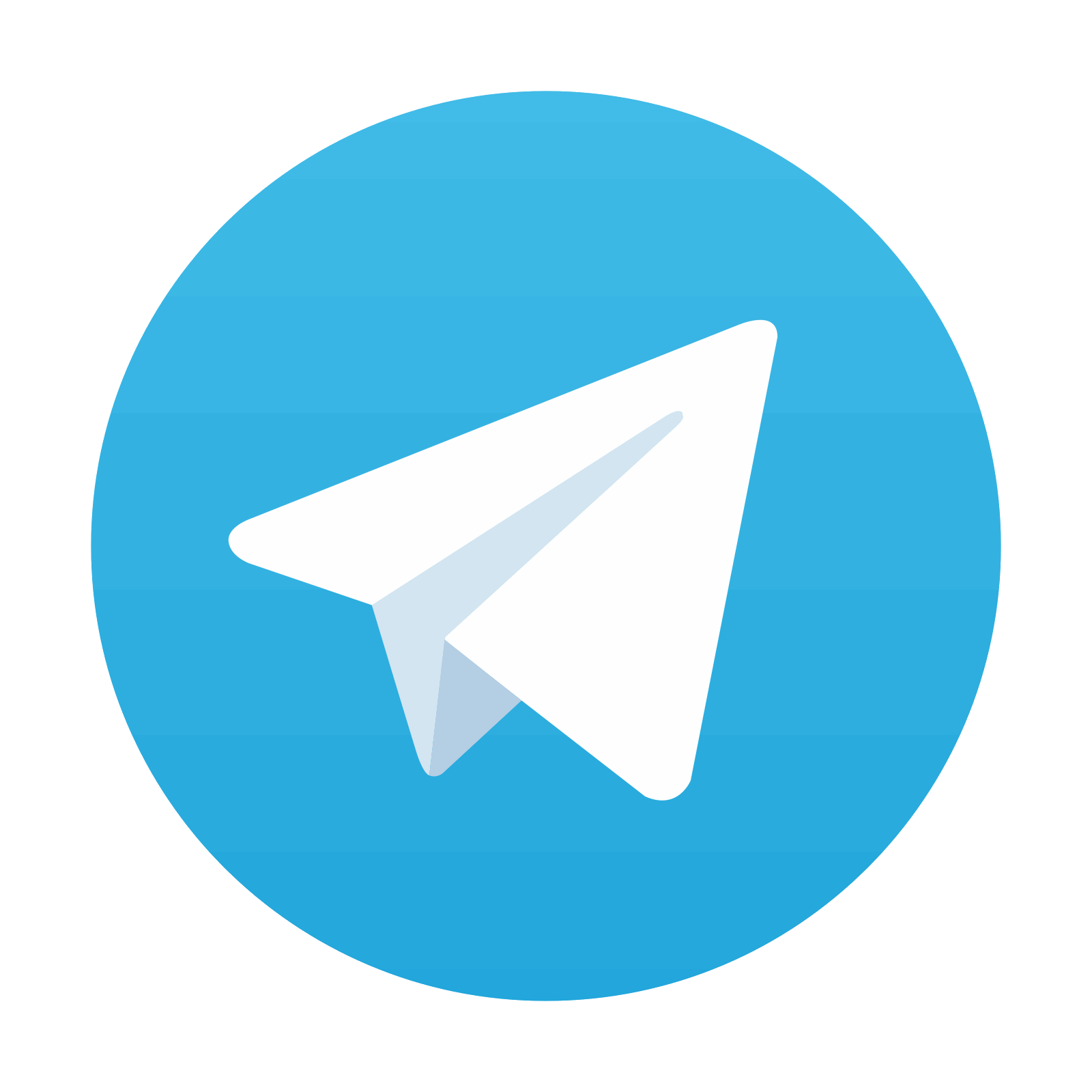
Stay updated, free articles. Join our Telegram channel

Full access? Get Clinical Tree
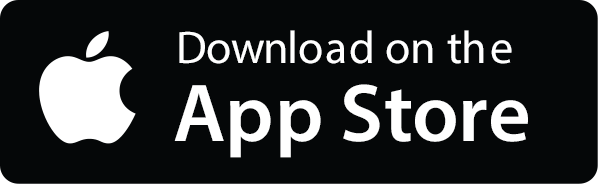
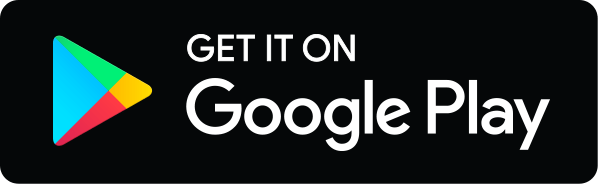