There are many methods which can be used to minimise the effect of variation, through different experimental designs (blocking, factorial design) or statistical analyses such as analysis of variance (ANOVA) or covariance analysis. See Festing et al.1 for full details and explanations. Factorial designs can be used to find the relationship between such variables as treatment, sex and strain; they also use fewer animals than other designs.
It is important to avoid bias due to one group of animals having a more favourable environment than another; as far as possible the animals and samples should be coded so that staff are ‘blind’ to the treatment groups. Otherwise it is possible that the observer may inadvertently score the treatment group differently from the control group when there is no difference.
Selection of animals: species, strain, genetically altered animals
Appropriate choice of animals is the first critical step to a successful scientific outcome. The first decision is which species of animal to use. Applying the principles of the three Rs and considering replacement, a number of procedures can be done effectively in vitro on cells in culture, or on invertebrates such as the insect Drosophila. When reviewing the choice of species, the principal factor influencing that decision will be the species that has the lowest capacity to suffer as a result of the procedures to be carried out, and the consequential housing and husbandry, which is consistent with obtaining satisfactory scientific results. Background historical data, financial cost and expertise may also have a bearing on the choices made. Although there has been an increase in the use of zebrafish for procedures, about 82% of scientific procedures were carried out on rodents in 2010 (www.understandinganimalresearch.org.uk/the-animals/types-of-animals/).
After deciding on the species, it is then necessary to decide on which stock or strain to use. More is known about the genome of the mouse than any other species, which is one reason for its popularity as a research animal, and there are many types of genetically modified mice available that are useful models for specific disease entities.
Rodents can be supplied as an outbred stock, which are colonies of genetically undefined animals. This means that the genotype of an animal at any given locus is usually unknown. Such animals usually have good fertility, with a good growth curve and a large litter size, and so are easy to produce. They are therefore cheaper and more readily available than inbred strains. (Outbred animals are known as stocks, whereas inbred ones are known as strains.) CD1 and MF1 are commonly used outbred stocks of mouse, and the Wistar and Sprague–Dawley varieties are common outbred rat stocks. A mutant is an outbred stock carrying a mutant gene such as nude or obese. The maintenance of mutant stocks depends on whether the mutant is dominant or recessive and whether homozygous mutant animals of one or both sexes are viable and fertile. Breeding systems are more complex to produce mutants because of harmful recessive genes, which may increase perinatal death and thus reduce productivity; therefore mutants are considerably more expensive to buy. Additionally special housing requirements may be necessary to protect susceptible mutants from infection.
Most frequently, research is carried out using an inbred strain, which is produced by 20 or more consecutive generations of brother×sister mating, producing animals that are 99% homozygous. Examples of commonly used inbred strains of mice are BALB/C, C57BL/6J and DBA/2. Fewer inbred strains exist for rats than for mice, but common ones include DA, F344, Lewis and PVG. Production of inbred strains requires much more by way of planned colony management than for outbred stocks, due to inbreeding depression in inbred strains, leading to a reduction in reproductive performance, survival and general fitness characteristics. These include lower weight, lower litter size, a higher pre-weaning mortality and a tendency to be more aggressive. The effects of inbreeding depression are most noticeable in the first few generations of brother×sister mating. An F1 hybrid is produced by mating two inbred strains.
As knowledge of genetics increases, more and more medical conditions are seen to result from the genotype. In order to understand the workings of genes and identify the causes of disease, increasing use is being made in biomedical research of genetically altered (GA) and harmful mutant (HM) animals. The genotype of an animal describes its genetic makeup, which is determined by the genes inherited from its parents. The phenotype of an animal describes its overall physical characteristics, which depend on the genetic makeup of the animal, and the interaction of the genotype with the environment. A GA animal is one in which the genotype has been altered deliberately by the insertion or deletion of DNA, in a way that does not occur naturally through mating or recombination of genes. Methods used for genetic modification include transgenesis (insertion of additional genes from the same or another species), or targeting of specific changes in genes or chromosomes, either by deletion or inactivation of genes (knock-outs), or addition of genes (knock-ins). An HM animal is one in which the genome has been altered by a point mutation or chromosomal change which do not involve insertion or deletion of DNA. Harmful mutants may arise spontaneously (e.g. the nude mouse, which is athymic and cannot mount a specific immune response), or be induced deliberately by mutagenesis, where viruses, chemicals or radiation are used to cause random changes in the genes. The offspring of animals exposed to such mutagens show random genetic changes and chromosomal rearrangements with resulting phenotypic changes. The alkylating agent ethylnitrosurea (ENU) is a powerful mutagen used in male animals including mice and zebrafish, and large number of F1 founder animals may be produced from a single treated male. These F1 mice can either be analysed directly for dominant mutations, or bred further to study recessive phenotypes. With dominant mutations, a clinical phenotype may only be observed in the homozygous state, but changes of specific parameters, for example a reduction in the activity of enzymes, can often be detected in heterozygotes. Recessive mutations can only be diagnosed from clinical appearance in homozygotes.
In any population, there is a considerable amount of genetic variation. This is caused by spontaneous mutations in the genome, since natural mutations occur at a low rate of about 1 in 10 000 to 100 000 individuals. The variations in genotype result in variations in the phenotype of animals, and this sometimes confers advantages on some animals over others in particular environments. This is the basis of natural selection, in which particular characteristics give an animal an advantage over its peers, so that it is more likely to survive and reproduce. This can be used as selective inbreeding: animals that bear the desired characteristics are chosen to breed, and those that do not are discarded, and thus are created the genetically stable lines of inbred rats and mice commonly used in research. However, many characteristics are poorly heritable, being controlled by many genes, so selective inbreeding is a very slow and inefficient way to increase the frequency of a particular gene in a population. Also, there can be undesirable side effects as selecting for some genes often results in the perpetuation of other, deleterious or recessive genes, leading to genetic diseases. Many inbred strains of mice and rats carry recessive genes, and are susceptible to particular diseases or exhibit poor growth or fertility2.
Transgenesis involves the microinjection of DNA containing the genes of interest into a fertilised egg, and implanting the resulting embryo into a recipient female. The effect of the transgene may depend on the background strain of the animal, effects being seen in one strain but not others depending on the other genes present3.
Embryonic stem cell manipulation was developed to overcome some of the problems encountered with microinjection into fertilised eggs. Manipulation of ES cells can also be used to stop the function of particular gene, producing a knock-out animal, or to add in a piece of DNA to change the way a particular protein is produced or regulated, producing a knock-in animal. It is also possible to delete or add in large regions of a chromosome with many genes. There are also other types of genetic manipulation (e.g. use of morpholinos or retroviral vectors) to inactivate genes temporarily and to manipulate genes in tissue specific areas.
Breeding and maintenance for both GA and HM animals is a regulated procedure, since this is deliberately done, albeit indirectly, to produce an individual embryo whose genetic makeup may result in harm, and the potential for harm persists beyond two-thirds of the way through gestation.
The nomenclature system of names and terminology is extremely important. Definition of the genetic background of the animals important to ensure the reproducibility of data and should be included in the methodology for the experiment.
Genetic and Welfare Monitoring of GA and HM Animals
GA and HM animals need to be monitored for the purity of the genetic line, in addition to the health status of the animals; and the welfare consequences of the genetic modification should also be monitored. Following genetic modification of fertilised eggs or embryos, the offspring produced are tested to make sure that the desired genes have integrated or been affected as required. It may be possible to determine this from the phenotype: sometimes the modified genes produce obvious effects such as coat colour changes, otherwise tissue samples may need to be analysed for the genes of interest. Methods such as saliva sampling or blood sampling may be possible if the gene can be amplified from tiny amounts of DNA using techniques such as polymerase chain reaction (PCR) prior to analysis. If not and the amount of DNA needed is greater, it may be necessary to take a biopsy from the ear. The smallest amount of tissue required should be taken.
There are considerable concerns about the effects of the genetic modification on the welfare of animals. The integration of a transgene within an endogenous gene may affect gene function, and the technologies employed in generating transgenic and cloned animals may result in an increased incidence of fetal and neonatal losses. New gene constructs may have unexpected adverse effects on the animal, producing obvious or subtle changes in the phenotype. It is expected that GA and HM animals will be monitored carefully for adverse effects, using scoring systems4. Detailed assessment of every new transgenic animal may be impractical: it can have considerable implications in terms of staff time and resources, and interference with neonatal animals may cause the mother to reject her young. It may be preferable to use a simple set of parameters such as litter size, birth weight and activity to identify those animals which are more likely to be affected. These may then be subjected to more detailed scrutiny, either as neonates or after weaning5. The welfare of affected animals may then be improved by revising husbandry methods such as floor feeding if locomotion is affected, or barrier rearing if they are immune-compromised.
Many normal animals are used in the generation of GA or HM animals, and if the techniques used are inefficient many of these animals are wasted. Many offspring produced in the initial stages may not be transgenic, and for lines maintained in a heterozygous state there will be a proportion of animals in every litter which are not carrying the gene of interest. In order to fully implement the three Rs, efforts should be made to utilise these normal animals as much as possible and to maximise the efficiency of the techniques used to generate new lines.
Background health status
Disease prevention is a prerequisite of any animal experiment to ensure scientific quality, animal welfare and safety6. Overt or subclinical infection is likely to affect experimental results so reducing reliability and reproducibility of the research. Infectious agents have the ability to cause ill health, thus compromising the welfare of the animals. Even subclinical infection can introduce greater variation between individuals, thereby increasing the numbers of animals required to produce statistically significant results. Some animal pathogens are transmissible to humans and it is essential to safeguard health of laboratory and research staff. It is therefore essential when selecting animals that due regard is paid to monitoring their health status.
While some infectious agents are pathogenic and will induce clinical signs of disease in laboratory animals with morbidity and/or mortality, many microorganisms induce only mild disease or no illness at all, particularly in cases of endemic disease. However, these cases are still important since the experimental procedures which cause stress and immunosuppression, or environmental factors, such as transportation or changes in diet, may convert subclinical infection into clinical problems. If it is recognised that an animal is unwell but that the changes are unrelated to the procedure it will be necessary to seek veterinary advice to investigate the causes of the disease and obtain advice on treatment. Different strains of mice may show various levels of sensitivity to an infectious agent, with some strains having asymptomatic infection while others may show differing degrees of clinical signs. In general the symptoms will be more serious in immunodeficient animals. The infectious agents do not only impact on the whole animal. Many will also affect experiments conducted on isolated organs or cells. They can persist in cells, tumour lines and other biological materials for prolonged periods of time and thus affect in vitro experiments or can be reintroduced back into whole animals with the passage of such contaminated material in animals. Thus the detection and elimination of such agents affects each one of the three Rs of Replacement, Reduction and Refinement: eradication of infection will improve the quality of in vitro work, reduce the number of animals that have to be used and improve the welfare if animals used are in good health. The breeding of GA and HM animals is no different. Health monitoring should be done as for any strain of animals, indeed increased use of animals with immune deficits may be contributing to the continued existence of various rodent pathogens such as parvovirus, rotavirus, Theiler’s murine encephalomyelitis virus (TMEV) and pinworms7.
Since most infections are subclinical and modifications of research results due to natural infections often occur in the absence of clinical disease, the lack of a clinical indication of the infection has only limited diagnostic value. It is prevention of infection, not just clinical disease, which is important. The presence of unwanted microorganisms and the suitability of a group of animals for a specific experiment can only be demonstrated by a comprehensive health-monitoring programme. The data generated by this programme constitute part of the experimental data and should be available for consideration in the interpretation of the test results. Thus the health monitoring information should be included in the publication of experimental results. There should be microbiological standardisation of laboratory animals to produce better and more reliable results of experiments with fewer animals. For details of the effects of rodent infections on experimental data see GV-SOLAS (the German laboratory animal science association)8, and for fish see Kent et al.9.
Sources of infection
The most important source of infection is other laboratory animals10, often due to population density and the turnover of animals within a unit. Animals entering a unit should be of known health status and come from accredited breeders. Accompanying such animals should be a report on their microbiological status and housing. There are various terms used to describe the microbiological status of laboratory animals, which in turn indicates the type of housing in which they are maintained.
- Germ-free (axenic) animals are free of any associated life and are maintained in isolators with no contact with the outside world.
- Gnotobiotic animals have one or more associated non-pathogenic microorganisms, the identity of which is known. These animals are also maintained in isolators with no contact with the outside world.
- Specific-pathogen-free (SPF) animals are free from specified pathogens. The SPF designation requires that the absence of the listed pathogens is supported by regular monitoring for those pathogens. These animals are maintained in barrier units which use a combination of construction, equipment (e.g. individually ventilated cages), operating procedures (including showering facilities, the provision of sterilised food, water and bedding) and restricted access to the unit to minimise any potential contact with those specified pathogens. Sometimes these are referred to as barrier-reared animals.
- Conventional animals carry normal microbial flora which is undefined. Their housing has less strict access and operating conditions. However, they are should still be monitored for the main animal pathogens.
- Wild-caught animals have an unknown microbial flora and are likely to carry pathogens that may cause disease to both staff and other animals.
Other animals brought into the unit, particularly in large facilities with multiple users, are a common cause of health status breakdown. A well-run facility will have a standard operating procedure (SOP) that should be followed for all incoming animals in order to protect the health of those already in the unit. The supplier’s health profile should be examined and should provide details of what tests were carried out, who did them and how the interpretation was arrived at. Environmental sources of contamination include water, food and bedding; all of which may therefore require sterilisation before being given to the animals. Effectiveness of water sterilisation should be checked regularly and food stored appropriately to prevent contamination from wild rodents and birds or depletion of vitamin levels or the development of moulds or toxins. Quality of the bedding must also be assured. Ventilation rates in rooms must be adequate and air filters changed regularly or there will predisposition to respiratory disease. All equipment must be clean so as not to spread infection to the animals. Examples of potential sources of contamination include such items as anaesthetic face masks and gavage cannulae. Cell lines and serum may be contaminated and should be screened before bringing them into units for use in animals. During transportation animals should be protected from infection where possible by transporting them in filter-protected cages11. Good hygiene procedures should be followed at all times in the animal unit.
Rodents, and many other species, are generally supplied with a health profile from the breeder. If the animals are to be kept only short term it may not be necessary to do any more, but if they are kept long term then continual regular screening will be necessary to ensure they have not picked up extra infections. If the animal is kept under conditions to prevent entry of disease it is important to maintain these barriers and not to bring microorganisms in to the unit.
Some animals may have predisposing factors such as a genetic variant which contribute to the development of disease. Genetic monitoring is vital to ensure that this predisposition remains a constant factor within the experimental model. The susceptibility of GA strains may be difficult to predict due to changes in the immune system. Nutritional status of the animal will also be a factor in susceptibility to disease. The single most important predisposing factor in the laboratory animal is stress, which leads to immunosuppression and the subsequent development of disease. It is therefore very important to do everything possible to reduce the stress caused to animals.
Health monitoring
Health monitoring of laboratory animals is a fundamental part of disease prevention used to determine their pathogen status and general health. Always note the date of health monitoring reports of colonies of animals, as they will only reflect the health status of a population at the time of testing.
The frequency of testing, which can vary between monthly and annually, depends on circumstances. The threat of disease – either clinical or subclinical – should be perceived as serious by all those involved in order for screening to be carried out effectively and for barriers to be maintained. The choice of agents to test for is based on pathogen significance and likelihood of interference with research and includes zoonotic diseases, subclinical or clinical diseases which may cause welfare consequences, and diseases which may interfere with or invalidate the experiment. The researcher should be aware of any infections which are likely to affect the research, and ensure that there is an appropriate screening programme in place to detect these infections before any problems are caused. Recommendations for health monitoring of mouse colonies may be found in publications by the Federation of Laboratory Animal Science Associations (FELASA)6 and Reuter and Dysko12.
Health monitoring in experimental units is a justifiable expense and an essential means of enhancing the reliability of the experiments. Health screening of the animals should not be a theoretical exercise and action should be taken if positive results are found. The epidemiology of the disease must be understood in order to know how frequently to test, what samples to take and how to deal with a positive result if it should be found. Advice should be sought from veterinary staff. The recommendations of FELASA6 for health monitoring in each species should be followed. The purpose of health monitoring is to supply the researcher with data on variables that might influence the outcome of an experiment. These data therefore are part of the experimental work and must be considered during the interpretation of the experimental results. The results of health monitoring should therefore be included in scientific publications. The decision about which infectious agents are acceptable and which are not is never straightforward. Many that are included on the standard list are historical and there is a need for rationalisation of the selection of agents to be screened for and those which management systems should be designed to keep out of rodent units. Since most infections are subclinical, the importance of an effective surveillance and barrier system is not always appreciated until it has been breached when short cuts are taken.
It has been argued that the move towards clean animals has reduced the ‘natural’ challenges to the immune system and can alter results from research previously carried out under conventional conditions and may even be detrimental to animal welfare13. However, the microbial variables are a critical influence on the research model and cannot be ignored. Exclusion is the most practical solution, which requires surveillance, diagnosis, control and management. It is important to interpret results in context, which requires an understanding of the biology of each agent, and the use of diagnostic pathology to support serological results rather than relying on serology alone.
In active health monitoring, animals are taken from the population at regular intervals. Blood is taken for serological testing for a number of viral infections and some bacterial infections and they undergo full post-mortem examination to detect any other infections. Sentinel animals can also be used to monitor the population. These are animals of the same species whose bacterial, parasitic and virological burden is already defined. They are housed in the same rooms as the experimental population, and some of the bedding from the cages of the experimental animals is placed into their cages at each clean out session. They will thus be exposed to the same pathogens and they can be sampled to determine their pathogen status which will reflect the status of the original experimental population. Immunodeficient animals (e.g. nude mice) do not produce sufficient amounts of antibodies to give reliable test results and are therefore not suitable for serological testing, although they may be used for bacteriological and parasitological investigations. Sentinel animals should be at least 10 weeks old and should have been housed in the unit and exposed to samples of used bedding for at least 4 weeks.
Sampling strategies
The determination of the number of animals to be screened for infection is very important and depends on the assumed rate of infection and the randomness in the sampling. There is a natural inclination to keep the sample size small on grounds of economy and availability, but it must be sufficiently large to give the required level of confidence in the results. For health screening for multiple agents which may have very different infection rates, it is impossible to ensure with 100% certainty that all agents will be detected with the same degree of precision. Routine sampling is usually based on assumed infection rates of around 40–50% in order to keep samples sizes reasonable but the limitations of this need to be recognised.
It is advisable to use two age groups of sentinels since young adults will be best for detecting recent viral infections and retired breeders give an indication of the history of the colony. The FELASA6 guidelines give advice on the frequency of testing, which can vary between monthly and annually, depending on circumstances.
Which diseases to monitor
It is not practical or cost-effective to test for all known infectious agents so testing, by necessity, has to be selective. The choice of agents to test for is based on pathogen significance and likelihood of interference with research and includes the following.
- Any zoonotic diseases should be the first priority in screening. This will enable any necessary precautions to be taken to prevent spread of disease to humans.
- The second group of diseases to look for will be those that might be present as subclinical infections but which will cause overt clinical disease when the animal is subjected to an experimental stress.
- The third group to consider are those diseases whose presence, whether clinically evident or only subclinical, will interfere with, and may invalidate the experiment. For example, this may be the presence of a viral infection that has no effect on the animal but elevates certain enzyme levels, the measurement of which may form part of the experimental study.
A discussion with the veterinary staff will aid in designing a sensible screening programme which will be cost-effective. Before embarking on a screening programme it is important to be prepared for the possibility of positive results, and to consider what action will need to be taken if the result shows the presence of certain infections. Action should be taken if positive results are found; otherwise it is a waste of time, money and animals not just in terms of health screening, but possibly for the whole experiment. The programme will need to be re-evaluated once the status of the colony is known, as subsequent tests may need to be done less frequently, or only certain groups of the population tested, after the initial screen. Screening can be a very costly and laborious task but this should not be used as an excuse to ignore the possibility that infections exist which may be of concern to personnel, detrimental to the animals’ welfare and to the validity of the science being carried out. Monitoring laboratories should follow the principles of Good Laboratory Practice (GLP) and the FELASA guidance for the accreditation of laboratory animal diagnostic laboratories.
Biosecurity and containment for rodents
Rather than conventional shoebox cages on open shelving or racks, housing for laboratory rodents is now frequently designed in such a way as to offer microbiological containment. This may be done because it is required as a strategy for the containment of animals which may pose a risk to human safety, because they are carrying biological agents that fall into hazard groups categorised by the Advisory Committee on Dangerous Pathogens. Sometimes the principal requirement is to protect staff from animal allergens, or it may be to prevent the spread of organisms between animals in order to maintain their high health status, perhaps to allow animals of different microbiological backgrounds to be housed in close proximity.
Individually ventilated caging systems
Individually ventilated cages (IVCs) are cages with filter tops held in a specialised rack that allows each cage to receive its air supply individually. Supplied air is high-efficiency particulate air (HEPA) filtered, and each cage is isolated from the others. This avoids spread of pathogens between cages, and prevents exposure of personnel to potential allergens or hazardous substances. The containment provided by the cage system must be maintained while the cage is cleaned or the animals examined. These procedures are therefore carried out in ventilated workstations or safety cabinets. There are three classes: class I protects the operator, class II protects the animal and the operator and has an easy access open front and class III is in effect a totally sealed box.
IVCs are complex cage systems that rely on engineering to support the animals’ environment. If this equipment is to fulfil its objectives thorough staff training is required, and standard operating procedures are required for IVCs and safety cabinets to ensure that the required level of containment is maintained. For more information see Chapter 5.
The IVC provides the animal with an environment that is controlled for ventilation, temperature and relative humidity. The containment provided by an IVC may be beneficial: the quality of the science may be improved by using healthier animals, enabling fewer animals to be used more effectively. However, since this type of caging makes the animal more remote from the staff and the animal technician cannot always see the animal easily, cannot hear or smell the animal, these valuable sources of information when assessing its overall condition are lost. The welfare of the animals will therefore depend even more on the qualities and skills of the staff employed.
Flexible film isolators
An isolator is like a large plastic bubble, which is sealed from the outside world. Filtered air is supplied, and equipment and food are passed in through a port which allows disinfection of the packaging prior to entry into the isolator. Isolators operate under negative or positive pressure, offering complete environmental protection against the spread of pathogens in or out of the isolator, so animals of different health status can be housed within the same room, in different isolators. They are mobile but do not make good use of available floor space. They must be alarmed in case of failure of the air supply and they can be difficult to work with.
Filter-top cages
Cages are held on an open rack, but covered with individual filters. Some containment of microorganisms is achieved with well-fitting lids, but the microenvironment in the cage is compromised as there is restricted airflow. The filter top should only be removed in a safety cabinet, which can make them difficult to work with.
Ventilated cabinets
These are similar to sealed cupboards with their own filtered air supply. The cabinet operates under negative pressure. Inside, filter-top cages are generally used. They are easy to install, relatively cheap and mobile but can present a risk when the cabinet doors are open or at cage changes.
References
1. Festing M, Overend P, Cortina-Borja M, Gaines-Das R and Berdoy ME (2002). The Design of Animal Experiments. Laboratory Animals Handbooks no. 14. London: RSM Press
2. Fox JG, Barthold SW, Davisson MT, Newcomer CE, Quimby FW and Smith L (eds) (2007). The Mouse in Biomedical Research, Vol. 1 and 2. New York: Academic Press
3. BVAAWF/FRAME/RSPCA/UFAW (2003). Refinement and reduction in production of genetically modified mice. Laboratory Animals 37 (suppl. 1): S1–51
4. Van der Meer M, Rolls A, Baumans V, Olivier B and van Zutphen LF (2001). Use of score sheets for welfare assessment of transgenic mice. Laboratory Animals 35: 379–89
5. Littin K, Acevedo A, Browne W, Edgar J, Mendl M, Owen D, Sherwin C, Wurbel H and Nicol C. (2008). Towards humane end points: behavioural changes precede clinical signs of disease in a Huntington’s disease model. Proceedings of the Royal Society B Biological Sciences 275: 1865–74
6. Federation of Laboratory Animal Science Associations (2002). Working Group on Health Monitoring of Rodent and Rabbit colonies. Recommendations for the health monitoring of rodent and rabbit colonies in breeding and experimental units. Laboratory Animals 36: 20–42
7. Clifford CB and Watson J (2008). Old enemies, still with us after all these years. ILAR Journal 49: 291–302
8. GV-SOLAS (1999). (German laboratory animal science association) Implications of infectious agents on results of animal experiments. Laboratory Animals 33 (suppl. 1): S1.39–87
9. Kent M, Feist S and Harper C (2009). Recommendations for control of pathogens and infectious diseases in fish research facilities. Comparative Biochemistry and Physiology Part C Toxicology & Pharmacology 149: 240–48
10. Swallow J, Anderson D, Buckwell A, Harris T, Hawkins P, Kirkwood J, Lomas M, Meacham S, Peters A, Prescott M et al. (2005). Guidance on the transport of laboratory animals: report of the Transport Working Group established by the Laboratory Animal Science Association (LASA). Laboratory Animals 39: 1–39
11. Barthold SW (2008). Microbes and the evolution of fancy mice. ILAR Journal 49: 265–71
12. Reuter JD and Dysko RC (2003). Quality assurance/surveillance monitoring programs for rodent coloines. In Laboratory Animal Medicine and Management, J Reuter J and MA Suckow (eds). www.ivis.org/advances/Reuter/reuter3/chapter.asp?LA=1
13. Gordon JW (2002). Barrier facilities for rodents in academic facilities: a two edged sword. Comparative Medicine 52: 397–402
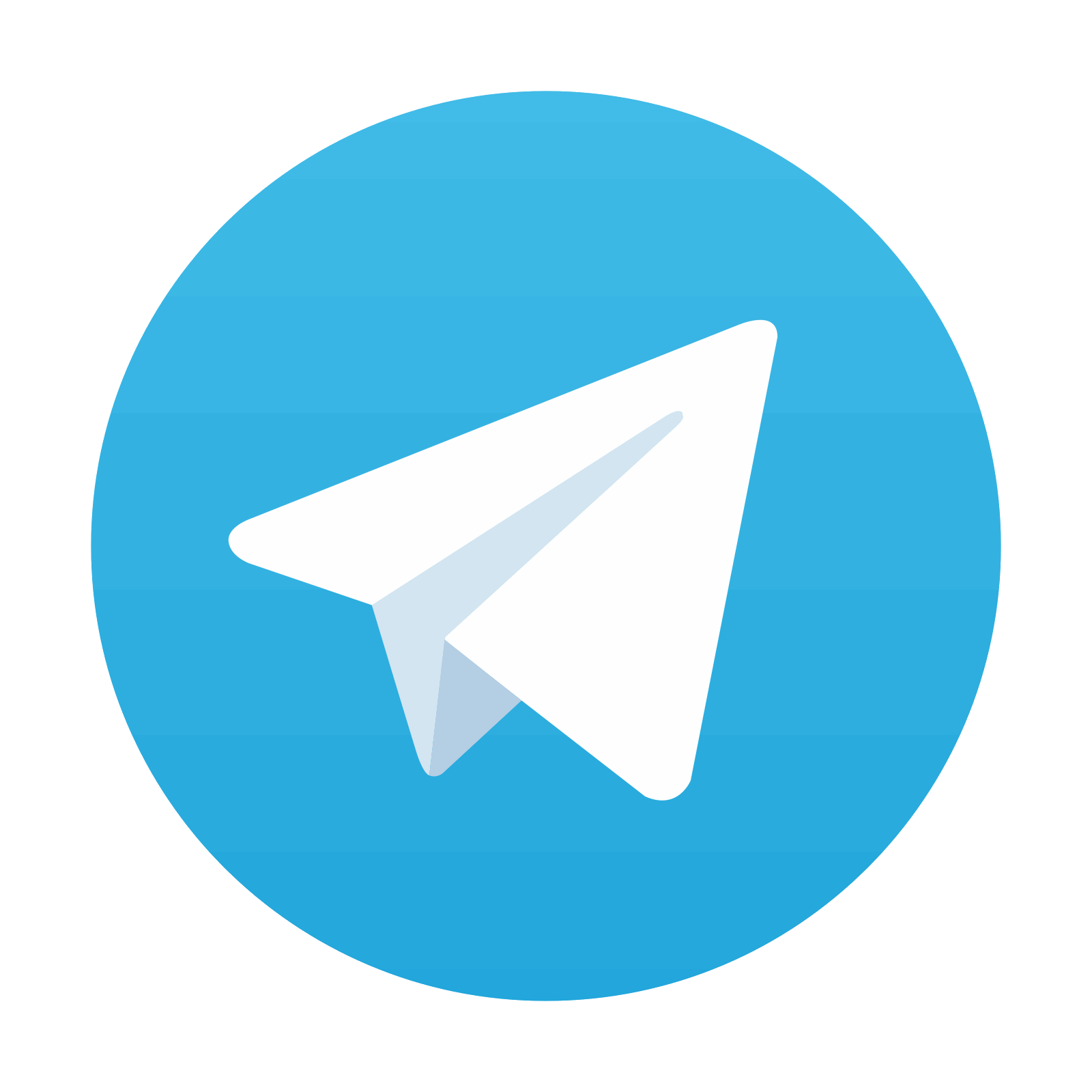
Stay updated, free articles. Join our Telegram channel

Full access? Get Clinical Tree
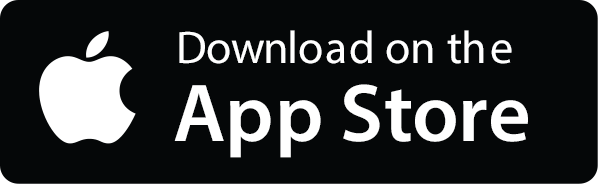
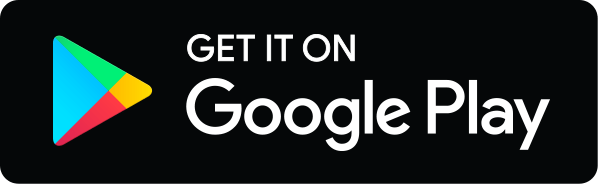