Fig. 11.1
Approaches to the development of universal vaccines against influenza A viruses. Antibody-based universal vaccines can be developed using various targets such as HA fusion peptides and stalk domains, ectodomain of M2, NP, NA and M1 proteins. Recently, efforts have also been directed to develop T cell-based universal vaccines that will protect the host by inducing cell-mediated immune response
The primary objective of the development of universal influenza vaccines is to induce broadly neutralising antibodies (Corti and Lanzavecchia 2013). However, prior influenza immunity may have its effect on the induction of such broadly protective antibodies. It was speculated that the pre-existing immunity produced in response to previous exposure to influenza virus or vaccine may impede the generation of broadly neutralising antibodies; and therefore can only be used in very young children having restricted exposure to influenza viruses or vaccines. However, mice and ferrets having pre-existing immunity to influenza virus produced broadly neutralising influenza antibodies when a prime-boost vaccine formulation, consisting of a DNA vaccine for priming and an inactivated seasonal vaccine as booster, were used. The source of pre-existing immunity whether due to natural exposure to a flu virus or in response to vaccination was inconsequential. These influenza-immune ferrets that had received the prime-boost vaccine preparation, developed broadly neutralising antibodies against the conserved stem region of HA, and showed protection to challenge with heterotypic influenza viruses. It, therefore, opens up the prospects of developing universal influenza vaccines for humans using the conserved stem region of HA despite previous influenza exposure (Wei et al. 2012).
Induction of broad-spectrum heterosubtypic immunity by T-cell-based vaccines is another approach (Berthoud et al. 2011; Goodman et al. 2011; Hillaire et al. 2011; Lillie et al. 2012; McKinstry et al. 2012; Cargnelutti et al. 2013). However, the rapid replication of influenza viruses in the host resulting in the development of clinical signs prior to development of effective cell-mediated immune response that restricts viral replication is a challenge to the design of such vaccines. Therefore, the vaccination with these set of vaccines must induce and maintain T cells in a high effector state without causing immunopathology (Subbarao et al. 2006; Tan et al. 2013). Because CD8+ T cell epitopes are often derived from highly conserved regions of influenza viruses, such vaccines need not be reformulated annually and, unlike current antibody-inducing vaccines, could provide cross-protective immunity against newly emerging pandemic viruses. A single vaccine which contains a combination of several viral immunogens that involves and stimulates different parts of the immune system would be much better than a single immunogen vaccine (Goodman et al. 2011). Identification of an immune correlate of protection and the methods to measure it is another important area that must be looked into for the T-cell-based universal vaccines (Sridhar et al. 2013; Subbarao and Matsuoka 2013).
Seasonal and pandemic influenza can be prevented primarily by vaccination (Geary and Beckett 2012). A universal vaccine that elicits broad protective immunity against multiple subtypes of influenza A viruses is highly desired. Broadly neutralising ‘heterosubtypic’ antibodies (BnAbs) have been recognised that bind to a extremely conserved regions on the stalk of HA present in all Group 1 influenza viruses (Sui et al. 2009; Ekiert et al. 2009) and inhibit virus replication by blocking virus–host cell membrane fusion. The identification of these broadly neutralising antibodies recognising the stalk domain of influenza virus HA has given impetus to develop a universal influenza virus vaccine in which only these epitopes are present, but the variable and immunodominant epitopes situated in the globular head of HA are absent.
There are 18 subtypes of HAs that have further been classified into two Groups. The Group 1 HA subtypes are H1, H2, H5, H6, H8, H9, H11, H12, H13, H16, H17 and H18; the Group 2 HA subtypes are H3, H4, H7, H10, H14 and H15 (Fig. 11.2). The influenza virus haemagglutinin molecule possesses two structurally distinct domains: (i) a globular head domain and (ii) a stalk domain at the membrane-proximal region. The globular head is composed of part of HA1, and the stalk structure is made up of portions of HA1 and all of HA2 (Wilson et al. 1981). The sialic acid binding pocket present in the globular head domain helps the virus to attach to the host cell, whereas the, HA2 fusion peptide present in the stalk domain, causes the fusion of the viral envelope with the endosomal membrane of the cell. These functions allow the virus to enter the host cell and production of progeny virions subsequent to replication, transcription and translation of the viral genome. Majority of the antibodies produced after influenza virus infection are directed against specific immunodominant regions located in the globular head domain of the HA and are strain specific. However, generation of broadly neutralising antibodies against various epitopes located on the HA stalk domain of the influenza virus have been reported (Corti et al. 2011; Ekiert et al. 2011; Sui et al. 2011; Wrammert et al. 2011).
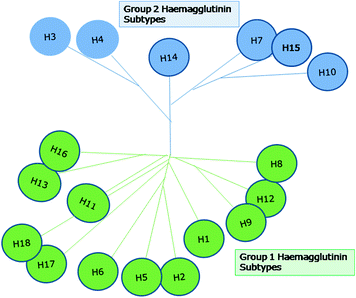
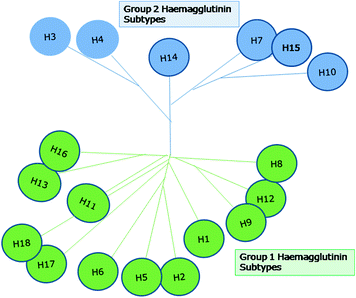
Fig. 11.2
Classification and phylogenetic relationship of various influenza A virus haemagglutinin subtypes. There are 18 subtypes of HAs that have further been classified into two groups. The group 1 HA subtypes are H1, H2, H5, H6, H8, H9, H11, H12, H13, H16, H17 and H18; the group 2 HA subtypes are H3, H4, H7, H10, H14 and H15
It has been reported that the human immune system can produce BnAb, not only to the conserved areas on the HA stem of Group 1 viruses, but also to unknown common epitope(s) present in Group 1 and 2 influenza A viruses (Sui et al. 2011). Recently recombinant influenza viruses expressing chimeric haemagglutinins encompassing a variety of globular head and stalk mixtures have been generated, not only from different haemagglutinin subtypes but also from diverse haemagglutinin phylogenetic groups (Hai et al. 2012).
Full coverage and protection is not conferred by the current flu vaccines against SI virus strains. The identification of broadly neutralising antibodies such as VH1-69, that work against majority of group 1 influenza A viruses have been identified. A human neutralising monoclonal antibody (CR8020) with broad specificity against most group 2 viruses, including H3N2 and H7N7, has been reported. The epitopes in the HA stalk recognised by the VH1-69 group 1 antibodies and the CR8020 monoclonals are distinct. The development of a universal flu vaccine and broad-spectrum antibody therapies got a boost from this observation that most subtypes of influenza A can be neutralised by just a mixture of two antibodies (Ekiert et al. 2011).
Novel immunogens were constructed that contained epitopes from the HA stalk but lacked the variable and immunodominant epitopes located in the globular head of HA. Studies were conducted to determine whether the 20-residue A-helix of the HA2 chain that makes up the main constituent of the epitope of broadly neutralising antibodies CR6261, F10 and others is by itself adequate to induce antibodies with comparable broad antiviral activity. Antibodies produced by the mice immunised with VLPs exhibiting the A-helix identified multiple HA subtypes from group 1 but not from group 2; and the nature and properties of the induced antibodies was similar to those of CR6261 and F10, except that the anti-A-helix antibodies were not able to neutralise influenza virus. Therefore, further manipulation of the transplanted peptide, and presence of additional regions that display some more parts of the epitope, is required to achieve protection (Schneemann et al. 2012).
Studies were carried out to determine whether the various strains within the highly diverse H5N1 HPAI viruses are able to elicit broadly neutralising antibody by constructing DNA plasmids encoding codon-optimised haemagglutinin (HA) from 17 representative strains covering all reported clades and subclades. Mice immunised with the triclade DNA vaccine encoding HAs of (sub)clades 0, 2.3.2.1, and 7.2 produced broadly neutralising antibodies against all H5 clades and subclades and were protected by these induced BnAbs against challenge with a lethal heterologous H5N1 virus (Zhou et al. 2012).
Although the potential of influenza B viruses to undergo genetic changes and emerge into dangerous pandemic is quite low, however, they have a considerable involvement in the annual flu sickness in humans. Identification of broadly neutralising antibodies against influenza A viruses has paved the way for the prevention and control of influenza through development of monoclonal antibody-based immunotherapy and universal vaccines. However, two co-circulating, antigenically distinct lineages of influenza B viruses are largely responsible for the annual flu losses. Mice given three human monoclonal antibodies (CR8033, CR8071 and CR9114), were found to be protected against lethal challenge with both of these lineages. However, the nature of these monoclonals was different. The CR8033 and CR8071 antibodies identified distinct conserved epitopes in the head region of HA of the influenza B and prevented the exit of virus particles from infected cells. On the other hand, the CR9114 monoclonal was observed to bind with a conserved epitope in the HA stem and conferred protection against lethal challenge with influenza A and B viruses by preventing pH-triggered conformational change in the HA and subsequent fusion of the virus with the outer membrane of a host cell that is required for virus replication. The protection given by these antibodies against broad range of influenza virus strains indicated that they are directed against relatively conserved epitopes from one flu virus strain to the next. The information derived from these antibodies may help in building strategies for long-term protection against a variety of flu viruses prevailing over a long period of time, based on the development of monoclonal antibody-based immunotherapy and universal flu vaccines (Dreyfus et al. 2012).
In September 2012, it was reported that the scientists from The Scripps Research Institute and Sea Lane Biotechnologies have solved the co-crystal structure of a human antibody (code named: C05) that can neutralise influenza viruses in a unique way. They hypothesised that the bone marrow can provide the complete fossil record of all the antibodies made by a person in his lifetime. An all-inclusive library of billions of flu antibodies, from the internationally and locally collected bone marrow from patients, who during their lifetime, got infected with some important strains of flu, was generated. The unusual new antibody (C05) was isolated by screening this huge library for antibodies that could bind to proteins from a variety of influenza A viruses.
The C05 recognises and blocks the receptor binding site (RBS), located on the heads of viral haemagglutinins and is responsible for viral attachment to host cells. The RBS is a very important region on flu viruses and is comparatively much more exposed to the immune system than many other components and areas of the virus. Except for the small size of RBS relative to an antibody’s usual grip area, it makes up an ideal target for antibodies. The binding of the targets by the antibodies is done with the help of two arm-like structures, each of which is made up of six protein fingers or loops. Both the RBS and some of the adjoining areas in the head, which differ among various flu strains, have to be captured by a usual antibody. The firm grip obtained by an antibody on this region for one flu strain generally will be lost due to mutation in the virus strain. This is the reason for the shift of focus more on the haemagglutinin stalk than on the head for the development of universal flu vaccine.
Instead of capturing the hypervariable regions around the flu RBS, the C05 uses a single-elongated protein loop to reach in and make a one-handed or one-fingered seize of the RBS itself. The antibody appears to produce maximum effect when two of these active loops, one on each arm, grab two viral RBSs on separate haemagglutinins, indicating a need for these antibodies to cross-link two haemagglutinins. The important function of the RBS does not allow it to change much from strain to strain; and thus variety of different harmful influenza A viruses can be neutralised by C05. Therefore, the C05 and other similar more potent antibodies may be used for protection from severe influenza infections. A universal flu vaccine designed to elicit such antibodies in people should be significantly more effective. Detailed studies on the mechanisms of action of such a broadly neutralising antibody has revealed that the replication of flu virus is inhibited by prevention of the membrane fusion during viral entry by insertion of the heavy chain of these antibodies into a conserved pocket in the stem region of virus HA (Ekiert et al. 2009; Sui et al. 2009).
The C05 also protected cells cultures infected with these flu viruses. Relatively low doses of C05 prevented infections in mice even after exposure to a lethal dose of influenza A virus. It was also found to confer complete post exposure protection of infected mice when this antibody was given up to 3 days post exposure.
It is not only important to select the correct target for the universal influenza vaccines, but the vaccine delivery method has to be unique and innovative as these conserved epitopes that might provide broad heterosubtypic immunity, are weak immunogens. The objectives, prospects and expectations of a commercial universal vaccine, whenever these become available, need to be well defined. Who should be the target group for such vaccine, whether there is a need for booster or revaccination and when should it be undertaken. What should be the life of such a vaccination before its composition and formulation needs updating (Subbarao and Matsuoka 2013).
11.5 Treatment
No specific drug therapy is practiced in birds and/or animals.
To reduce the secondary bacterial infections, antibiotics and supportive therapy have been recommended.
Anti-Flu drugs (Amantadine, Rimantadine, Zanamivir/Relenza and Oseltamivir/Tamiflu) can be given for both prevention of people from getting infected and treatment purpose also. They can turn the illness into a milder form and help in preventing serious complications. These must strictly be prescribed by a medical doctor only. Antipyretic medications and suitable anti-inflammatory drugs are also prescribed.
Relenza and Tamiflu are NA inhibitors, which hold back the flu viruses from reproducing within the host cell, and are found to be most effective. Tamiflu is the drug of choice.
11.5.1 Treatment for Human Influenza Viruses
There are two classes of antiviral drugs: NA inhibitors (Zanamivir and Oseltamivir) and adamantanes (Amantadine and Rimantadine), which inhibit a viral protein called Matrix-2 (M2). These commercial antiviral drugs for treatment of the human flu are available only on the prescription of a medical doctor (Monto 2003; Das 2012; Nguyen et al. 2012). Adamantanes were used initially in the outbreak of H5N1 in Hong Kong in 1997 (Yuen et al. 1998). Amantadine and Rimantadine are effective against many subtypes of human influenza A viruses but not against H5N1 (Li et al. 2004), H1N1 (Parmar et al. 2011), or influenza B or C viruses (Vargese et al. 1983). These two drugs exert their antiviral effect by two mechanisms. First, the release of transcriptionally active ribonucleoprotein complex for transport to nucleus is prevented by blocking the influx of the H+ ions into the core of the virion from the acidified endosome due to its effect on viral M2 protein. Second, the HA maturation during transport from the ER to the plasma membrane is blocked (Hay 1992). However, resistance of these two drugs to influenza viruses has increased to a very large extent and is widespread (Anonymous 2006; Weinstock and Zuccotti 2006; Ilyushina et al. 2007; Lan et al. 2010; Nguyen et al. 2012); and it is due to L26I or S31 N mutations of the M2 protein. The binding of the drug is decreased by S31 N mutation (Pielak et al. 2009). The other two drugs Zanamivir and Oseltamivir exert their antiviral action against all influenza A virus subtypes (including H5N1) and influenza B viruses by inhibition of the viral NA (Tumpey et al. 2002; Moscona 2005a, b; Parmar et al. 2011; Hsu et al. 2012; Vijayan et al. 2012). Oseltamivir can be taken orally, but the optimal effect of zanamivir can be achieved after inhalation or intranasal administration only (Hsu et al. 2012). Various other NA inhibitors viz., peramivir and CS-8958 are also active against the H5N1 virus (Boltz et al. 2008; Kiso et al. 2010a). NA inhibitors can be used to combat pandemic influenza (Democratis et al. 2006). However, the resistance of influenza A viruses (Gupta et al. 2006; Monto et al. 2006; Alexander et al. 2007; Sheu, et al. 2008; Stittelaar et al. 2008; Fleming et al. 2009; Hauge et al. 2009; Burch et al. 2009; Naughtin et al. 2011; Nguyen et al. 2012), and influenza B viruses (Hatakeyama et al. 2007; Sheu et al. 2008) to NA inhibitors has been reported. Oseltamivir resistance can emerge and leads to treatment failure more commonly due to H274Y and N294S (N2 numbering) and less frequently due to V116A, I222L, K150 N and S246 N substitutions within the NA (de Jong et al. 2005; Renaud et al. 2011; Nguyen et al. 2012; Jarhult 2012). Oseltamivir resistance was observed in majority of the human influenza A (H1N1) viruses during the 2008–2009 influenza seasons in the United States (Anonymous 2009a) and Australia (Hurt et al. 2012). The S-OIV is susceptible to oseltamivir and zanamivir but resistant to the adamantanes (Anonymous 2009b). Pyrosequencing is used to determine the molecular markers of antiviral resistance in influenza A (H5N1) viruses (Deyde et al. 2009). Therefore, it becomes crucial to monitor the antiviral resistance among the field influenza viruses (Hurt et al. 2012; Nguyen et al. 2012; Jarhult 2012) to inform public health strategies for the control of influenza infections. Studies have been conducted to determine the effects of combination therapy for highly pathogenic H5N1 influenza virus infection in mice and cell cultures (Ilyushina et al. 2008; Smee et al. 2009). The antiviral activity of Carbocyclic Cytosine nucleosides against H5N1 has been demonstrated (Chu et al. 2008; Rao et al. 2009). Corticosteroids, when given for a long period of time or at higher doses can cause serious adverse reactions in influenza A (H5N1) virus-infected patients. Antibiotic may be given for suspected bacterial co-infection in patients with A(H5N1) virus infection. Oxygen may be given for correction of hypoxemia (WHO 2007). Apart from these, rest, good plan of nutrition, quitting smoking and adequate exercise will help the body to fight against influenza viruses.
The use of viral M2 inhibitor, adamantanes has been discontinued. The NA inhibitors are facing increased resistance, and the number of such mutants is feared to increase rapidly due to their indiscriminate and continuous use, putting the population at risk to a drug-resistant epidemic (Baranovich et al. 2011; Sheu et al. 2011). The development of increased resistance to FDA-approved antiviral drugs against influenza virus has encouraged a paradigm shift in the strategies for the development of antiviral drugs for influenza virus (Barik 2012; Eyer and Hruska 2013; Hayden 2013; Motohashi et al. 2013; Lee et al. 2014b). Various approaches have been used to develop antivirals against influenza viruses (Fig. 11.3). The inhibitors of cellular factors and host signalling cascades that directly or indirectly interact with virus replication are potential new development candidates (Karlas et al. 2010; Ludwig 2011; Shaw 2011; Muller et al. 2012; Lee and Yen 2012; Loveday et al. 2012; Beyleveld et al. 2013; Edinger et al. 2014; Terrier et al. 2013, Zhao et al. 2013). The cellular miRNAs have been shown to inhibit influenza viral replication by degradation of the viral PB1 gene (Song et al. 2010). The siRNA have been observed to inhibit influenza virus replication targeting the M and NP genes, and thus can be used as potential antiviral therapeutic agent against influenza virus (Ge et al. 2004; Barik 2010; Raza et al. 2011). A cocktail of multiple drugs against influenza virus, which may target two viral functions or one viral and one cellular function, can be developed (Nguyen et al. 2010). New compounds have been screened against various viral targets, such as NA (Kiso et al. 2010b; Ikematsu and Kawai 2011; Ivanenkov et al. 2013; Chen et al. 2014), HA (Prasad et al. 2013; Shen et al. 2013; Zhu et al. 2012; Yang et al. 2013a), the NP (Kao et al. 2010; Chenavas et al. 2013), M2 ion channel (Balannik et al. 2009), and NS1 (Jablonski et al. 2012). Nucleozin was found to trigger the aggregation of NP and inhibits its nuclear accumulation that is required for influenza virus replication (Kao et al. 2010). A mathematical model has been developed for building an antiviral strategy against influenza A infection (Hur et al. 2013).
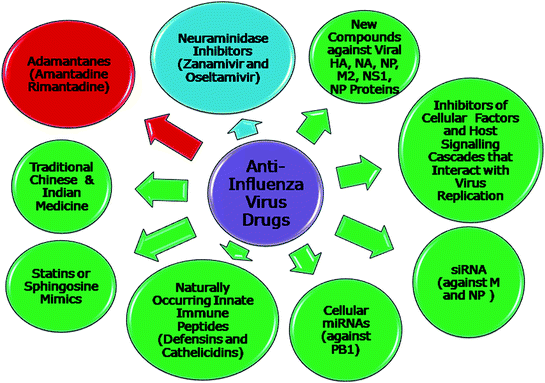
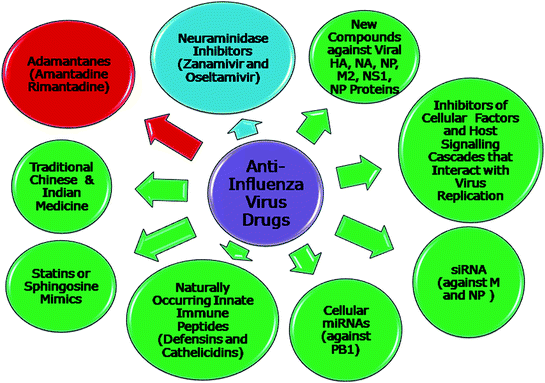
Fig. 11.3
Various approaches (past, present and future) to anti-influenza virus drugs. The admantanes have been discontinued. Currently, NA inhibitors are being used. But resistance against them has started to built in. A variety of approaches as mentioned in the illustration are being developed
Various novel peptides that inhibit influenza viruses have also been documented (Jones et al. 2006; Rajik et al. 2009; Matsubara et al. 2009, 2010; Triana-Baltzer et al. 2009). Defensins and cathelicidins, the naturally occurring innate immune peptides, have been tried as antivirals against influenza virus, though their mechanism of action has been observed to be different (Salvatore et al. 2007; Barlow et al. 2011; Tripathi et al. 2013). The antiviral activity of serum amyloid P against influenza A viruses has recently been demonstrated (Job et al. 2013). A different approach in the form of immunomodulatory therapy against severe influenza has been reported (Fedson 2006, 2013; Marsolais et al. 2009; Darwish et al. 2011; Viasus et al. 2011; Walsh et al. 2011) in which statins or sphingosine mimics have been used with the assumption and hypothesis that the cytokine-storm induced mortality and morbidity will be reduced. Broad subtype-specific DNA aptamers that bind with high affinity to influenza A viruses have recently been described (Shiratori et al. 2014). Traditional Chinese Medicine (Ge et al. 2010; Yang et al. 2013b; Lu et al. 2014b) and plant-based compounds (Song et al. 2005; Hsu et al. 2010; Lee et al. 2013), have also been tried for the treatment of influenza virus. Indian traditional system of medicine, Ayurveda, promotes the immunity of the host and involves the intake of herbs basil (Ocimum basilicum), Ginger (Zingiber officinalis), garlic (Allium sativum), gooseberry (Embelica officinalis), aloevera, camphor and eucalyptus oil (Parmar et al. 2011), Ginkgo biloba leaf extract (Haruyama and Nagata 2012), Red Sea grass (Thallasodendron ciliatum) (Ibrahim et al. 2013), flavanoids of various plants (Costa et al. 2012) and acidic polysaccharides from Coccomyxa gloeobotrydiformi, a green alga (Komatsu et al. 2013). The intake of these herbs may have beneficial effects during influenza infection.
11.6 Pandemic Preparedness
The WHO uses certain parameters and criteria to define various phases of influenza virus pandemic (Fig. 11.4). Rough guidelines for the responses that may be undertaken during a pandemic cycle have been documented (Fig. 11.5). Influenza is classified as a C category agent in potential bioterrorist agent (Rotz et al. 2002). Although the past pandemics of influenza were events naturally occurring but an influenza strain deliberately altered can lead to initiation of pandemic. With the increase in transportation facilities, urbanisation and introduction of new influenza virus subtypes (Starbuck et al. 2012), WHO and health authorities have recognised that the world is under threat of influenza pandemic worldwide, which could potentially have serious impact on health of human as well as animal population (Patriarca and Cox 1997). During pandemics of influenza in the twentieth century, death toll was millions and enormous social as well as economic losses were incurred globally. The forecasting of pandemic influenza virus, its pathogenicity and the extent of susceptible population is very difficult. So, the pandemic preparedness for influenza may help in reduction of virus transmission, and thus reduce the clinical cases and deaths. The preparedness varies from country to country and requires the commitment and input facilities. Planning healthcare for influenza pandemic is quiet tough and is not be possible within days or a month but require multicentric approach involving government, experts from various departments viz., policymakers, legislative persons, human and animal health professionals, pathology laboratories, extension workers and volunteers. On healthcare resource capacities, the AsiaFluCap Simulator can provide evidence-based and illustrative information during future pandemics and help to generally understand dynamics in resource capacities (Stein et al. 2012).
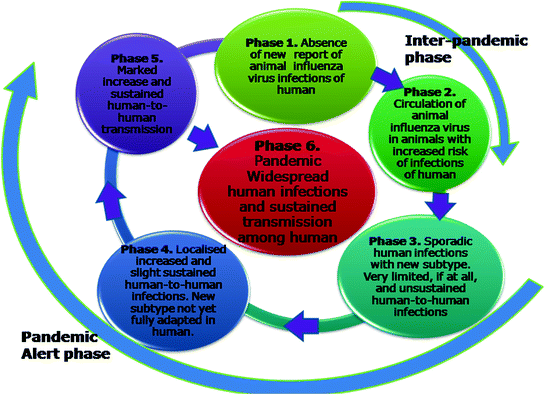
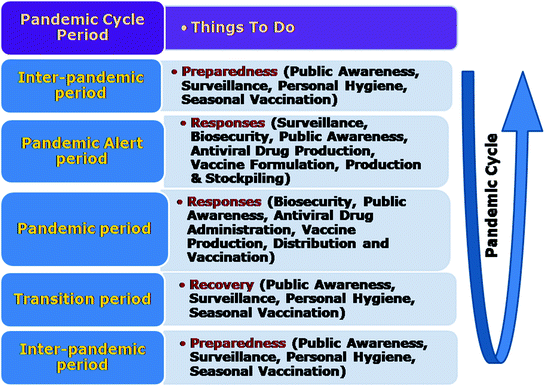
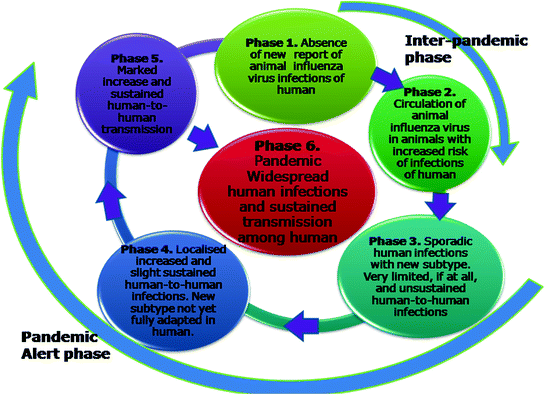
Fig. 11.4
Pandemic phases as classified by World Health Organization. The World Health Organization has classified the influenza virus pandemic into six phases, 1–6, using certain parameters and criteria as shown in the illustration. The Phases 1–2 are inter-pandemic phases. The phases 3–5 are pandemic alert phases and on crossing the 5th phase, the WHO declares a new pandemic of influenza virus
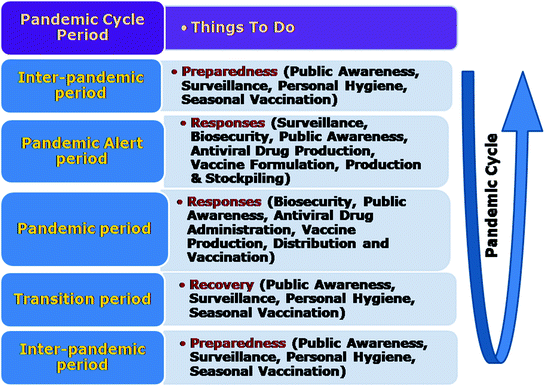
Fig. 11.5
Pandemic cycle and various responses to be undertaken. A pandemic cycle consists of inter-pandemic period in which continuous surveillance is carried out, general public is made aware of the benefits of personal hygiene and seasonal vaccination is done. During the pandemic alert in addition to the above, drug production, vaccine formulation, production, stockpiling and administration is carried out. During the pandemic period, besides the above legislation enforcement, vaccine distribution and vaccination and drug administration is done. The transition period is the period of recovery
Sound epidemiological approach is the basis for pandemic awareness for controlling HPAI in Asia and other parts of the world but complete eradication is difficult due to the presence of the virus in wild reservoir birds. The range of epidemiological scenarios existing in different systems of poultry production in several Asian countries is considered in this approach. A high incidence of disease with greater frequency of outbreaks in poultry and humans are included in the epidemiological scenarios along with incidence of disease at low frequency; sporadic outbreak of disease or risk of contracting disease. Combined accurate disease control options (FAO Position paper: Recommendations on the prevention, control and eradication of HPAI in Asia, September 2004, http://www.fao.org/ag/againfo/subjects/en/health/default.html ) is received via approval and support of OIE. Depending on the stage at which various nations and farming systems have reached along with the variable disease status, this approach received both approval and support of OIE.
Following the outbreaks of SARS, HPAI and swine flu (H1N1), many countries learned lessons from these outbreaks but there is always a space for further improvement so there should be regular revision of pandemic preparedness which may be divided into following steps:-
Emergency preparedness. For starting of pandemic preparedness for any disease, human resources (policy making) as well as financial resources (funding) are important and need to be taken care of. The persons should be identified from various organisations for contribution in the planning. For influenza pandemic preparedness planning, the persons to be included are epidemiologists, virologists, experts from human and animal health professionals, administration, military and paramilitary persons, representatives of NGOs, press and media persons and schools (Rebmann et al. 2012). The proper coordination among these persons requires appropriate command and control structure. For carrying out essential functions, there is a need to develop standard operational procedures and role. Individual and group responsibilities during a pandemic should be known to all. In order to fill the gaps in knowledge about the influenza pandemic and especially vaccine in poorer nations, extension works should be done where considerable differences in education and access to media are evident (Kouassi et al. 2012; Cantey et al. 2013; Sims 2013).
Surveillance. Surveillance is collection of data along with their interpretation and dissemination for developing the evidence-based interventions. In influenza pandemic, surveillance for influenza-like illness (ILI) must be established in man and animals along with creation of links between animal and human health professionals. The highly dynamic nature of influenza viruses demands regular systematic surveillance of influenza in both humans as well as animals for getting all-inclusive picture of the prevalence of influenza viruses. For this, there is a need for simple and easy to perform test for the characterisation of influenza virus that has recently emerged (Mak et al. 2012).
Case investigation and their treatment. For confirmation of suspected influenza cases, diagnostic laboratories for human as well as animal should be well equipped with immunofluorescence (IF); reverse transcriptase polymerase chain reaction (RT-PCR) testing along with training facilities. Daily reporting of the influenza cases is compulsory. To ensure treatment of the affected individuals, clear-cut guidelines should be there.
Prevention of disease spread in the community. In case of influenza pandemic, medicines and vaccines are limited in developing countries, so the focus should be on non-medical interventions to check the spread of the disease. In animals, it may be possible up to some extent, but in humans, it affects the behaviour and rights of humans and needs firm educational and legal support. There is a need to strengthen the personal respiratory hygiene. Enzootic circulation of HPAI viruses at present requires increased ability to produce pandemic influenza vaccine globally which becomes one of the important components of pandemic preparedness plans, and its targeted use during pandemic alert or in early pandemic situation is likely to mitigate the consequences of an influenza outbreak (Plosker 2012). There must be some strategies to ensure rapid and quick supplies of vaccines, their distribution and importation to countries that may bear the brunt of a future epidemic (Mihigo et al. 2012; Sims 2013).
Border controls. In the event of an outbreak of HPAI, the movement of birds and products internationally is controlled as per OIE recommendations. Countries must restrict import of poultry and products from infected countries until safe trade recommences, which will depend on whether or not the infected country or countries are adhering to recommendations laid down by OIE. Border control measures decrease but cannot rule out risk of infection. Entire banning of smuggling cannot be done. In some instances, advanced measures to quarantine may give rise to a lucrative environment for smuggling especially if there is shortage of products. Geographical, economical, social and political reasons, individually or collectively, ensure reasonably well-secured borders. The chances of borders leakage must be thought by veterinary authorities while disease control and prevention strategy are developed. International borders cannot be identified by wild birds. Veterinary authorities must take steps to prevent infected wild bird population from coming in contact with domestic poultry and transmitting the infection to them (WHO 2005).
Maintaining essential services. To keep a society going about with their daily chores, essential services are required. To reduce the morbidity and case fatality rates, it is very important that health services should be functional all the time. The requirements of human and animal healthcare professionals, their sources and their training, disposal of corpse, animal carcasses and their infected belongings etc., have to be identified in advance. Thorough understanding of the contributory factors to the willingness of healthcare authorities to report to work during a public health crisis is significant in planning for pandemic preparedness (Devnani 2012). Duty to healthcare has been identified as a pressing ethical issue in contemporary discussions of pandemic preparedness, and women still dominate the front lines of healthcare work (Godderis and Rossiter 2012). There is a need of tight coordination, communication, integration, and alignment in any management structure (Fieldston et al. 2012; Sims 2013).
Research and evaluation. During a pandemic, the country is having an extra burden on resources to control the disease but this situation might help in understanding of the disease and effect of preventive measures, which will contribute to global knowledge. The research may include virus transmission (Fouchier et al. 2012; Van Gageldonk-Lafeber et al. 2012), antigenic and molecular characterisation of virus strain, antiviral drug resistance, vaccine efficacy and socio-economic impact of the pandemic etc.
11.7 Seasonal Influenza
Apart from the influenza virus pandemics that occur only very rarely, the annual/SI infections occur every year and are responsible for lots of morbidity, deaths and huge direct and indirect economic losses. In temperate regions, low-level flu infection/disease may occur throughout the year but the peak occurs in January/February with gradual increased incidence being observed between October to March. Thus, there is a seasonal aspect to influenza virus induced disease in temperate regions. The seasonality of influenza and its effects and impact in the tropical regions is less obvious (Cox and Subbarao 2000). The understanding of the seasonality of influenza may provide greater knowledge about the maintenance, spread and transmission of the disease (Naumova 2006) that in turn will help in putting in place better preventive and control measures. Although many theories based on influenza virus biology, host immunity at individual and population level that are influenced by factors such as photoperiod, nutritional status, crowding, ambient temperature, indoor heating, air travel, bioaerosols and paths of global wind streams (Dowell 2001; Hammond et al. 1989) and many mathematical models such as seasonal forcing, dynamical resonance, bifurcation phenomenon (Dushoff et al. 2004; Smith 2003, 2006) have been described, yet none of them have been able to completely address the mystery of seasonality of influenza (Lofgren et al. 2007).
Every year simultaneous co-circulation of a number of different influenza virus strains in the human population is observed. The protective immunity to influenza viruses is of short duration, homotypic and is mainly mediated by antibodies. Therefore, cross-protection by antibodies generated against one influenza virus type, or subtypes of influenza A virus normally does not occur against another type or subtypes of influenza A virus or their antigenic variants (Couch and Kasel 1983). The accumulated point mutations are responsible for the frequent appearance of antigenic variants causing the annual epidemics of seasonal influenza. It is for this reason that the influenza vaccines are reformulated in most seasons to include those influenza virus strains that are expected to prevail and cause disease during the upcoming season.
SI is an acute viral infection mainly of the upper respiratory tract caused by subtypes of influenza A virus and influenza B virus strains. Presently, the H1N1 and H3N2 among the many subtypes of influenza A virus are responsible for seasonal influenza. Although SI can occur in age group, but children <2 years, adults >65 years, and people of any age with diabetes, or some chronic diseases of heart, lung, kidney, liver or immune-incompetent persons constitute the high-risk group. The main clinical signs and symptoms of SI are abrupt onset of pyrexia, dry cough, sore throat, rhinitis, headache, myalgia and severe malaise. Except for the high-risk groups where severe complications, pneumonia and death may occur, most other healthy infected adults do not need medical care and natural recovery occurs within 7 days. A rough estimate of annual influenza-associated deaths ranging between 3,000 and 49,000, during 30 seasons from the 1976–1977 season through the 2005–2006 season, have been reported (CDC 2010). The economic burden due to SI is due to complications and death in high-risk group as well as productivity losses on account of absence of the workforce. Antiviral drugs and vaccines are available for prevention of SI. Vaccines are quite effective in healthy adults and can prevent 70–90 % of influenza-specific illness in them. Reduction of severity and complications by up to 60 % and mortality by 80 % has been observed in elder persons through vaccination against SI.
The selection of the virus strains that will constitute the SI combined vaccine regimen by the World Health Organization (WHO) is a complex process and involves the national influenza centers, five WHO Collaborating Centers for Reference and Research on Influenza, Essential Regulatory Laboratories (Figs. 11.6 and 11.7). After detailed deliberations and consultations in the month of February of each year, the recommendations for the SI vaccine’s composition are given by the WHO. The timeline for the manufacture of SI virus vaccine is shown in Fig. 11.8.
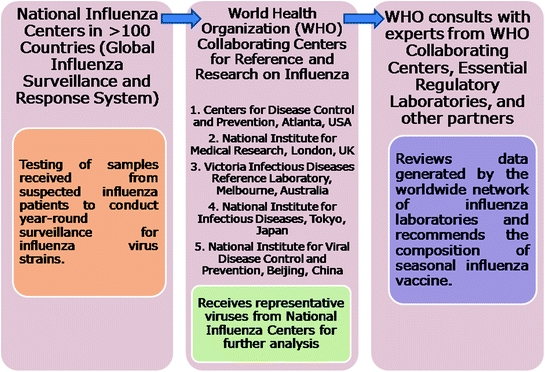
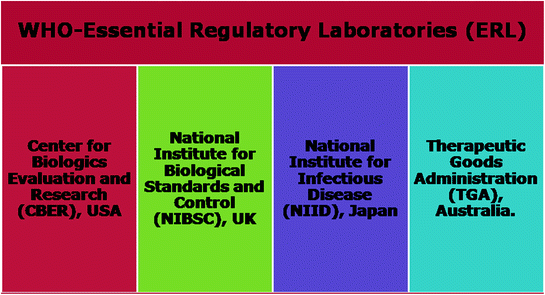
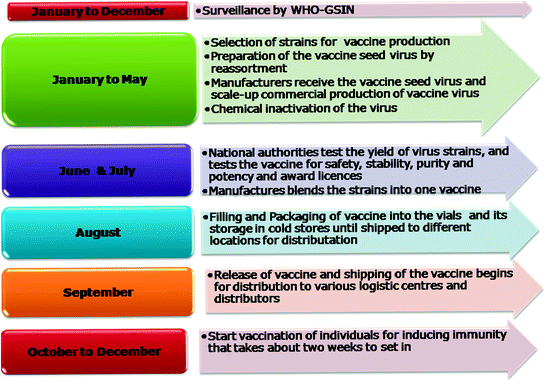
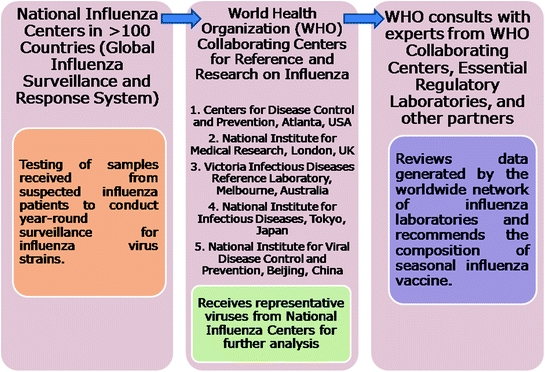
Fig. 11.6
Selection of virus strains for the seasonal influenza vaccine
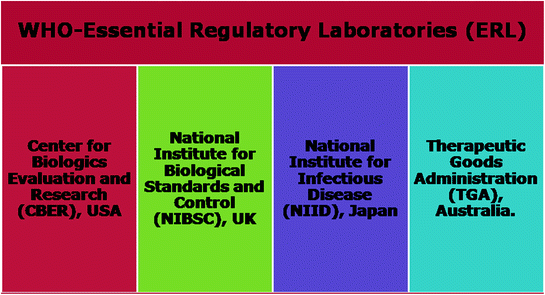
Fig. 11.7
Essential regulatory laboratories that help WHO in selection of the composition of seasonal influenza vaccine
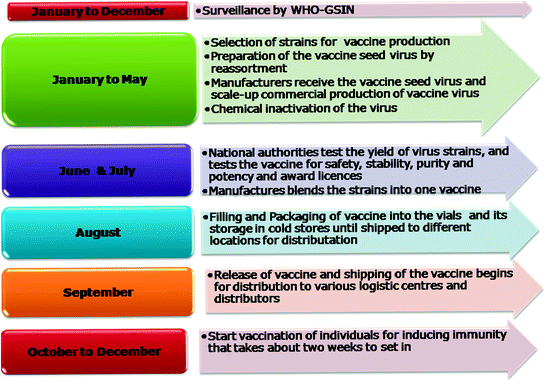
Fig. 11.8
Manufacturing timeline for the seasonal influenza vaccine
The WHO Vaccine Composition Meeting for Northern Hemisphere 2013–2014 and Southern Hemisphere for 2014 influenza season vaccines were held on February 21, 2013 and September 23–26, 2013, respectively, at WHO headquarters in Geneva, Switzerland (WHO 2013). The recommended composition for both the hemispheres was the same. The Vaccines and Related Biological Products Advisory Committee (VRBPAC) a part of the USFDA on February 27, 2013 approved the WHO recommendations for this year’s seasonal flu vaccine for the United States (FDA 2013). The equivalent agency to VRBPAC of U.S. is the European Centre for Disease Prevention and Control (ECDC) for the EU/EEA countries for this purpose.
For the 2013–2014, the SI vaccines can be Inactivated Influenza Vaccine (IIV), Live Attenuated Influenza Vaccine (LAIV) grown in embryonated eggs, or cell culture (cc) and a recombinant vaccine (Fig. 11.9). The inactivated influenza vaccines can be trivalent (IIV3) or quadrivalent (IIV4). Similarly quadrivalent live attenuated influenza vaccines (LAIV4) are available for the 2013–2014 season (Grohskopf et al. 2013). The antigenically distinct Victoria and Yamagata lineages of influenza B provide only limited cross-protection. Unpredictability of the predominance of B virus lineage during a given season and limited cross-protection led to the inclusion of one strain from each of the two influenza B virus lineage in SI vaccines (Ambrose and Levin 2012; Block et al. 2012; Reed et al. 2012). Also, for the first time, an approved and licensed trivalent recombinant HA influenza vaccine (RIV3) containing purified HA proteins is available in U.S. for use against SI in persons aged between 18 and 49 years (Protein Sciences 2013). It can safely be used in persons who are allergic to eggs.
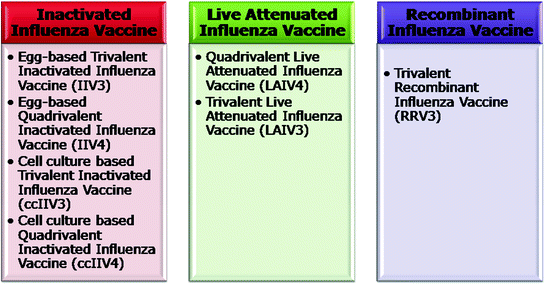
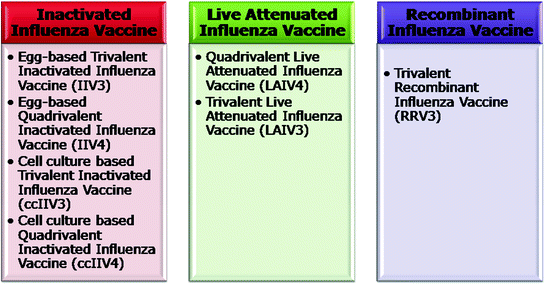
Fig. 11.9
Types of influenza virus vaccines available for 2013–2014 influenza season
Besides the RIV3, the other recently approved vaccines, including LAIV4, IIV4, trivalent cell culture-based inactivated influenza vaccine (ccIIV3) are expected to be in great demand in U.S. this season. Although, in those situations or target groups where more than one type of vaccine formulation is available, WHO is unbiased and does not recommend any favoured treatment to any category or manufacture of the influenza vaccines. It is estimated that production of about 135 million and 139 million doses of inactivated and live vaccine in the U.S. will occur for the current influenza season. The European Medicines Agency (EMA) is presently evaluating the quadrivalent LAIV. For the 2013–2014 influenza season, the ACIP has recommended routine annual influenza vaccination for all persons aged ≥6 months who do not have contraindications. The ACIP has, in its report, has also given specific recommendations and guidance on the use of alternative influenza vaccines for the high-risk groups etc. such as the persons aged ≥65 years and <2 years, pregnant women, persons allergic to eggs, and those with medical conditions that confer high risk for complications from influenza (Des Roches et al. 2012; Erlewyn-Lajeunesse et al. 2009; Jamieson et al. 2012; Grohskopf et al. 2013).
The 2013–2014 season influenza quadrivalent influenza vaccines will contain haemagglutinin obtained from the following:
an A/California/7/2009 (H1N1)-like virus,
an (A/Texas/50/2012 (H3N2) virus,
a B/Massachusetts/2/2012–like (Yamagata lineage) virus,
a B/Brisbane/60/2008–like (Victoria lineage) virus.
The trivalent influenza vaccines for 2013–2014 season influenza will be similar to the quadrivalent influenza vaccines except that this will not contain a B/Brisbane/60/2008–like (Victoria lineage) virus.
The composition of the influenza vaccines for the following influenza season is recommended two times a year, after analysing the influenza virus surveillance data generated by the WHO Global Influenza Surveillance and Response System (GISRS) after detailed discussion with advisory group of experts. The WHO meeting to deliberate on the composition of influenza virus vaccines for the Northern Hemisphere 2014–2015 is going to be held on 17–19 February 2014, in Geneva, Switzerland.
In order to create public awareness about the advantages of continuing influenza vaccination, National Influenza Vaccination Week (NIVW) is celebrated every year. The NIVW for last year was observed from December 8–14, 2013.
References
Alexander DJ (2001) Orthomyxoviridae- avian influenza. In: Jordan F, Pattison M, Alexander D and Faragher T (eds) Poultry diseases, 5th edn. WB Saunders, London, pp 272–279
Alexander ME, Bowman CS, Feng Z et al (2007) Emergence of drug resistance: implications for antiviral control of pandemic influenza. Proc R Soc B 274:1675–1684PubMedCentralPubMed
Ambrose CS, Levin MJ (2012) The rationale for quadrivalent influenza vaccines. Hum Vaccin Immunother 8(1):81–88
Anonymous (2006) High levels of adamantine resistance among influenza A viruses (H3N2) viruses and interim guidelines for use of antiviral agents—United States, 2005–2006 influenza season. MMWR Morb Mortal Wkly Rep 55:44–46
Anonymous (2009a) Update: influenza activity—United States, September 28, 2008-April 4, 2009, and composition of the 2009–2010 influenza vaccine. MMWR Morb Mortal Wkly Rep 58:369–374
Anonymous (2009b) Update: drug susceptibility of swine-origin influenza A (H1N1) viruses, April 2009. MMWR Morb Mortal Wkly Rep 58: 433–435
Atsmon J, Kate-Ilovitz E, Shaikevich D et al (2012) Safety and immunogenicity of multimeric-001—a novel universal influenza vaccine. J Clin Immunol 32:595–603PubMed
Balannik V, Wang J, Ohigashi Y et al (2009) Design and pharmacological characterization of inhibitors of amantadine-resistant mutants of the M2 ion channel of influenza A virus. Biochemistry 48(50):11872–11882. doi:10.1021/bi9014488 PubMedCentralPubMed
Bano S, Naeem K, Malik SA (2003) Evaluation of pathogenic potential of avian influenza virus serotype H9N2 in chickens. Avian Dis 47:817–822PubMed
Baranovich T, Webster RG, Govorkova EA (2011) Fitness of neuraminidase inhibitor-resistant influenza A viruses. Curr Opin Virol 1(6):574–581PubMedCentralPubMed
Barik S (2010) siRNA for influenza therapy. Viruses 2:1448–1457. doi:10.3390/v2071448 PubMedCentralPubMed
Barik S (2012) New treatments for influenza. BMC Med 10: 104. Published online 13 Sept 2012. doi:10.1186/1741-7015-10-104
Barlow PG, Svoboda P, Mackellar A et al (2011) Antiviral activity and increased host defense against influenza infection elicited by the human cathelicidin LL-37. PLoS ONE 6(10):e25333. doi:10.1371/journal.pone.0025333 PubMedCentralPubMed
Barr IG, Jelley LL (2012) The coming era of quadrivalent human influenza vaccines: who will benefit? Drugs 72(17):2177–2185PubMed
Belshe RB, Mendelman PM, Treanor J et al (1998) The efficacy of live attenuated, cold-adapted trivalent, intranasal influenzavirus vaccine in children. N Engl J Med 338:1405–1412PubMed
Belshe RB, Newman FK, Wilkins K et al (2007) Comparative immunogenicity of trivalent influenza vaccine administered by intradermal or intramuscular route in healthy adults. Vaccine 25:6755–6763PubMedCentralPubMed
Berthoud TK, Hamill M, Lillie PJ et al (2011) Potent CD8+ T-cell immunogenicity in humans of a novel heterosubtypic influenza A vaccine. MVA-NP+M1. Clin Infect Dis 52:1–7PubMedCentralPubMed
Beyleveld G, White KM, Ayllon J et al (2013) New-generation screening assays for the detection of anti-influenza compounds targeting viral and host functions. Antiviral Res 100(1):120–132PubMed
Block SL, Falloon J, Hirschfield JA et al (2012) Immunogenicity and safety of a quadrivalent live attenuated influenza vaccine in children. Pediatr Infect Dis J 31(7):745–751PubMed
Bodewes R, Kreijtz JHCM, Baas C et al (2009) Vaccination against human influenza A/H3N2 virus prevents the induction of heterosubtypic immunity against lethal infection with avian influenza A/H5N1 virus. PLoS ONE 4(5):e5538PubMedCentralPubMed
Boltz DA, Ilyushina NA, Arnold CS et al (2008) Intramuscularly administered neuraminidase inhibitor peramivir is effective against lethal H5N1 influenza virus in mice. Antivir Res 80:150–157PubMedCentralPubMed
Botteron CA, Aquilino J (2004) Avian influenza (the bird flu): a worldwide cause for concern. Report for the international foundation for the conservation of natural resources (IFCNR)
Boyce TG, Gruber WC, Coleman-Dockrey SD et al (1999) Mucosal immune response to trivalent live attenuated intranasal influenza vaccine in children. Vaccine 19:82–88
Bright RA, Carter DM, Crevar CJ et al (2008) Cross-clade protective immune responses to influenza viruses with H5N1 HA and NA elicited by an influenza virus-like particle. PLoS ONE 3:e1501PubMedCentralPubMed
Bright RA, Carter DM, Daniluk S et al (2007) Influenza virus-like particles elicit broader immune responses than whole virion inactivated influenza virus or recombinant hemagglutinin. Vaccine 25(19):3871–3878PubMed
Bunn D, Beltran-Alcrudo D, Cardona C (2011) Integrating surveillance and biosecurity activities to achieve efficiencies in national avian influenza programs. Prev Vet Med 98:292–294PubMed
Burch J, Paulden M, Conti S et al (2009) Antiviral drugs for the treatment of influenza: a systematic review and economic evaluation. Health Technol Assess 13(58):1–265PubMed
Campos-Outcalt D (2012) Battling influenza: changes for the 2012–2013 season. J Fam Pract 61(10):606–609PubMed
Cantey PT, Chuk MG, Kohl KS et al (2013) Public health emergency preparedness: lessons learned about monitoring of interventions from the national association of county and city health official’s survey of nonpharmaceutical interventions for pandemic H1N1. J Public Health Manag Pract 19(1):70–76PubMed
Capua I, Cattoli G, Marangon S (2004) DIVA-a vaccination strategy enabling the detection of field exposure to avian influenza. Dev Biol (Basel) 119:229–233
Capua I, Marangon S (2003) Vaccination policy applied for the control of avian influenza in Italy. In: Proceedings of a international symposium on vaccines for OIE list-A and emerging animal diseases. Ames, Iowa, USA, pp 213–219
Capua I, Marangon S (2006) Control of avian influenza in poultry. http://dx.doi.org/10.3201/eid1209.060430
Capua I, Marangon S, Dalla P et al (2000) Vaccination for avian influenza in Italy. Vet Rec 147:751PubMed
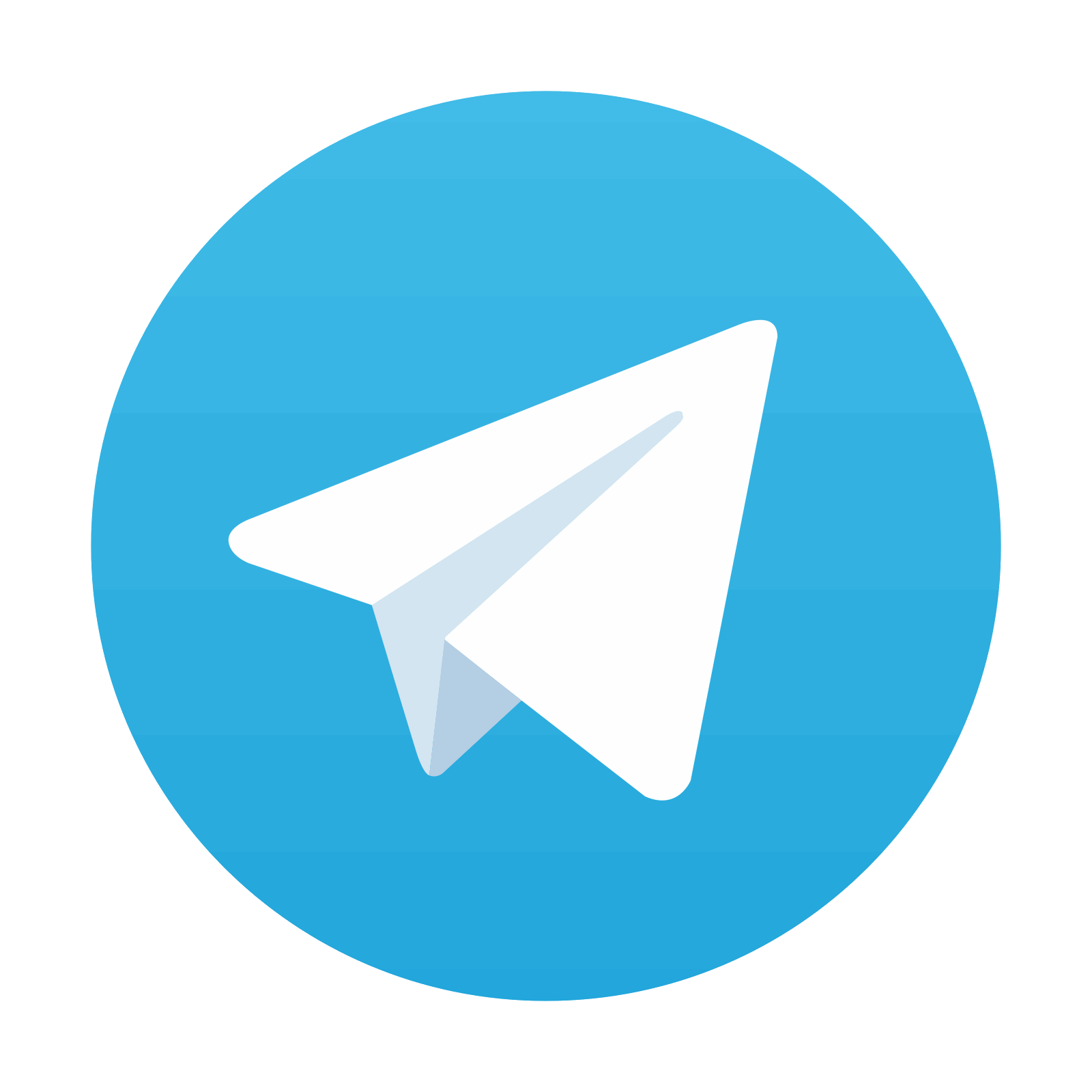
Stay updated, free articles. Join our Telegram channel

Full access? Get Clinical Tree
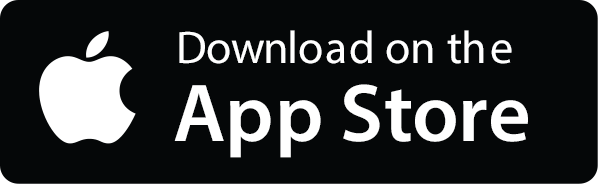
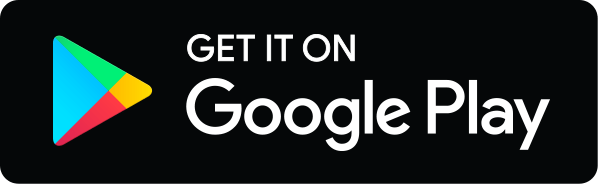