HuiChu Lin Department of Clinical Sciences, College of Veterinary Medicine, Auburn University, Auburn, AL, USA General anesthesia in farm animals, like cattle, sheep, goats, llamas, alpacas, and pigs, requires special attention due to the uniqueness of the anatomical and physiological characteristics as compared to dogs, cats, and horses. Camelids (llamas and alpacas) only have two forestomachs but are otherwise similar in many ways to cattle and small ruminants. Although some farm animals may cost as much as purebred companion animals, farm animal veterinarians are often faced with economic constraints and a limited number of approved drugs for use in surgical procedures requiring anesthesia. Physical restraint and local anesthetic techniques are most commonly employed to produce immobility and analgesia for these species. Ruminants generally tolerate physical restraint and recumbency well. This, along with local and/or regional anesthetic techniques, allows many minor surgical procedures to be performed in the standing position and under field conditions. General anesthesia is more frequently performed in camelids and swine for even minor surgical procedures due to their intolerance of physical restraint. It is important to remember that farm animals perceive pain no differently than other species, therefore analgesia for prevention and easing of pain is just as important as it is for companion animals. With surgical procedures requiring general anesthesia, balanced anesthetic technique should be employed to provide narcosis, analgesia, and muscle relaxation, thereby minimizing the stress response induced by surgery and anesthesia. Most of the anesthetics and anesthetic adjuncts commonly used in farm animal practice do not have Food and Drug Administration (FDA) approval for use in ruminants, camelids, and swine [1, 2]. However, per the Animal Medicinal Drug Use Clarification Act (AMDUCA) of 1994, extralabel use of drugs is permitted when animal health is threatened or death may result if not treated [3]. While prevention of violative residues should always be considered, anesthetics are usually used for a short duration, and anesthetized animals are unlikely to be marketed immediately after surgery. Furthermore, anesthetics used today tend to have very short half‐lives (t½), and they are potent enough that only low doses are required to produce general anesthesia. The possibility of an animal carrying anesthetic residues within its edible tissues after the surgical incision has healed, which normally occurs within an average of 14 days, is extremely low. Thus, problems with anesthetic drug residues appear to be rare [4]. Nevertheless, veterinarians should consult the Food Animal Residue Avoidance Databank (FARAD) for meat and milk withdrawal intervals for extralabel use of analgesics, sedatives, and injectable anesthetics as well as for updates of drugs prohibited from extralabel use [1, 2]. Prior to anesthesia, an appropriate patient history, including breed, age, sex, condition, and temperament of the patient, and a complete physical examination are indicated. Due to economic reasons, blood work including complete blood count and chemistry profile is performed only in farm animals with significant systemic diseases and those considered to have a higher anesthetic risk. For example, animals with severe gastrointestinal (GI) abnormalities often suffer extreme dehydration with or without electrolyte alteration, which may require intervention to optimize the patient’s condition with fluid therapy prior to the induction of anesthesia [5]. In healthy animals, total plasma protein and packed cell volume are sufficient indicators of a patient’s hydration status. Most sedatives and general anesthetics cause some degree of cardiovascular depression, which may not be a great concern for healthy patients. However, normal cardiovascular protective mechanisms or reflexes in response to the depressing effects of anesthetics may be obtunded in animals with compromised cardiac function or severe electrolyte imbalances as a consequence of disease conditions. Maintaining a balance of concentrations of electrolytes like calcium, sodium, and potassium across the cell membranes is essential in establishing normal cell membrane potential and contractility. Disturbance of these electrolyte balances across cell membranes changes cellular resting membrane potentials and subsequent initiation and propagation of cellular depolarization and repolarization. Acidosis has been shown to cause electrolyte imbalances resulting in decreased myocardial contractility and increased response of the myocardial cells to circulating catecholamines. Therefore, anesthetic‐induced cardiovascular depression combined with severe preexisting acidosis and electrolyte imbalances can lead to detrimental side effects like severe cardiac arrhythmias, bradycardia, decreased myocardial and vascular cellular contractility, reduced cardiac output, and hypotension. As a result, anesthetized animals may not be able to maintain adequate cardiac output or arterial blood pressure leading to significantly decreased peripheral tissue and muscle perfusion with subsequent development of severe adverse effects such as irreversible postanesthetic neuromyopathy [6]. Ruminants, camelids, and swine are susceptible to complications associated with anesthesia and recumbency. Positioning of these animals, particularly adult cattle, in dorsal or lateral recumbency for surgery allows for the weight of abdominal viscera to shift ventrally and cranially, causing the diaphragm to be pushed further into the thoracic cavity, thereby reducing the functional residual capacity of the lungs (Figure 1.1). As a result, an increased ventilation/perfusion mismatch may lead to significant hypoventilation and hypoxemia during anesthesia. Furthermore, the weight of the abdominal viscera may compress great vessels such as the vena cava leading to decreased venous return, cardiac output, and arterial blood pressures [7]. Therefore, close monitoring of cardiovascular and pulmonary functions and institution of appropriate treatments to ensure normal arterial blood pressure and adequate ventilation are important parts of perioperative anesthetic management. Figure 1.1 The effect of lateral recumbency on the position of the diaphragm in ruminants: A, rumen; B, diaphragm; C, lungs. Source: Illustration by Kim Crosslin. Ruminal tympany, bloat, regurgitation, and aspiration pneumonia are common problems associated with general anesthesia in farm animal species that should be anticipated and addressed with proper precautions. Ruminal fermentation continues even in anesthetized animals. Postprandial gas production at an average of 30 l per hour has been reported in cattle [8]. Normal, awake animals are able to relieve the gas produced by fermentation through the eructation. Sedatives and anesthetics tend to inhibit GI motility and prohibit eructation, thus allowing gas to accumulate in the rumen. The rumen of an adult large ruminant has a capacity of 115–150 l [9]. An average capacity of 15–18 l has been reported in small ruminants [10]. Bloating, especially in nonfasted animals, can occur during anesthesia and compromise the cardiopulmonary systems by increasing intra‐abdominal pressure resulting in compression of the diaphragm and great vessels such as the vena cava in the abdominal cavity, thus further complicating the already compromised cardiopulmonary function resulting from abnormal positioning required by the surgery. Fasting of these animals prior to anesthesia reduces the amount of gas produced by fermentation and minimizes its detrimental effect on the cardiopulmonary systems. Regurgitation and aspiration of stomach content can occur in farm animal species during anesthesia, especially in nonfasted animals. The risk of regurgitation decreases significantly when water is withheld for 6–12 hours and feed is withheld for 12–24 hours prior to anesthesia in small ruminants. Pigs are monogastrics. It has been indicated that alfalfa or any type of hay delays gastric emptying time, and vomiting with possible aspiration may occur during induction of anesthesia after a recommended fasting period of 12 hours. Thus, removal of alfalfa or other types of hay from their routine diet should be instituted 2–3 days prior to anesthesia [11]. Domestic ruminants have a large rumen that is usually full of liquid materials, and it does not empty completely even after 24–48 hours of fasting. Regurgitation can occur either during light (active regurgitation) or deep (passive regurgitation) anesthesia in ruminants and camelids in spite of preoperative fasting and withholding of water. Active regurgitation usually occurs during light anesthesia and is characterized by explosive discharge of large quantities of ruminal materials. Passive regurgitation occurs during deeper planes of anesthesia when the esophageal muscles and transluminal pressure gradients relax as a result of anesthetic‐induced muscle relaxation. If the airway is not protected, a large amount of ruminal materials can be aspirated into the trachea and reach the small airways. Aspiration of acidic stomach fluid causes immediate reflex airway closure and destruction of type II alveolar cells and pulmonary capillary lining cells. Consequently, pulmonary edema and hemorrhage, hypoxemia, and arterial hypotension develop due to loss of alveolar and capillary integrity leading to reflex airway closure, bronchospasm, dyspnea, hypoxemia, and cyanosis. Recovery from aspiration pneumonia depends on the pH and amount of ruminal materials aspirated [10]. Pigs tend to have very acidic stomach fluid with a pH as low as 1.5–2.5 [12], whereas the rumen pH remains within 5.5–6.5 in cattle, sheep, and goats [13] and 6.4–7.0 for C1 of camelids [14]. Thus, the greater impact of aspirating rumen contents lies in the amount of bacterial microflora and solid food materials aspirated. In pigs, the level of acidity of stomach fluid is the primary factor affecting the severity of damage to the pulmonary tissues upon aspiration. Severe consequences like reflex airway constriction, mechanical airway obstruction, and aspiration of bacteriologically active materials can still occur in the presence of a neutral pH in ruminants [10]. Animals may die before an endotracheal tube can be placed to protect the airway in extreme cases. Please refer to Chapter 7 for prevention and treatment of aspiration pneumonia. Preoperative withholding of feed and endotracheal intubation with an adequately inflated cuff immediately following induction of anesthesia are recommended in all anesthetized farm animals. Ruminants normally salivate profusely during anesthesia. Total amounts of saliva secretion in conscious adult cattle and sheep have been reported to be 50 l and 6–16 l per 24 hours, respectively [15, 16]. In the past, anticholinergics like atropine were used routinely as part of the anesthetic induction regimen in an effort to prevent salivation. However, atropine only reduces the water content of the saliva [17], thus causing the saliva to become more viscous and increasing the potential of airway obstruction, particularly in neonates. If the trachea is left unprotected during anesthesia, large amounts of saliva may be aspirated. Thus, tracheal intubation with appropriate inflation of the cuff immediately following induction should be instituted to protect the airway. For large ruminants, setting up the surgery table in a way that the head is lower and the throatlatch area is elevated relative to the mouth and thoracic inlet will help drainage and prevent pooling of the saliva and ruminal contents in the oral cavity (Figure 1.2). Placing a sandbag or rolled‐up towel under the neck of a small ruminant or camelid patient to elevate the throatlatch so that the mouth opening is lower than the occiput allows saliva to escape, avoiding the potential for aspiration (Figure 1.3). This technique also helps to minimize the flow of passive regurgitation during deep anesthesia [18]. Figure 1.2 Lateral recumbency of an adult bovid; note the elevation of the throatlatch. Source: Illustration by Kim Crosslin. Figure 1.3 Lateral recumbency of a small ruminant; note the elevation of the throatlatch. Malignant hyperthermia, also referred to as porcine stress syndrome, is a genetic disorder that occurs due to mutation of the ryanodine receptors (ryr‐1 locus) of the calcium channels in the skeletal muscles [19–21]. The presence of abnormal ryanodine receptors allows a massive amount of calcium to be released from the cells into the sarcoplasmic reticulum, resulting in generalized extensive skeletal muscle contraction. Though malignant hyperthermia has been reported in other animal species, pigs and humans seem to be the most susceptible. Certain breeds of pigs, like Pietrain, Poland China, or Landrace, are very susceptible to this syndrome, while Large White, Yorkshire, and Hampshire, on the other hand, are much less so [22, 23]. The clinical signs of malignant hyperthermia syndrome in susceptible pigs are manifested in a sudden and dramatic rise in body temperature and end‐tidal CO2 followed by muscle fasciculation, muscle rigidity, tachypnea, tachycardia, arrhythmias, myoglobinuria, metabolic acidosis, renal failure, and often death. The prognosis is usually poor once the episode is initiated. The triggering agents of malignant hyperthermia include stress (e.g. excitement, transportation, or preanesthetic handling), halogenated inhalation anesthetics (e.g. halothane, isoflurane, sevoflurane, and desflurane), and depolarizing neuromuscular blocking drugs (e.g. succinylcholine). Stress associated with transportation or preslaughter conditions can trigger malignant hyperthermia/porcine stress syndrome. Pigs affected by this mutation tend to develop pale, soft, and exudative meat, which can lead to significant economic loss for the procedures [24]. Lidocaine and ketamine have been indicated as triggering agents, but there is no evidence to support this theory [25]. Halogenated inhalation anesthetics are known triggers for malignant hyperthermia, and halothane has been indicated to be the most potent trigger [26]. However, a report in humans demonstrated that in a total of 75 malignant hyperthermia cases, 42 were isoflurane related, 12 were sevoflurane related, 11 were halothane related, eight were enflurane related, and only two were desflurane related [27]. Further study showed that the augmentation of caffeine‐induced contractures of frog sartorius muscle by isoflurane is three times and by enflurane is four times, whereas by halothane is 11 times [28]. Similarly, halothane appears to be the most potent and most frequently reported trigger of malignant hyperthermia in pigs. Isoflurane has been reported to trigger malignant hyperthermia in susceptible pigs like Pietrain or Pietrain‐mixed pigs [29]. Only one incidence of isoflurane‐induced malignant hyperthermia has been reported in a potbellied pig [30]. Sevoflurane‐induced malignant hyperthermia also has been reported in purebred Poland China pigs [31]. Episodes of malignant hyperthermia induced by desflurane have been reported in Large White, Pietrain, and Pietrain‐mixed pigs [29, 32]. A case of malignant hyperthermia in cattle was reported in an isoflurane‐anesthetized Angus bull [33]. At 75 minutes after induction, progressive increase of heart rate from 55 to 70 beats/minute and end‐tidal CO2 from 38 to 60 mmHg occurred and the result of arterial blood gas taken at this time showed a respiratory acidosis (pHa 7.268) with moderate increase of PaCO2 (from 29.7 to 66.4 mmHg) and a decrease in PaO2 (from 449 to 267 mmHg). Isoflurane was discontinued at this time, but heart rate (from 70 to 155 beats/minute) and end‐tidal CO2 (from 60 to 218 mmHg) continue to increase at 140 minutes post‐induction. Body temperatures recorded from nasopharyngeal probe increased from 39.5 °C (103.1 °F) at 75 minutes and 43.5 °C (110.3 °F) at 140 minutes. Unlike malignant hyperthermia in pigs [34, 35], muscle rigidity, one of the typical clinical signs of malignant hyperthermia, was not observed in this bull. In addition, clinical signs of malignant hyperthermia in pigs developed within minutes of exposure to triggering agent, whereas progression of the clinical signs in this case did not occur until 75 minutes after initiation of isoflurane. The delay onset of clinical signs has also been observed in horses [36], dogs [37], and cats [38]
1
Preanesthetic Considerations
1.1 Positioning
1.2 Ruminal Tympany
1.3 Regurgitation
1.4 Salivation
1.5 Malignant Hyperthermia
Stay updated, free articles. Join our Telegram channel

Full access? Get Clinical Tree
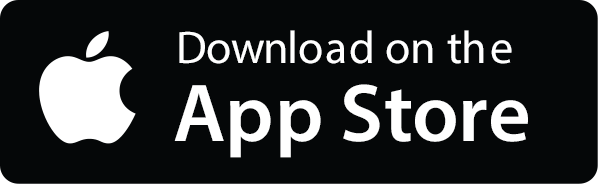
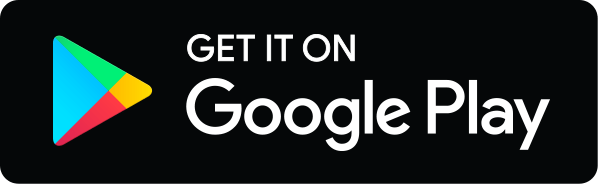