Alexander Ereskovsky1,2,3 and Andrey Lavrov2,4 1 Institut Méditerranéen de Biodiversité et d’Ecologie Marine et Continentale (IMBE), Aix Marseille University, CNRS, IRD, Avignon University, Marseille, France 2 Department of Embryology, Faculty of Biology, Saint‐Petersburg State University, Saint‐Petersburg, Russia 3 Koltzov Institute of Developmental Biology, Russian Academy of Sciences, Moscow, Russia 4 Pertsov White Sea Biological Station, Biological Faculty, Lomonosov Moscow State University, Moscow, Russia Sponges (Porifera) belong to an ancient metazoan lineage that represents one of the earliest branches of the animal tree (Simion et al. 2017). The Porifera represent one of the most diverse taxa of sessile invertebrates with over 9000 extant species. Phylum Porifera comprises classes Demospongiae, Calcarea, Homoscleromorpha, and Hexactinellida. Sponges form a monophyletic group with two clades: Demospongiae + Hexactinellida and Calcarea + Homoscleromorpha. Sponges are aquatic, mostly marine, sedentary multicellular animals, with filtration feeding and respiration. The body shape of sponges is very diverse; they may be film‐like, encrusting, lumpy or spherical, tubular, branching, flabellate, etc. The body size of sponges varies as much as their body shapes, from 3–10 mm to 1.5–2 m. Their organization is particular; they have no distinct gut, muscles, gonads, nervous system, or respiratory system; however, sponges have a complex system of canals and chambers for water pumping – the aquiferous system (Table 2.1). Age approximations of sponge species range from several months in freshwater sponges to 100 years in some marine sponges. However, research on the Caribbean giant barrel sponge Xestospongia muta suggests that this species might be capable of living more than 2000 years (McMurray et al. 2008). For sponges, both asexual and sexual reproductions are characteristic. Sexual reproduction is fundamentally no different from similar processes in other multicellular animals. Sponges can be oviparous and viviparous. In the first case, sponges are usually dioecious, while in the second, often hermaphrodites. In many viviparous sponges embryonic development is accompanied by deep destruction of aquiferous system (see section ). Asexual reproduction occurs in all poriferan clades. It may proceed by fragmentation, gemmulogenesis, and budding (for review see Fell 1974, 1993; Simpson 1984; Ereskovsky 2010). With few exceptions, sponges have a biphasic pelagobenthic life cycle with a tiny, planktonic ciliated larva that metamorphoses and grows into a large, benthic adult that is sexually reproductive (Ereskovsky 2010). Sponges are mostly filter‐feeding animals. They are the only type of Metazoa, with the exception of Placozoa, that lack phagocytoblasts in the form of intestinal epithelium. Practically all of the covering cells and many cells of the internal space participate in the capture of food particles (microbes, microalgae, organic particles, dissolved organic matter) in sponges (Hahn‐Keser and Stockem 1997). However, sponges can be “carnivorous.” These sponges feed almost exclusively on small crustaceans, which are entangled in a kind of “trapping network” formed by long thread‐like outgrowths covered by a pinacoderm with microscleres in the form of anchors on the surface (Vacelet & Boury‐Esnault 1995). Digestion, lasting for several days, is carried out both extracellularly and intracellularly in the mesohyl (Vacelet and Duport 2004). Presently sponges are gaining increased scientific attention because of their secondary metabolites and biotechnological applications. Unique and innovative structural leads have been discovered with cytotoxic, antifouling, antitumoral, antibiotic, antiviral or cytoprotective, enzyme‐inhibitory, antiinflammatory and anti‐Alzheimer activities. Sponges could also be promising, highly biocompatible biomaterial for stem cell‐based tissue engineering applications. a Alternative names for organs are provided parenthetically, in italics. The superficial region of the sponge body is devoid of choanocyte chambers (which are a component of the aquiferous system) and referred to as the ectosome (Figure 2.1a). The main component of the body, which occupies the middle part of the body wall and includes choanocyte chambers, is referred as the endosome or choanosome (Figure 2.1a). The hypophare is located in the basal part of the sponge and delimited by the endopinacoderm (an internal epithelial layer) from the endosome and by the basopinacoderm (an external epithelial layer) from the external milieu. The hypophare consists of the mesohyl (which is the mesenchyme) devoid of any elements of the aquiferous system (Figure 2.1a). The aquiferous system is a continuous water‐conducting system of variably branching tubes between the ostia and the oscules, which comprises the inhalant system, choanocyte chambers or tubes and the exhalant system. The rigidity of the sponge body is ensured by the collagen and spongin fibrils (in some Demospongiae orders) of the mesohyl and by the inorganic skeleton, consisting of either calcium carbonate (CaCO3) (Calcarea, some Demospongiae) or silica (SiO2) (Hexactinellida, Demospongiae, Homoscleromorpha). Inorganic skeleton may be represented by separate small elements (spicules), connected or fused spicules, or monolithic mineral skeleton (see section 2.4.3.1). All skeleton structures are secreted or assembled by special cells. The classes of sponges differ by the type of their organization. Sponges from classes Calcarea, Demospongiae, and Homoscleromorpha have a cellular level of organization and are combined into the nonsystematic group Cellularia. In contrast, the body of sponges from class Hexactinellida is mainly built by a voluminous network of syncytial formations. In this chapter we describe mostly the histology of Cellularia; for Hexactinellida see Leys et al. (2007). Representatives of the class Calcarea Bowerbank, 1864, the calcareous sponges (Figure 2.1b), are characterized by a calcium carbonate mineral skeleton in the form of free diactines (i.e., spicules with one axis), triactines (i.e., spicules with three rays), tetractines (i.e., spicules with four rays), and/or multiradiate spicules. This class includes approximately 770 species. A dense basal skeleton, with the main spicules cemented together, is sometimes present. The aquiferous system may have various organizational shapes, termed asconoid, solenoid, syconoid, sylleibid, or leuconoid (see section 2.4.2.1 for definitions of these shapes). Calcareous sponges are viviparous, with hollow larvae (calciblastula and amphiblastula). All Calcarea are marine sponges. The class Homoscleromorpha Bergquist, 1978 includes 120 species (Figure 2.1c). The inorganic skeleton, if present, consists of small siliceous calthrops (equiangular tetraxon with equal rays) and/or their derivatives. The aquiferous system is sylleibid or leuconoid, often with vast basal exhalant cavities. Choanocyte chambers are large. True basement membrane underlies the choanoderm (which is the epithelium lining choanocyte chambers) and the pinacoderm (which is the epithelium lining the body cavities, external surfaces, and all portions of the aquiferous system except the choanocyte chambers); pinacocytes are flagellated. All the Homoscleromorpha are viviparous with hollow cinctoblastula larvae (entirely hollow flagellated larva, with a belt of cells with intranuclear paracrystalline bodies in the region of the posterior pole). They can be both gonochoric and simultaneous hermaphrodites. All homoscleromorphs are marine sponges. Figure 2.1 Gross anatomy. (a) Scheme of sponge organization; black arrows: water currents. (b) Clathrina arnesenae (Calcarea, Calcinea) in vivo. (c) Oscarella viridis (Homoscleromorpha) in vivo. (d) Isodictya palmata (Ip) and Halichondria panicea (Hp) (Demospongiae) in vivo. (e) Oopsacas minuta (Hexactinellida) in vivo. The class Demospongiae Sollas, 1885 (about 8850 species) comprises sponges whose skeleton consists either of spongin fibers only or of spongin fibers in combination with siliceous spicules (usually, mega‐ and microscleres) (Figure 2.1d). Megascleres are larger than microscleres, and are mostly monoaxial and tetraxial. In some groups, the reduced spicular skeleton is compensated by a complex organic one (see section 2.4.3.2); in some other groups, there are no special skeletal elements at all. In several groups, a hypercalcified basal skeleton develops in addition to other skeletal elements. The aquiferous system is leuconoid. Some sponges from the order Poecilosclerida lost the aquiferous system and became carnivorous. Some species are boring sponges and live in the midst of various calcareous substrate, contributing to its bioerosion. The larvae are mostly parenchymellae or, in some groups, single‐layer larvae. Reproductive strategies within the class are oviparity and viviparity. Demosponges inhabit marine and fresh waters. Representatives of the class Hexactinellida Schmidt, 1870 (about 670 species), commonly called glass sponges, are very variable in shape (Figure 2.1e). Typically, spicules are represented by hexactins (six rays), with three axes. Spicules are divided into micro‐ and megascleres, the latter, often fused together, forming rigid skeletal lattices. Dense spongin or nonspicular skeletons are absent. Tissues of glass sponges are syncytial and consist of the dermal and atrial membranes, the internal trabecular reticulum enclosing cellular components of the sponge and flagellated chambers. Separate nucleated cells are located in syncytial pockets. Large flagellated chambers are organized according to leuconoid type. All glass sponges are viviparous, with the trichimella larva (larva with median zone of multiciliated mononucleate cells, with syncytial structures and special larval stauractin skeleton). Hexactinellida are marine, mainly deep‐sea, sponges. In general, standard protocols of tissue processing for histology are suitable for sponges. However, sponge tissues are highly sensitive to fixation procedure and subsequent manipulations. Sponges should be fixed and processed as soon as possible after collection. Contact with air should be avoided at any stage of processing, especially during manipulations with live sponges, when air could cause severe damage to fine structures in aquiferous systems. The majority of sponges possess porous tissues, highly permeable for solutions, thus requiring a shorter time for each step of the protocol. The fixation for histology should be done at 6 °C within 2–12 hours preferably in Bouin fixative or in 4% formaldehyde on sea water for marine sponges. For sponges with a mineral skeleton, its elements (spicules) should be removed after the fixation procedure by applying 5% hydrofluoric acid (for silica spicules) or 5% solution of ethylenediaminetetraacetic acid, disodium salt (EDTA) (for calcareous spicules) for two hours at room temperature. Then fixed tissues should be dehydrated through an ethanol series, placed in toluene or xylene and, finally, embedded in paraffin. Sections, 5–7 μm in thick, are mounted on glass slides and stained, with hematoxylin and/or eosin. A characteristic feature of the Porifera, distinguishing them from the other Metazoa, is a high plasticity of cellular differentiation, anatomic and tissue structures throughout the life cycle. Various differentiated cells of the sponge can move, transdifferentiate, and switch functions. The direction of the differentiation depends on the current needs of the organism. Thus, the sponge is constantly in the state of rearrangement of all its structures (Gaino and Burlando 1990; Bond 1992; Gaino et al. 1995; Maldonado and Uriz 1999; Galera et al. 2000). This “chronic morphogenesis” contributes to the growth of the animal, reconstructing of somatic tissue after degradation during sexual and asexual reproduction, and during movements of the animal (Pavans de Ceccatty 1979; Bond 1992; Gaino et al. 1995; Bonasoro et al. 2001; Lavrov and Kosevich 2018). In many Demospongiae, some stages of ontogenesis are accompanied by profound reconstructions of all the anatomic and histologic systems (Ereskovsky 2000), which can result in the destruction of all or most of the aquiferous system. These reconstructions may be caused by adaptation to adverse conditions (Simpson 1968; van de Vyver and Willenz 1975), regeneration processes (Borisenko et al. 2015; Ereskovsky et al. 2015), formation of reduction bodies (as delimited by a pinacoderm multicellular mass, consisting primarily of archaeocytes and presumably capable of reorganizing into a new functional sponge; reduction bodies result from a tissue disorganization of freshwater and estuarine demosponges) (Simpson 1984), gemmulogenesis (formation of gemmules, resistant asexual reproductive bodies) or sexual reproduction (Simpson 1984; Ereskovsky 2000; Ereskovsky et al. 2013). In general, these massive rearrangements do not interfere with the normal transport of water through the sponge and occur continuously along with the active pumping of water. Histologically, the sponge body is divided into three parts: the outer epithelial layer (exopinacoderm and basopinacoderm), the inner epithelial layers (choanoderm and endopinacoderm), and mesohyl, the inner space of the sponge body that is enclosed by the epithelial layers. The pinacoderm is represented by the exo‐, baso‐, and endopinacoderm. Exopinacoderm forms the external cover of the sponge. Basopinacoderm develops at the sponge base, attaching it to the substrate. Endopinacoderm forms the walls of the subdermal cavities and the aquiferous system canals, excluding regions of choanocyte chambers (which are lined by choanoderm; see below). There are, correspondingly, several types of pinacocytes. The exopinacoderm consists of the exopinacocytes – the covering cells of the sponge, that may be T‐shaped or spindle shaped in cross‐section (Figures 2.1a, 2.2a,b, 2.3c,d,f). The spindle‐shaped exopinacocytes are described in many Demospongiae from the orders Spongillidae and Poecilosclerida (Bagby 1970; Weissenfels 1989), in all the Homoscleromorpha (Muricy et al. 1996, 1999; Ereskovsky et al. 2014) and in some Calcarea (Borojevic 1969; Eerkes‐Medrano and Leys 2006). The T‐shaped exopinacocytes are described in part of Demospongiae and Calcarea (see section 2.4.1) (Boury‐Esnault 1973; Willenz and Hartman 1989; Ereskovsky et al. 2011; Lavrov et al. 2018). In most sponges, exopinacocytes lack specialized cell junctions, but are united with a well‐developed adhesive system (Blumbach et al. 1998; Schütze et al. 2001); for example, in Hippospongia communis, Ephydatia fluviatilis, Sycon coactum, S. ciliatum, and Leucosolenia variabilis, the sites of exopinacocyte contacts have electron‐dense thickenings of the membranes resembling zonula adhaerens (Pavans de Ceccatty et al. 1970; Pottu‐Boumendil 1975; Eerkes‐Medrano and Leys 2006; Lavrov et al. 2018). In Homoscleromorpha exopinacocytes have specialized cell junctions, and the exopinacoderm in general has all the structural features of eumetazoan epithelium (Ereskovsky and Tokina 2007). Unique characteristics of the homoscleromorph exopinacocytes are the flagellum (Muricy et al. 1996, 1999; Ereskovsky et al. 2014), and the ability to synthesize spicules (Maldonado and Riesgo 2007). Exopinacoderm contains ostia – numerous microscopic structures, 4–100 μm in diameter, through which water is drawn into the aquiferous system of the sponge. Exopinacoderm exhibits many functions characteristic of the typical eumetazoan epithelia, such as absorption, secretion, transport, excretion and protection (Harrison and de Vos 1991; Meyer et al. 2006; Leys and Hill 2012). Moreover, exopinacocytes are capable of contractile responses (Pavans de Ceccatty 1986; Wachtmann and Stockem 1992a, b; Adams et al. 2010), amoeboid movement (Ereskovsky et al. 2015), incorporation of exogenous silica particles (Bavestrello et al. 1998), and phagocytizing of food particles (Willenz and van de Vyver 1982). The basopinacoderm consists of the basopinacocytes – flattened cells, which are located at the basal surface of the sponge and function in attachment of the sponge to the substrate. Synthesizing basal spongin and fibronectin, basopinacocytes function as spongocytes (Garrone and Rozenfeld 1981; Labat‐Robert et al. 1981). During sponge growth, marginal basopinacocytes actively secrete proteins that make up spongin (Garrone 1978). In the demosponges with a massive calcareous skeleton, such as Acanthochaetetes wellsi (order Clionaida), Ceratoporella nicholsoni and Stromatospongia norae (order Agelasida), basopinacocytes participate in the formation of this skeleton (Willenz and Hartman 1989; Reitner and Gautret 1996). In calcareous sponge Petrobiona massiliana basopinacocytes participate in formation of the basal massive skeleton by producing an extracellular organic framework that might guide the assemblage of submicronic amorphous Ca‐ and Mg‐bearing grains into higher structural units (Gilis et al. 2012). Basopinacocytes of the freshwater demosponges have a well‐organized cytoskeleton (Wachtmann et al. 1990; Wachtmann and Stockem 1992a, 1992b; Kirfel and Stockem 1997; Adams et al. 2005). Actin is located in the cortical layer and in the fibrils in the cytoplasmic matrix. Microtubules radiate from the perinuclear zone, finishing at the cell periphery. At the same time, intermediate filaments have not been described. In Ephydatia muelleri, basopinacocytes were shown to have desmosome‐like junctions (Pavans de Ceccatty 1986). The endopinacoderm consists of the endopinacocytes – flattened, polygonal cells, spindle shaped on cross‐section (Figures 2.1a, 2.2b, 2.3c; see also Figure 2.7a,b,f). The endopinacocytes are divided into prosopinacocytes, lining the inhalant canals, and apopinacocytes, lining the exhalant canals of the aquiferous system. The external surface of the endopinacocytes is covered with a glycocalyx layer (Boury‐Esnault et al. 1984; Vacelet et al. 1989; Harrison and de Vos 1991). The basal surface often forms numerous projections (pseudopodia) for anchoring in the extracellular matrix. In all Homoscleromorpha and in some Demospongiae, endopinacocytes bear flagella (Boury‐Esnault et al. 1984; Vacelet et al. 1989; Ereskovsky et al. 2014). In particular, this is the case in most studied representatives of the orders Dictyoceratida and Dendroceratida (Thiney 1972; Donadey 1982; Vacelet et al. 1989; Boury‐Esnault et al. 1990). The presence of flagella appears to be associated with the involvement of endopinacocytes in the generation of water currents through the aquiferous system. However, in some demosponges, the endopinacocytes lining the oscular tube (large exhalant opening) have short nonmotile cilia, presumably with sensory function, which may be involved in the coordination of simple sponge behavior (Ludeman et al. 2014). Generally, endopinacocytes contact each other by simple overlap. However, in the oscular tubes of freshwater sponges they are united by desmosome‐like junctions (Masuda et al. 1998). Endopinacocytes of Homoscleromorpha are joined by zonula adhaerens junctions and underlined with basement membrane (Ereskovsky and Tokina 2007; Ereskovsky et al. 2009). Figure 2.2 The ectosome, dermal membrane, and cortex. (a) Semithin section of a body wall of asconoid Leucosolenia variabilis (Calcarea, Calcaronea, Leucosolenida), showing a very thin ectosome, T‐shaped exopinacocyte, mesohyl, and choanoderm. (b) Semithin section of leuconoid Oscarella tuberculata (Homoscleromorpha, Oscarellidae), showing a very thin ectosome, flat exopinacocytes, and endosome. (c) Histologic section of the ectosome of Crambe crambe (Demospongiae, Poecilosclerida). (d) Histologic section of the ectosome of Petrosia ficiformis (Demospongiae, Haplosclerida). (e) Histologic section of the ectosome of Geodia atlantica (Demospongiae, Tetractinellida) showing a cortex, reinforced with special skeletal elements – microscleres (astroscleres), and the endosome with the megascleres. Source: Image courtesy of Paco Cardenas. (f) Histologic section of the ectosome of Pleraplysilla spinifera (Demospongiae, Dictyoceratida). (g) Histologic section of the ectosome of Spongia officinalis (Demospongiae, Dictyoceratida). (h) Histologic section of upper part of Dysidea incrustans (Demospongiae, Dictyoceratida), showing the cortex with foreign material embedded into the organic skeleton. Scale bars: (a) 20 μm; (b,c,d) 100 μm; (e) 1 mm; (f,g,h) 500 μm. Figure 2.3 Cuticle, exopinacoderm, and pores. (a) Histologic section of Aplysina cavernicola (Demospongiae, Verondiida): cortex and cuticule. (b) Histologic section of Halisarca dujardinii (Demospongiae, Chondrillida): ectosome, noncellular, amorphous cuticle. (c) Histologic section of ectosome of Lubomirskia baicalensis (Demospongiae, Spongillida), showing flat exopinacocytes. (d) Semithin section of ectosome and choanosome of Oscarella lobularis (Homoscleromorpha, Oscarellidae), showing a basal membrane lining pinacoderm and choanocyte chambers. (e) Histologic section of upper part of Halisarca dujardinii (Demospongiae, Chondrillida): ectosome, choanosome, and multicellular pores. (f) Semithin section of a body wall of asconoid Leucosolenia variabilis (Calcarea, Calcaronea, Leucosolenida), showing porocytes. Scale bars: (a,c,d) 50 μm; (b) 100 μm; (e) 200 μm; (f) 20 μm. Endopinacocytes and choanocytes of some demosponges, homoscleromorphs, and calcareans are thought to be functionally and ontogenetically interrelated. For example, during reparative regeneration the choanocytes of different species from these taxa can differentiate into endopinacocytes by reduction of the flagellum and the microvilli and the subsequent flattening of the cell (Diaz 1974; Borisenko et al. 2015; Ereskovsky et al. 2015; Lavrov et al. 2018). The apopylar cell is a particular cell type forming the boundary between apopinacoderm (epithelium lining the exhalant canal) and choanoderm (see section 2.3.2.2) and linking choanocytes and apopinacocytes (de Vos et al. 1990). Apopylar cells display morphologic characteristics intermediate between those of choanocytes and pinacocytes. In Homoscleromorpha, these cells possess a flagellum and an unfolded collar of microvilli (Boury‐Esnault et al. 1984). In demosponges, the apopylar cells were observed in all investigated species of Dictyoceratida and Dendroceratida, in some Chondrosida (Halisarca dujardinii, Thymosia guernei) and Haplosclerida, in the freshwater sponge E. fluviatilis and in Tethya wilhelma (Tethyida) (Langenbruch et al. 1985; de Vos et al. 1990; Hammel and Nickel 2014). Earlier, these cells were referenced as “cone cells” and “cell‐ring” (de Vos et al. 1990). In T. wilhelma, the apopyle (the exit from the choanocyte chamber) has also a reticuloapopylocyte, a modified apopylar cell, which has numerous small intracellular pores, which give them a mesh or grid‐like morphology (Hammel and Nickel 2014). The choanoderm consists only of choanocytes, which form the choanocyte chambers (or tubes in asconoid and solenoid sponges) (see section 2.4.2) (Figure 2.2b; see also Figure 2.6a–e). Contrary to the pinacoderm, the choanoderm has a cubic or palisade epithelium. Choanocytes can be cylindrical, cubic, trapezoid, or slightly flattened. These cells bear a flagellum surrounded with a collar of cytoplasmic microvilli interconnected by glycocalyx bridges. In some demosponges choanocytes have a periflagellar sleeve, such as in Suberitidae, Polymastiidae, Acanthochaetetidae, and Halisarcidae (Connes et al. 1971; Boury‐Esnault et al. 1990, 1994; Ereskovsky et al. 2011). Another cell type associated with the choanocyte chambers of some demosponges is the central cell (Reiswig and Brown 1977; Diaz 1979; Langenbruch and Scalera‐Liaci 1986; Langenbruch and Jones 1989; Sciscioli et al. 1997; Ereskovsky et al. 2017a). Central cells have an irregular, branched shape with numerous projections and holes. The cell is perforated with a vast canal, into which flagella of the choanocytes enter. The central cells participate in the regulation of beating of the choanocyte flagella within a chamber and thus in the regulation of water currents through the aquiferous system. Diverse cells, which compose the tissue of the internal environment of sponges, are located in the mesohyl – a highly complex system occupying the internal parts of the animal’s body, between its surface and elements of the aquiferous system. Besides cells, the mesohyl includes a skeleton (both organic and mineral) (see section 2.4.3), organic ground substance, which encompasses all cellular and skeletal elements, dissolved macromolecules and often bacteria, archaea and cyanobacteria. The mesohyl has a parenchymal structure with various cell types intermixed with each other and with noncellular elements. No structural compartments can be defined in the mesohyl. Moreover, the majority of cells demonstrate apparent motility and the ability to transdifferentiate, making the structure of the mesohyl highly unstable. However, the sponge cell populations of the internal environment can be subdivided according to their functions: supportive‐connecting tissue, protective‐secretory tissue, and contractile cells, which occur in some demosponges (Ereskovsky 2010). Although these tissues are not structurally delimited from each other, they comprise groups of cells with a specific function. In addition, occasionally during various stages of sexual and asexual reproduction the gametes, embryos, and specific somatic cells participating in gamete formation (trophoblasts, nurse cells, histoblasts, thesocytes, etc.) develop in the mesohyl, significantly changing its overall structure (see section 2.4.4). This tissue comprises a variety of cells participating in the formation of organic and mineral skeleton and ground substance of the mesohyl. Collencytes (lophocytes) are mobile cells participating in the secretion of collagen and formation of its fibrils (Figure 2.4a). These cells are frequently characterized by a nucleus without nucleolus, a well‐developed rough endoplasmic reticulum (RER), few nonspecific inclusions, and specific vacuoles containing collagen, which can be dense and homogenous or contain clear fibrillar material. Collencytes are devoid of phagosomes. These cells can be found all over the mesohyl. The secretory activity of these cells is evident from the deposition of oriented collagen fibrils near the cells, sometimes attached to the cell membrane (Borojevic 1966; Bonasoro et al. 2001). Two names are used for this type of cell (Lévi 1970; Simpson 1984; Boury‐Esnault and Rützler 1997): collencyte usually refers to a stellar‐like or spindle‐like cell, while lophocyte refers to an obviously motile cell with anterior–posterior polarity and often forming a collagen bundle, which is associated with its posterior pole (Garrone 1978; Bonasoro et al. 2001). Figure 2.4 Cells of the internal environment. (a) Lophocyte of Chondrilla sp. (b) Sclerocyte of Leucosolenia variabilis. (c) Amoebocyte of Leucosolenia variabilis. (d) Archaeocyte of Crellomima imparidens. (e) Bacteriocyte of Aplysina cavernicola. (f) Myocyte of Leucosolenia sp. (g) Vacuolar cell of Oscarella tuberculata. (h) Spherulous cell of Halisarca caerulea. (i) Granular cell of Chondrilla sp. (j) Microgranular cell of Halisarca dujardinii. (k) Gray cell of Chondrilla sp. Inset – glycogen rosettes. Scale bars: (a,c,d,g,h,i,j,k) 2 μm; (b) 1 μm; (e,f) 5 μm; (inset) 0.25 μm. Spongocytes are amoeboid cells responsible for the secretion of spongin in different forms (see section 2.4.3.2). Spongocytes always form groups of several cells during spongin secretion. They are characterized by a nucleus with nucleolus, well‐developed RER, perinuclear cisterns of Golgi complex and vesicular cytoplasm, containing numerous homogenous dense inclusions with spongin precursor (Garrone 1978). Special types of spongocytes participate in the development of gemmules (a resistant asexual reproductive body, composed of internal mass of archaeocytes [thesocytes] charged with reserves and enclosed in a noncellular protective envelope) in freshwater sponges from the family Spongillidae. These spongocytes form a palisading epithelium around the developing gemmule and secrete collagenous shell and chitin for its coat (de Vos 1971, 1977; Langenbruch 1981, 1982; Ehrlich et al. 2013). Sclerocytes are mobile cells, secreting elements of the mineral skeleton – spicules. Depending on the size and hence type of spicule produced (megasclere or microsclere) (see section 2.4.3.1), the sclerocytes are divided into megasclerocytes and microsclerocytes. Megasclerocytes have a nucleus with nucleolus, prominent Golgi complex, free ribosomes, mitochondria, few phagosomes and cisterns of RER (Figure 2.4b). Microsclerocytes are characterized by smaller size and a nucleus without nucleolus (Wilkinson and Garrone 1980; Garrone et al. 1981; Custodio et al. 2002). Microscleres and microsclerocytes are characteristic only for Demospongiae and Hexactinellida. The mineral skeleton of Homoscleromorpha and Calcarea consists of megascleres of different size, so representatives of these classes have only megasclerocytes. Silica spicules of Demospongiae, Hexactinellida, and Homoscleromorpha are synthesized intracellularly in vacuoles around an organic axial filament (Uriz et al. 2003; Uriz 2006; Leys et al. 2007; Maldonado and Riesgo 2007). During synthesis of large spicules, which are bigger than sclerocytes, several cells join (Uriz et al. 2003). In Demospongiae and Hexactinellida the membrane forming the spicule vacuole has a specific structure and is called a silicalemma (Uriz et al. 2003; Uriz 2006; Leys et al. 2007). It pumps silica inside the vacuole, producing a higher concentration inside for effective deposition around the organic axial filament (Uriz 2006). No information exists about the structure of analogous membranes in Homoscleromorpha. In contrast, in calcareous sponges spicules are always synthesized extracellularly by the group (2–6 cells) of sclerocytes, which are joined by septate junctions and form an extracellular vacuole, where increased concentration of calcium ions is produced (Jones 1970; Ledger and Jones 1977; Uriz 2006). Transport cells are peculiar amoeboid cells of the mesohyl described from the freshwater sponge E. fluviatilis (Nakayama et al. 2015). Transport cells are attached to the newly synthesized megascleres and transport a spicule from its place of synthesis to the final position in skeletal framework. No specific structural features of the transport cells are yet known. This cell type is defined only by its location on the newly synthesized megascleres, motile behavior, and specific expression of the gene EflSoxB1 (Nakayama et al. 2015). Various amoeboid cells and cells with specific inclusions compose this tissue. The functions of protective‐secretory tissue include transfer and distribution of nutrition and oxygen, excretion, immune protection, and secretion of specific substances. Amoebocytes sensu lato are common motile cells of the mesohyl. There is no clear definition of these cells and at various times they were called thesocytes (Sollas 1888), spherulous cells (Topsent 1892), polyblast or hyaline cells (Tuzet and Pavans de Ceccatty 1958), amoebocytes (Müller 1911), or nucleolated amoebocytes (Wilson and Penney 1930; Faure‐Fremiet 1931; Efremova 1972). They occur in all regions of the mesohyl and often are the main cell type in it. The amoebocytes have a large nucleus with nucleolus associated with Golgi complex, numerous RER cisterns and unspecific inclusions, especially phagosomes, and symbiotic zoochlorellae in the cytoplasm of freshwater sponges (Figure 2.4c) (Gilbert and Allen 1973; Williamson 1979). Amoebocytes are traditionally considered to execute several functions including digestion and distribution of nutrition, immune response in the form of phagocytes, elimination of refractory leftovers, and functioning as stem cells. Considering the broad variety of executed functions and absence of obvious structural features, amoebocytes could represent a highly heterogenous cell group and should be further researched. Currently, one subpopulation of amoebocytes can be distinguished – archaeocytes. Archaeocytes are amoebocytes with a high nuclear/cytoplasmic ratio, with cytoplasm reach in RER and ribosomes and devoid of special cytoplasm inclusions (Figure 2.4d) (Smith and Hildemann 1990; Harrison and de Vos 1991). They occur in Demospongiae and Hexactinellida (in which they are the only cellular elements independent from the main syncytial tissues) and represent one of the stem lines in sponges of these classes (Lévi 1970; Korotkova, 1981, 1997; Simpson 1984; Harrison and de Vos 1991; Funayama, 2008, 2018). In addition, participation of some amoebocytes in various immune reactions is well known (Smith and Hildemann 1986, 1990), and some attempts to distinguish this subpopulation have been made, using molecular markers (Funayama et al. 2005). Bacteriocytes represent mobile cells with specific vacuoles, containing various symbiotic prokaryotes. This cell type is known only in demosponges. Bacteriocytes can contain single large or several small vacuoles with symbionts (Figure 2.4e) (Vacelet 1970; Vacelet and Donadey 1977; Bigliardi et al. 1993; Vacelet and Boury‐Esnault 1996; Maldonado 2007). Bacteriocytes participate in food digestion in carnivorous sponges (Vacelet and Duport 2004) and are responsible for vertical transmission of symbionts in some demosponges (Ereskovsky et al. 2005; Maldonado 2007), as they penetrate the embryos during their development and remain intact until the larval settlement and metamorphosis (Lévi and Lévi 1976). Cells with specific inclusions are an important element in sponge mesohyl. This heterogenous cell group includes various cells types, which are united by the presence of specific inclusions in the cytoplasm but differ by structure of these inclusions. Currently, the function of most cells with specific inclusions is unknown. Some evidence indicates that these cells can realize the content of their vacuoles in the mesohyl, participate in metabolism of glycogen, excrete metabolic by‐products, and produce metabolites with antibiotic functions, which may be involved in the regulation of symbiotic bacteria or defense against foreign bacteria. Cells with inclusions can be found in most demosponges and homoscleromorphs but are rare in calcareous sponges and hexactinellids. According to Simpson (1984), cells with specific inclusions are subdivided into two major categories: cells with larger inclusions and cells with smaller inclusions. Cells with larger inclusions. Vacuolar cells (cystencytes) are characterized by the presence of one or several large transparent vacuoles, occupying almost all cytoplasm of these cells (Figure 2.4g; see also Figure 2.7b,f). The free cytoplasm is reduced to a thin film around inclusions and nucleus. The nucleus may be displaced to the cell periphery by the inclusions. In addition, cytoplasm may contain Golgi complex, some RER and smooth endoplasmic reticulum, few mitochondria, and small phagosomes. Cystencytes are vacuolar cells of freshwater sponges, having one large inclusion with amorphous material of a polysaccharide nature (Tessenow 1969; Pottu‐Boumendil 1975; Ereskovsky et al. 2016). Vacuolar cells can be used as a diagnostic characteristic in closely related species of sponges without a skeleton, for example Oscarella and Halisarca (Muricy et al. 1996; Ereskovsky 2006, 2007). Spherulous cells have several large membrane‐bounded inclusions (0.8–8 μm diameter). Free cytoplasm is reduced to narrow strands between the inclusions and on the cell periphery. The cytoplasm contains few mitochondria and rare cisterns of RER (Figure 2.4h; see also Figure 2.8f). The nucleus is usually small, anucleolated, and deformed by the inclusions. Inclusion content is usually homogenous but can be paracrystalline, fibrillar, or lamellar (Diaz 1979; Thompson et al. 1983; Bonasoro et al. 2001; Ereskovsky et al. 2017b). In some species, spherulous cells are localized near the sponge surface or aquiferous system canals, although they can lie diffusely in the mesohyl (Uriz et al. 1996; Bonasoro et al. 2001; Ereskovsky 2007; Maldonado 2016). The presumable function of the spherulous cells varies in different species, indicating possible heterogeneity of this cell type. The spherulous cells were reported to participate in storage of various metabolites (including toxic ones) (Thompson et al. 1983; Uriz et al. 1996; Becerro et al. 1997), immune response against nonsymbiotic bacteria, defense against fouling, predation by release of metabolites (Thompson et al. 1983; Ternon et al. 2016), excretion (Vacelet 1967; Donadey 1978; Maldonado 2016; Ereskovsky et al. 2020), and mesohyl extracellular matrix synthesis and maintenance (Donadey and Vacelet 1977; Donadey 1982; Bretting et al. 1983; Smith and Hildemann 1990). Granular cells have numerous membrane‐bound inclusions (0.5–2 μm diameter) in their cytoplasm. The inclusions of the granular cells are smaller, and their number is higher in comparison with spherulous cells (Figure 2.4i). The shape of inclusions varies from round to irregularly ovoid. Using electron microscopy, the inclusions are usually homogenous, but they also can be fine‐grained or have a fine‐grained periphery with a homogenous central region. The content of the inclusions is often separated from the surrounding membrane by the transparent space. The nucleus is round with or without a nucleolus. A few phagosomes, unspecific inclusions and vacuoles can appear in the cell cytoplasm (Pomponi 1976; Ereskovsky et al. 2011, 2017a, 2017b; Willenz et al. 2016). The exact functions of granular cells are unknown, but they may be involved in the immune response, as the inclusion contents show antimicrobial activity (Krylova et al. 2003). In addition, in some demosponges maternal granular cells penetrate into the forming larvae (Ereskovsky and Gonobobleva 2000; Rützler et al. 2003) and can be retained there until the beginning of metamorphosis (Gonobobleva and Ereskovsky 2004). Granular and spherulous cells can be used as a diagnostic characteristic in closely related species (Pomponi 1976; Boury‐Esnault et al. 1994; Bergquist 1996; Muricy et al. 1996; Reveillaud et al. 2012; Gazave et al. 2013; Willenz et al. 2016). Microgranular cells are characterized by cytoplasm filled with minute dense granules (~0.09–0.3 μm diameter). The nucleus is often anucleolated and cytoplasm contains few mitochondria and RER cisterns (Figure 2.4j) (Sciscioli et al. 2000; Pinheiro et al. 2004). These cells could contribute to the synthesis of glycoprotein components of the extracellular matrix. Others functions of the microgranular cells remain unknown. Cells with smaller inclusions. Gray cells (glycocytes) contain numerous small ovoid membrane‐bounded inclusions (~0.2–0.8 μm diameter). The inclusions are acidophilic and osmiophilic. Another characteristic feature of these cells is glycogen rosettes in the cytoplasm (Figure 2.4k). Besides the glycogen rosettes, the cytoplasm of gray cells contains well‐developed RER and Golgi complex (Boury‐Esnault 1977). The nucleus usually contains a small nucleolus. Presumably these cells participate in glycogen metabolism (Boury‐Esnault 1977) and also have been considered as immunocytes, responsible for the allogeneic response (Humphreys 1994; Yin and Humphreys 1996; Sabella et al. 2007). Rare type of cells with inclusions. The types of cells with inclusions described above are widespread and found in many sponge species. In addition to these, several rarer types of cells with inclusions occur in some sponges: rhabdiferous cells (Simpson 1968; Smith 1968; Smith and Lauritis 1969; Ereskovsky et al. 2011), sacculiferous cells (Smith 1968; Smith and Lauritis 1969), spumeuse cells (Donadey and Vacelet 1977; Donadey 1982), globoferous cells (Borojevic and Lévi 1964; Simpson 1968) and styllocytes (Harrison et al. 1974). These rare cell types have been found in one or several sponge species, consequently additional studies of their structure and functions are required. Moreover, these rare cells with inclusions could possibly be species‐specific modifications of common types of cells with inclusions. Contractile cells of the mesohyl, myocytes, are found in the mesohyl of many demosponges and calcareous sponges. In some sponges, the myocytes can occur in large concentric multilayered structures (sphincters) around large exhalant canals and oscula, while in other species they lie sparsely in the mesohyl near the aquiferous system canals and/or dermal membrane (Bagby 1966). The myocytes are fusiform cells with an ovoid nucleus, lying in the central part of the cell (Figure 2.4f). Other organelles (Golgi complex, mitochondria, unspecific inclusions) are usually located near the poles of the nucleus. The bundles of myofilaments are concentrated at the cell periphery. In some sponges, the myocytes have two types of filaments, which are spatially organized, forming regular patterns (e.g., Tedania ignis) (Bagby 1966; Thiney 1972). Considering their position and ultrastructural features, the myocytes are thought to be contractile cells, which regulate the diameter of large canals of the aquiferous system, thus participating in the regulation of water flow. The mesohyl occupies the internal spaces of the sponge body and is delimited by the pinacoderm and choanoderm. The degree of mesohyl development varies greatly according to the type of sponge body organization: in asconoid sponges the mesohyl has a thickness of only dozens of micrometers (see Figure 2.6a), while in leuconoid sponges it comprises the main volume of a sponge (see Figure 2.6e). The mesohyl is a complex compartment, comprising numerous cells of different types, organic and inorganic skeletal components, collagen fibers, unstructured extracellular ground substance, and symbiotic organisms. It does not have a permanent structure or structural units, appearing as a highly dynamic and variable system, although the mesohyl of the specialized parts of the sponge body (e.g., cortex, dermal membrane, etc.) can have permanent structural features like extracellular matrix arrangement and/or cell type composition (see section 2.4.1). All mesohyl cells are in constant movement (Bond 1992; Gaino et al. 1995). However, semipermanent structures can appear in the mesohyl. Mesohyl cells have a tendency to form small transient groups of 2–10 cells. Sometimes such groups are united in a single accumulation of cells, moving in the same direction, so‐called cell tracts. Cell tracts can be formed in various regions of the sponge mesohyl, but usually are characteristic for regions of growth and leading edge of moving sponge (Bond and Harris 1988; Bond 1992). The only known permanent structures in sponge mesohyl are peculiar cellular strands, described in the genus Aplysina (Leys and Reiswig 1998). These strands run through the endosome of the sponge and are composed of elongate cells tightly aligned along bundles of collagen (Figure 2.5). The cells have a permanent position in the strands and do not actively move. According to experiments, the strands are involved in nutrition transport and thus may represent a primitive nutrient transport pathway (Leys and Reiswig 1998). The mesohyl always comprises well‐developed extracellular matrix. A common fibrillar component in mesohyl of all sponges is collagen. The collagen fibrils may be dispersed through the mesohyl or form bundles and tracts. In some species collagen fibrils are the only skeletal elements and are greatly elaborated (see section 2.4.3.2). The mesohyl ground substance is rich in glycoproteins, but also contains fibronectin, various glycosaminoglycans, mucopolysaccharides, proteoglycans, sugars (including the unusual arabinose), amino acids, etc. (Gross et al. 1956; Katzman et al. 1970; Evans 1975; Junqua et al. 1975; Garrone 1978). The composition of ground substance varies by species and even from individual to individual within a single species. It appears likely that a considerable proportion of the components of the mesohyl ground substance is synthesized and released by various cells with specific inclusions (see section 2.3.3.2). The mesohyl ground substance and collagen fibrils represent a basic scaffold for mesohyl cells and may play a crucial role in cell–cell or cell–matrix interactions, immune reaction, self/nonself recognition, cytodifferentiation, cell aggregation (e.g., during formation of gemmules), and possibly other physiologic processes. Figure 2.5 Mesohyl cellular strands of Aplysina cavernicola. (a) General view of endosome with several cellular strands. (b) Structure of cellular strands. Scale bars: (a) 500 μm; (b) 100 μm. The mesohyl varies in cell type and composition, both between and within species. For instance, the mesohyl of calcareous sponges contains few cells, most of which are sclerocytes, while amebocytes and cells with specific inclusions are rare (Eerkes‐Medrano and Leys 2006; Lavrov et al. 2018). In homoscleromorphs, the mesohyl contains numerous cells with inclusions, especially vacuolar cells, which can be represented by several types (see Figure 2.7b) (Gazave et al. 2013). Both calcareous and homoscleromorph sponges could lack archaeocytes in their mesohyl. Demosponges usually have highly cellular mesohyl amoebocytes, archaeocytes, skeleton‐secreting cells and several types of cells with inclusions, which vary from species to species (Simpson 1984). The intraspecies variations in mesohyl cell composition occur due to different physiologic states of sponge tissues, mainly during the reproduction and life cycles (see section 2.4.4.3). All sponges are associated with microbial communities, with representatives of 41 different prokaryotic phyla (Thomas et al. 2016; Moitinho‐Silva et al. 2017), which are located in the mesohyl, extracellularly, in the ground substance, or in the special cells, bacteriocytes (Lee et al. 2001). Sponge species were observed to harbor dense communities of symbiotic microorganisms in their tissues, while others were almost devoid of microorganisms. The former were termed “high microbial abundance” (HMA) and the latter “low microbial abundance” (LMA) sponges (Hentschel 2003). In HMA sponges, microbial biomass can comprise up to one‐third of the total biomass (Vacelet 1975), and bacterial densities are 2–4 orders of magnitude higher than in LMA sponges. Moreover, HMA microbiomes are highly complex, while LMA microbiomes are mainly represented by Proteobacteria and Cyanobacteria (Moitinho‐Silva et al. 2017). The sponge‐associated microorganisms participate in nutrient cycling, vitamin and secondary metabolism, and chemical defense (Taylor et al. 2007; Webster and Taylor 2012).
2
Porifera
2.1 Introduction
Organ system
Organs
Body wall – ectosome
Glycocalyx, cuticle, exopinacoderm, dermal membrane, cortex
Digestive
No special system
Alimentary canal
No special organs; aquiferous canals perform these functions
Digestive organs
No special organs; choanocyte chambers perform these functions
Excretory
No special organs or structures; these functions are realized at the cellular level
Circulatory
No special system; aquiferous system perform these functions
Aquiferous system
Ostia, subdermal (vestibular) cavities, inhalant canals, prosodus, choanocyte chambers/tubes, aphodus, exhalant canals, atrium, oscula
Immune
No special organs or structures; functions are realized at the cellular level
Respiratory
No special organs or structures; functions are realized at the cellular level
Nervous
No special system; some functions are realized at the cellular level
Reproductive
Only temporary structures
Male
Temporary spermatocysts
Female
Temporary incubate chambers and follicles
Special senses/organs
No special system or organs; this function is realized at the cellular level
2.2 Gross Anatomy
2.2.1 Keys for Dissection/Processing for Histology
2.3 Histology
2.3.1 Particularity of Sponge Tissues
2.3.2 Bordering Tissues – Epithelia
2.3.2.1 Pinacoderm
2.3.2.2 Choanoderm
2.3.3 Tissues of the Internal Environment
2.3.3.1 Supportive‐Connective Tissue
2.3.3.2 Protective‐Secretory Tissue
2.3.4 Loose Connective Tissues (Mesohyl)
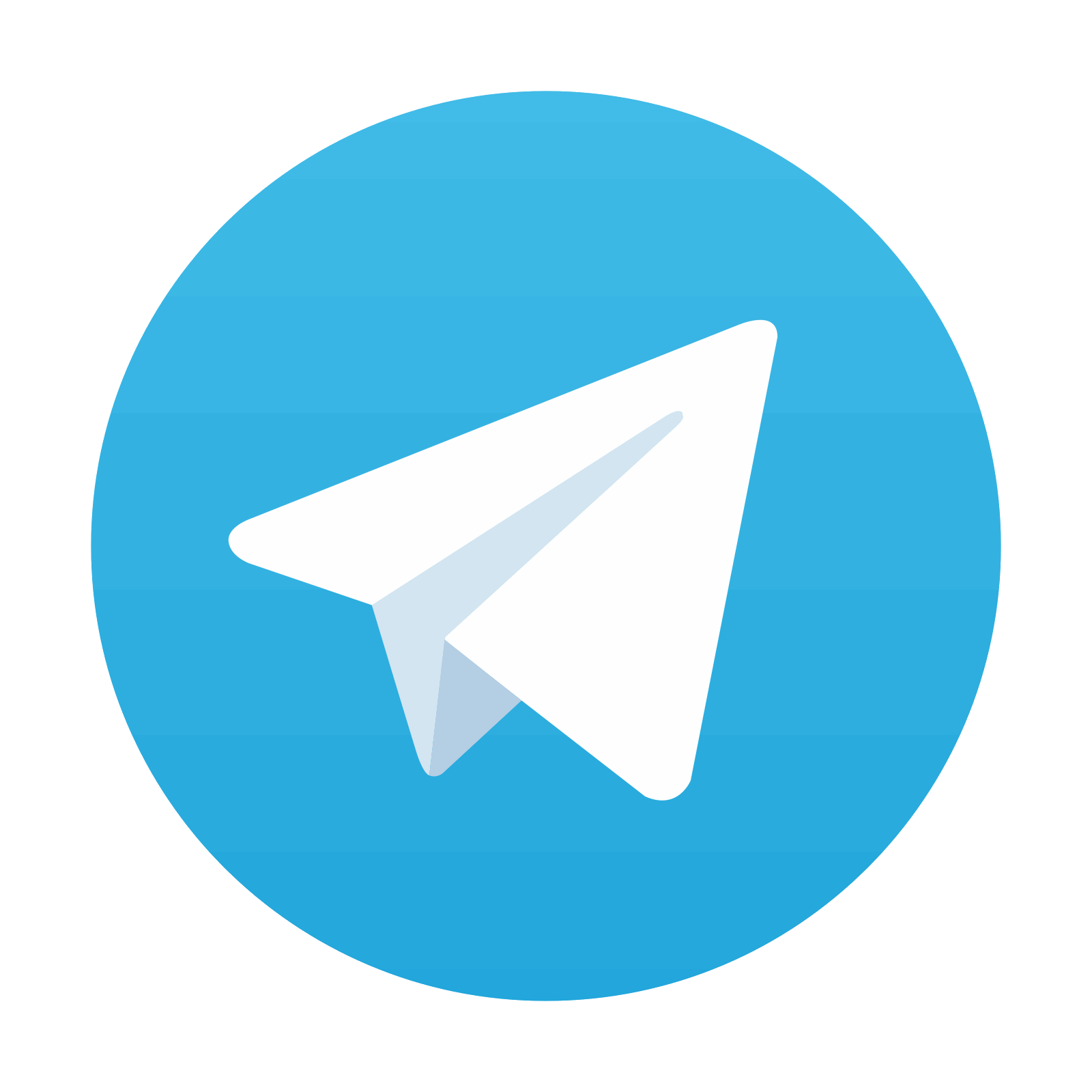
Stay updated, free articles. Join our Telegram channel

Full access? Get Clinical Tree
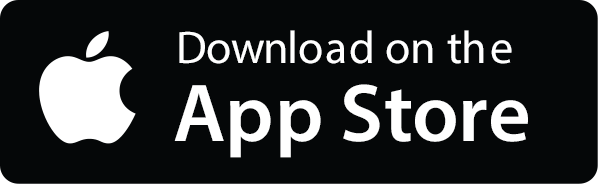
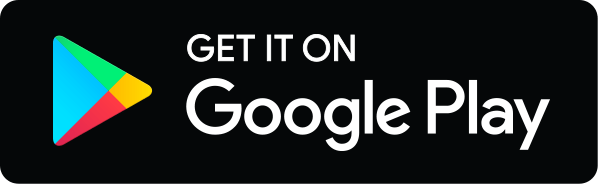