9 Porcine Rubulavirus (PoRV-LPMV) Swedish University of Agricultural Sciences, Uppsala, Sweden In the early 1980s, a seemingly new disease of pigs appeared in the state of Michoacán, Mexico. This new disease was characterized by respiratory signs, encephalomyelitis and corneal opacity in young pigs (Stephano et al., 1988). It was often fatal in the early days in very young pigs, while older pigs were considerably more resistant. In older pigs, reproductive disorders were typically associated with the disease. A few years after the initial outbreak of the disease, Moreno-López et al. (1986) isolated a paramyxovirus. Later on, in a series of molecular studies, it was shown that the causative agent was a virus (Fig. 9.1), now classified into the rubulavirus genus, in the paramyxoviridae family. Initially, as the classification system was different at that time, this new virus was found to be a paramyxovirus, closely related to mumps virus and simian virus 5 (Berg et al., 1991, 1992, 1997; Sundqvist et al., 1992; Svenda et al., 1997). The virus has been called La Piedad Michoacán virus (LPMV). The current recognized name is porcine rubulavirus (PoRV), sometimes with the name of the isolate such as LPMV at the end (PoRV-LPMV), as a reminder of the initial name of the virus La Piedad Michoacán virus or the town it was isolated from. In the literature, different isolates have different names, which may be confusing. But there are mainly two reference strains used in most studies, the abovementioned LPMV from 1984 and PAC-3 from 1992. The name PAC is from the Spanish abbreviation of Department of Porcine Animal Production (Producción Animal Cerdos). In addition, some isolates are called C-I to C-IV (it is unclear what this abbreviation stands for). As has been described elsewhere, and will be described more in a later section, the typical clinical signs largely depend on the age of the pigs and thus vary a lot. Later experimental studies confirmed field observations (Allan et al., 1996) showing a severe pattern in young pigs. There are some indications that the pathogenicity has changed over the years and PAC-3, for example, does not show the same severe neurological signs as LPMV from 1984 did in young pigs (Ramírez-Mendoza et al., 1997; Stephano, 2002; Sánchez-Betancourt et al., 2008). The evolution of PoRV has provided a number of subgroups, which are suggested to be three based on the HN gene (Sánchez-Betancourt et al., 2008). For the other genes the sequence information is scarce and therefore any general conclusion on viral evolution over time cannot be made. However, observation of the phylogenetic tree in the paper by Sánchez-Betancourt (2008) suggests that there may be more subtypes/genotypes. For example, LPMV and PAC4 make up one, PAC6-9 one, PAC2-3 one, CI-III one and finally C-IV another one. Fig. 9.1. Electron microscope picture of PoRV-LPMV (from Moreno-López et al., 1986), typically showing a damaged virion with the herring-bone nucleocapsid leaking out. The origin of PoRV has been discussed since its first appearance in pigs. Fruit bats are known reservoirs of viruses, including several recently appearing paramyxoviruses such as Nipah virus, Hendra virus, Menangle virus, Tioman virus and Mapuera virus, so fruit-eating bats have been suspected to be the host of PoRV. In fact, Mapuera virus is rather closely related to PoRV both on a sequence level and in genomic organization (Wang et al., 2007). This virus was isolated from a fruit bat in Brazil in 1979, when PoRV first appeared. They are however quite divergent in sequence and too far away geographically to be a direct transmission. But it clearly shows that related viruses were in circulation in the Americas at the time. Strong evidence for fruit-eating bats being the reservoirs of PoRV was provided by Salas-Rojas et al. (2004). They showed that one sample from several collected fruit bats from different species in Mexico was seropositive for PoRV. Even if it was only one out of many, this indicated fruit bats in the region can carry PoRV. It is not clear if this finding is important for the epidemiology of the virus, but it indicates that the virus originally came from fruit bats, even if other reservoirs cannot be ruled out. It is also possible that the virus has been present in pigs in Mexico for a long time, but changed its pathogenicity in the early 1980s. The most likely reservoir of the virus at this day and from the original introduction is likely persistently infected pigs or pigs with milder clinical symptoms. Although older pigs are not very susceptible in terms of disease signs, this does not mean that they are resistant to infection. On the contrary, several studies suggest that older pigs are susceptible to infection and can therefore maintain the virus in the pig population. Furthermore, serological surveillance studies indicate that the virus is widespread in the pig population (Escobar-López et al., 2011). Taken together, the virus is circulating freely in the pig population and a wild reservoir has minor importance for the maintenance of PoRV in Mexico. The situation in Mexico is still problematic and several possible serotypes are co-circulating. A recent study by Escobar-López et al. (2011) showed that the overall sero-prevalence is quite high and differs between the states. For example, in the state of Guanajuato, the sero-prevalence has been as high as 29.7% (Morilla et al., 2002), which was confirmed by Escobar-López et al. (2011). There is also a typical pattern where different isolates predominate in different states, while some co-circulate in others (Escobar-López et al., 2011). Some studies suggest that there are at present subgroups that share serological and genetic similarities. The disease is endemic in Mexico, but has not been found or described elsewhere. In Mexico, the presence of the virus/disease is well correlated with the density of pig production, since states with large production also have more problems with the disease (Escobar-López et al., 2011). In fact, PoRV is considered to be one of the most severe diseases affecting the pig industry. As noted before, the clinical signs are variable and depend on the age of the pig. Piglets 2–15 days old are most susceptible, and the clinical signs are quite sudden in onset. The main characteristics of the disease are encephalomyelitis, pneumonia and corneal opacity. The disease involves infection of the brain and other parts of the central nervous system, producing nervous signs: ataxia, weakness, rigidity in the hind legs, muscle tremor, involuntary movements etc. After the appearance of the first signs, piglets usually die within 48 hours (Stephano et al., 1988). It has been suggested that the virus is responsible for an increased number of stillbirths and mummified foetuses (Stephano and Gay, 1984). Some boars develop orchitis, epididymitis and later on unilateral or bilateral testicle atrophy. The semen quantity and quality is affected. Abscess on the epididymis head histologically corresponding to spermatic granulomas was also observed. The patho-biology and clinical signs of the infection were reviewed by Stephano et al. (1988; Stephano, 1999). The main disease signs are also reproducible experimentally in piglets, pregnant gilts and boars. The outcome of experimental infection in animals at different age is described below: The clinical outcome of experimental infection in piglets at the age of 3 or 17 days has been described by Hernández-Jáuregui et al. (2004). The piglets killed sequentially at different days post-infection showed clinical pathological signs of the central nerve system (CNS) (ataxia, muscle tremor), increased respiratory rate, some of them developed corneal opacity, and several pigs died. The clinical sigs in the older pigs were less severe than in the younger animal. Sequential post mortem examination revealed gross and microscopic lung and brain lesions. Neural lesions commenced in the olfactory pathway, and subsequently occurred in other fore mid- and hind-brain regions. Brain lesions were associated with PoRV antigens in neurons and macrophages; viral antigens were demonstrated in pneumocytes and intra-septal and intra-alveolar macrophages in pigs that had gross lung lesions. These results indicate that 3-day-old pigs are more susceptible to PoRV infection than 17-day-old pigs and that, following intranasal and intra-conjuntival inoculation, PoRV can gain anterograde access to the brain via the olfactory pathway. This finding may explain the rapid onset of nervous signs in young pigs during spontaneous outbreaks of PoRV infection. The histopathological lesions and viral distribution in different tissues in most of the experimental infected piglets were in agreement with observations on age-dependent susceptibility in naturally infected piglets (Stephano et al., 1988; Allan et al., 1996; Kennedy et al., 2002). In experimentally infected animals both viral proteins and viral nucleic acid could be detected. Using a polymerase chain reaction (PCR) technique the viral P gene was detected in different organs including the brain (Berg et al., 1992). The gilts at 6 or 10 weeks of gestation were experimentally infected and killed at 8 or 15 weeks of gestation or after natural parturition (Hernández-Jáuregui et al., 2004). Oro-nasal exposure of pregnant gilts to PoRV resulted in virus-induced reproductive failure. The gilts show focal congestion and haemorrhages in the placenta and endometrium. Abnormal pregnancies were found on gilts and PoRV was isolated from lungs, tonsils, ovaries, placenta, uterus and lymph nodes. The allantoic fluid from one gilt, at 9.5 weeks of gestation and 3.5 weeks after inoculation, was positive for virus isolation. Many foetuses were smaller than normal and had dermal ecchymoses. Dead foetuses, brown in colour and with autolysis, were frequently found and were dehydrated or mummified. Early signs of foetal damage were diffuse haemorrhages on the skin and diminished size, compared with no affected foetuses in the same or parallel gravid horn. Older foetuses that died in uteri were also found to be dehydrated and with advance autolysis (mummies). Virus isolation from brain and lung tissues was possible from several foetuses. These observations supported previous clinical studies indicating susceptibility of pregnant swine after natural outbreaks of PoRV. The anatomy of the porcine placenta would seem to preclude direct transfer of virus between maternal and foetal circulation. In the absence of immunity, gilts and sows may be susceptible to viral infection, resulting in stillbirths, mummies and abortions. It appears that the placenta and endometrium are not selective sites of virus replication and cell damage. These observations supported previous clinical studies indicating susceptibility of pregnant swine after natural outbreaks of PoRV. The results indicated that after experimental infection, PoRV can replicate in tissues of seronegative pregnant gilts, cross the placenta, and cause foetal death and mummification. In experimentally infected animals both viral proteins and viral nucleic acid could be detected. Using a polymerase chain reaction (PCR) technique the viral P gene was detected in different organs, including the brain (Berg et al., 1992). Boars infected with PoRV at 9 months of age were killed sequentially on different days post inoculation (Ramírez-Mendoza et al., 1997; Moreno-Lopez and Hernández-Jáuregui 2002; Hernández-Jáuregui, 2003). In general, post-mortem examinations of the reproductive tract showed swelling, severe fibrosis and nodules in the head of the epididymis and some testes were atrophic with degeneration of seminiferous tubules and interstitial mononuclear cell infiltration. Histopathological alterations included formation of spermatic granulomas and vacuolar degeneration of ductular epithelium. In immunofluorescence and immunohistochemistry assays using a monoclonal antibody against the NP protein, PoRV antigens were also found in the head of the epididymis. The results indicate that porcine rubulavirus can cause severe epididymitis, orchitis and reduced semen quality in sexually mature boars. The porcine rubulavirus is closely related to human mumps virus, sharing 41–47% protein sequences homologies (Sundqvist et al., 1990, 1992; Berg et al., 1991, 1992). Mumps virus infection in man causes epididymitis, orchitis, parotitis, pancreatitis and meningoencephalitis (Gnann, 1992), and similar organ tropism (brain, testicles, epididymis) is a characteristic feature of PoRV infection. Based on these results and the close genetic relationship to mumps virus in humans, it is of particular interest to correlate the pathological lesions observed in PoRV-infected boars with those of mumps virus infection in humans. In both cases the infection is characterized by epididymitis, orchitis and meningoencephalitis (Gnann, 1992; Ramírez-Mendoza et al., 1997). In mumps virus and PoRV infections, the epididymitis may be of a short duration, followed by a relatively rapid restoration of affected tissues. In view of the genomic relatedness of porcine rubulavirus to mumps virus, it is not surprising that both viruses have tropisms for a similar range of tissues. In parotitis, epididymo-orchitis occurs in approximately 25–30% of post-pubertal men with mumps infection and pancreatitis is also present (Gnann, 1992). Based on these results, it is concluded that the lesions in the epididymis and testicles of PoRV-infected boars may resemble those of mumps virus infection in humans. Considering the similarities of the PoRV lesions in the epididymis and the lack of more relevant information of the lesions in the human with mumps infection, PoRV infection in sexually mature boars may therefore be a useful model for mumps virus infection in the reproductive tract of post-pubertal human males. The observation that PoRV appeared to have changed its pathogenicity is intriguing. The viral genetic background of its molecular mechanism is still unclear but would be interesting to study in more detail. The initial pathogenicity was considered to be very high in young pigs with very unpleasant disease signs. The HN protein may be a key factor of pathogenicity, as suggested by Sánchez-Betancourt et al. (2008). They performed a thorough analysis of selected viruses with different pathogenicity from low neurovirulence to more severe form of the diseases, and concluded that this difference is associated with HN gene sequences. They observed that the PAC 6–9 group, being closely related genetically, also were more neurovirulent. This led to the conclusion that the HN gene might be responsible for the increased neurovirulence (Sánchez-Betancourt et al., 2008). But, as they pointed out, there are likely to be more factors of relevance for the differences in pathogenicity. It might also be a multi-genic property, such that the HN gene and some immune evasion mechanisms, mediated from other viral genes, cooperate to give PoRV its highly pathogenic properties. There are very few other known attempts to figure out the viral genetic background behind the pathogenicity determinants for PoRV. But the virus has been characterized in some detail molecularly and in cell culture system. A number of experimental infection studies have been performed, but no comparative studies in the sense that a known genetic factor can be linked to the high neurovirulence of PoRV have been conducted. The whole genome of the virus has been determined for one isolate from 1984 LPMV (Berg et al., 1991, 1992, 1997; Sundqvist et al., 1992; Svenda et al., 1997; Wang et al., 2007). In addition, the HN (Sánchez-Betancourt et al., 2008) and F genes (Berg et al., 1997) have been sequenced from a number of isolates. But in total, we know very little on the genetic variation over time of this virus, and further studies would be valuable. The genome follows the standard organization of viruses in the mononegavirales, assuming that the functions of the genes are similar to other related viruses. The full-length genome sequence was determined in 2007, including the gene-ends, and was assembled with the sequence data from earlier reported genes (Wang et al., 2007). The order of the genes from the 3′-end is NP-P/V/C-M-F-HN-L (Fig. 9.2). However, one peculiar notification that LPMV shares with the related Mapuera virus of fruit bats was that the P-gene has an open reading frame of a putative C-protein (Berg et al., 1992; Wang et al., 2007). This protein has an internal AUG start codon in a different reading frame from P/V. Similar between these viruses is also that the P-mRNA needs to be edited to ‘open-up’ the C-terminal part of the P protein at its editing site (Berg et al., 1992). The unedited mRNA expresses the V protein. A third product, sometimes called I, may also be expressed via insertion of one G at the editing site. This putative protein has an early stop codon and has only six amino acids from the editing site (Berg et al., 1992). No studies have been conducted to address the functional relevance of the editing and differential expression of the P, V and I proteins. Unpublished data indicated that during persistent infection in cell culture there was significantly more expression of V than P protein. The data have however not been verified in persistently infected animals or in any other in vivo situation. If this is true during infection, this may well be related to the known function of the V protein of related viruses of inhibiting type I interferon (Versteeg and García-Sastre, 2010). It is not know whether the V protein of PoRV has the ability to block the IFN system, but ongoing work in several laboratories may soon answer this question.
9.1 Historical Aspects and General Background
9.2 Origin and Maintenance of the PoRV
9.3 Current Situation
9.4 Clinical Picture and Pathogenesis
9.4.1 Experimental infection in piglets
9.4.2 Experimental infection in pregnant gilts
9.4.3 Experimental infection in boars
9.5 Molecular Biology
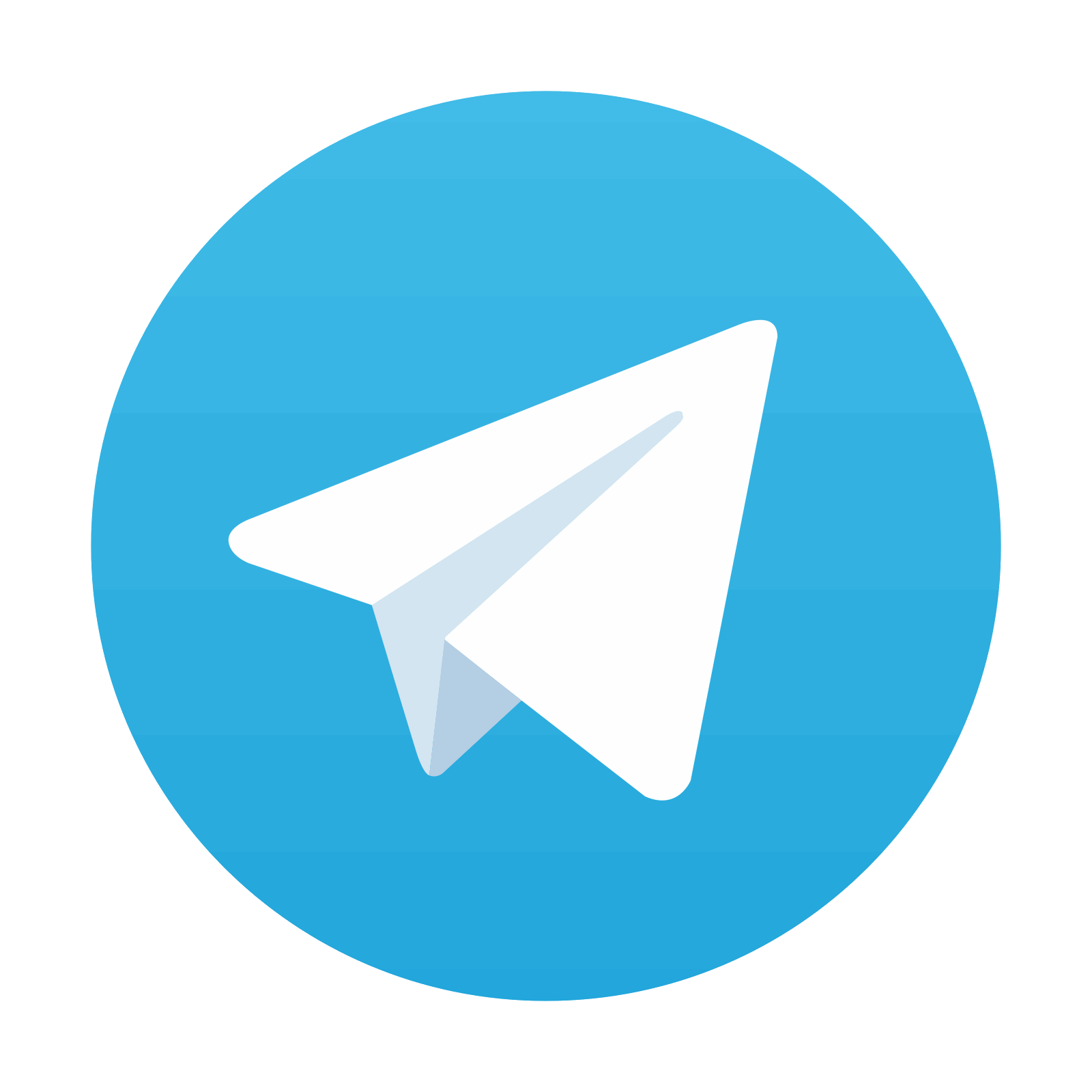
Stay updated, free articles. Join our Telegram channel

Full access? Get Clinical Tree
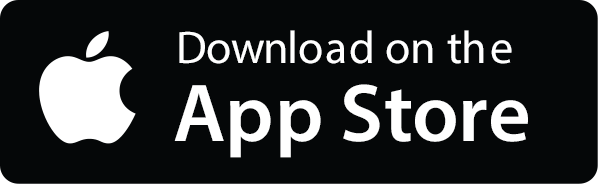
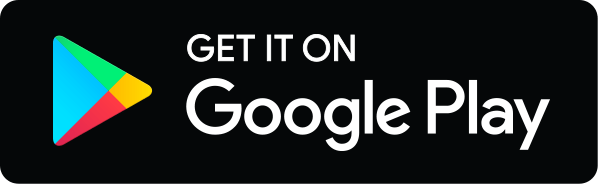