Søren R. Boysen1 and Daniel S.J. Pang2 1 Faculty of Veterinary Medicine, University of Calgary, Calgary, Alberta, Canada 2 Faculty of Veterinary Medicine, University of Calgary, Calgary, Alberta, Canada and Faculty of Veterinary Medicine, Université de Montréal, Saint-Hyacinthe, Québec, Canada In 2004, publication of the abdominal‐focused assessment with sonography for trauma (FAST) exam in dogs suffering motor vehicular injury [1] was the first work in small animals to become more broadly known as point‐of‐care ultrasound (POCUS). Although the terms POCUS and FAST are often used interchangeably, they are not synonymous. FAST exams in both human and veterinary medicine were developed to, and still primarily focus on, the search for free fluid in body cavities and pneumothorax [1–6]. By contrast, POCUS is broader in scope; it incorporates all clinically driven real‐time assessments that can be answered within minutes using ultrasonography [4,5]. More specifically, POCUS is defined as the acquisition, interpretation, and immediate clinical integration of ultrasonographic imaging performed patient‐side by an attending clinician, not by a radiologist or cardiologist, with the goal of answering a focused question or series of questions rather than assessing all structures of an organ(s) [5]. To this end, it is best applied as a problem‐based assessment based on Bayes’ theorem of pretest probabilities, which enables the clinician to gather key pieces of information in real time to help narrow or determine a diagnosis, streamline care, guide ongoing management, and reduce cognitive errors [7,8]. The concept of POCUS as a focused (“limited” or “goal‐directed”) exam [9,10] is in contrast to sonography performed by an imaging specialist/cardiologist (Table 18.1). Finally, POCUS provides relevant information that is otherwise unattainable on physical examination alone and is therefore complementary to triage, physical examination, and other point‐of‐care diagnostic and clinical tests or findings; it does not replace them. Evidence in human medicine supports that POCUS can result in improved clinical decision‐making [11,12], and when combined with other clinical findings, confirms the suspected clinical diagnosis in up to 50% of cases and supports a change in the initial diagnosis in 23% of cases [13]. With the exception of ultrasound‐guided regional anesthesia and vascular access, POCUS is in its infancy in veterinary anesthesia. In contrast, POCUS is widely used in human anesthesia to answer specific questions in daily patient management, narrow differential diagnoses, and improve the safety of routine procedures [14–20] (Table 18.2). When compared to traditional assessment techniques, human studies suggest anesthesiologist‐performed POCUS improves diagnostic accuracy of new pathologies as well as assessing the severity of known pathologies [21], and impacts perioperative management decisions [21–23]. Currently, perioperative POCUS (P‐POCUS) is most widely used for cardiovascular evaluation [16,22,24,25], pulmonary/airway [23,26–28], and abdominal/gastric [29,30] examinations, although it can be used to augment almost all aspects of the physical exam (Table 18.2). With growing evidence demonstrating the efficacy of anesthetist‐performed POCUS, its uptake by human anesthesiology training programs is increasing. A 2017 survey reported 100% (13/13) of academic anesthesiology programs surveyed had some POCUS training as part of their residency training and 70–77% believed POCUS should be expanded beyond ultrasound‐guided vascular access and nerve blocks, which are currently part of the National Curriculum for Canadian Anesthesia Residency [31]. Additional POCUS applications that respondents felt should be included in anesthesiology residency programs included lung ultrasound and vena cava assessment in 12, abdominal ultrasound in 8, gastric ultrasound in 4, airway ultrasound in 3, and optic nerve sheath diameter in 1 out of the 13 academic anesthesiology programs surveyed [31]. Table 18.1 Comparison of formal and point‐of‐care ultrasound. The application of POCUS varies depending on the clinical setting (Fig. 18.1) [34]: (1) triage POCUS uses the minimum number of views possible to identify the most immediate life‐threatening and critical conditions; (2) serial POCUS is applied to monitor progression or resolution of pathology and response to therapy; (3) systemic or multiorgan POCUS is performed in more stable patients (with or without specialist assistance) to detect asymptomatic conditions, new developments, and/or to ensure sonographically detectable problems have not arisen before undertaking procedures, anesthesia, or discharge/service transfer; and (4) interventional POCUS is used to reduce complications of procedures where applicable (Fig. 18.1). Which POCUS examination(s) to choose will also depend on the pretest probability of a problem being present [7,8]. Though it is rapid, repeatable, and associated with minimal risk, POCUS should never be completed at the risk of compromising patient safety. The decision to apply POCUS should always be dictated by the context of the clinical setting encountered. Only if the patient is sufficiently stable should multiorgan, systemic POCUS be performed. In unstable patients, the objective is to identify immediately life‐threatening conditions with the minimum number of sonographic views possible, stabilize the patient, reassess the patient, and then decide if, and what, further point‐of‐care diagnostics, including POCUS are required. Table 18.2 Common point‐of‐care applications in human anesthesiology [4,32,33], which should be applicable to veterinary medicine, although veterinary research is lacking for most applications. Use of POCUS is an active field of research, with new applications identified regularly; however, applications employed should be determined by an individual sonographer’s proficiency. The American Society of Anesthesiologists (ASA) recently introduced a POCUS certification program with three primary applications (cardiac, lung, and abdominal ultrasound) and six secondary applications (airway, musculoskeletal/soft tissue, ocular, renal/genitourinary, transcranial Doppler, and deep venous thrombosis) [4,32,33,35]. In principle, these suggested primary and secondary applications in human anesthesiology are applicable in veterinary anesthesia (Table 18.2). However, in veterinary medicine Doppler is not routinely used, musculoskeletal and soft tissue POCUS have not been studied, and optic nerve sheath diameter (ONSD) is only just starting to be investigated in small animals. A case series in dogs suffering trauma reports monitoring ONSD values serially in two dogs [36], and reference intervals have been reported in dogs and cats [37–40]. A single study in cats (n = 57) suggests an ONSD of 1–1.4 mm indicates the absence of intracranial hypertension (ICH), while a value > 1.5 mm is suggestive of ICH (based on seven cats with suspected ICH) [38]. These three POCUS applications will not be covered in this chapter, and nerve blocks and vascular access are addressed elsewhere in this textbook. Figure 18.1 POCUS is patient centered and targeted. It is also integrative and has four general applications, which vary depending on the targeted objectives of the scan, the pretest probability of a problem being present (based on history, initial clinical exam, and other diagnostic findings), and the clinical setting encountered: (1) triage POCUS uses the minimum number of windows possible to identify the most immediate life‐threatening and critical conditions; (2) serial POCUS is applied to monitor progression or resolution of any pathology, and response to therapy; (3) systemic or multiorgan POCUS uses multiple windows in more stable patients (with or without specialist assistance) to detect asymptomatic conditions, new developments, and/or to ensure sonographically detectable problems have not arisen prior to undertaking procedures, anesthesia, or discharge/service transfer; and (4) therapeutic POCUS is used to reduce complications of interventions where applicable. POCUS, point‐of‐care ultrasound. Source: Dr. Søren Boysen, with permission. An advantage of POCUS is the ability to bring the ultrasound machine to the patient, limiting interference with patient care and comfort. Although some authors prefer to restrain patients in a specific position to perform POCUS and FAST exams [3,41,42], there is minimal evidence to support this practice and increasing evidence that restraining cats and dogs in a particular position can increase patient anxiety and stress [43]. The authors advocate performing POCUS in the position in which the patient is most comfortable. Given patient position may be dictated by surgical or other procedures in anesthetized animals, it is particularly important that anesthesiologists become familiar performing POCUS with animals in different positions. However, sonographers must be aware that localization of some sonographically identifiable pathology will change with patient positioning (e.g., fluid falls and gas rises). Where the probe is situated on the patient should be modified to ensure the location of positionally dependent pathology is assessed. Although certain sonographic examinations are facilitated by specific ultrasound probes, in general, a 5 mHz (larger dogs) to 7 mHz (smaller dogs and cats) micro‐convex probe and B‐mode imaging are used for nearly all POCUS applications [1–3,42]. For vascular access and ultrasound‐guided nerve blocks, a linear probe is generally preferred over a micro‐convex probe, though the latter can still be used. To maintain efficiency, fur is not clipped but parted to provide access to the skin, and alcohol is used as the coupling agent, with or without gel [2,41]. In unstable patients where time is critical, the authors use only alcohol as the coupling agent. Caution is advised in some situations, as alcohol may cause radiographic artifact and poses a fire hazard if electrocautery, defibrillators, or laser devices are used following its application. In more stable patients, gel can be added to the probe head in addition to applying alcohol to the patient if image quality is suboptimal. Alternatively, fur can be clipped and ultrasound gel applied as the sole coupling agent at the probe–skin interface, particularly if image quality remains suboptimal [1]. Assessment of the airway typically involves assessment of the cervical region for evaluation of correct endotracheal (ET) tube placement. A recent meta‐analysis in human medicine documented a pooled sensitivity of 0.93 (95% CI: 0.86–0.96) and specificity of 0.97 (95% CI: 0.95–0.98) for identification of esophageal intubation using POCUS [44]. Although there is a lack of research in veterinary medicine, a small cadaveric study in dogs (n = 6) demonstrated sensitivity of 62–75% and specificity 38–62.5% for identification of esophageal intubation when the probe was placed on the ventral neck just caudal to the larynx near the jugular furrow, depending on the experience of the operator [45]. ET intubation is recognized by identifying the presence of only one hyperechoic curved line with distal reverberation artifact, created by the presence of air inside a correctly placed ET tube (Fig. 18.2). Esophageal intubation is recognized by the presence of two hyperechoic curved lines with distal reverberation artifact, created by the presence of air inside the trachea and air contained within an esophageally placed ET tube [45] (Fig. 18.2). Figure 18.2 Labeled still ultrasound images of tracheal versus esophageal intubation. Right: Tracheal intubation where only one circular gas‐filled structure (intubated trachea) with distal shadowing is visible. Left: With esophageal intubation, a second gas‐filled structure with distal shadowing (air‐filled endotracheal tube within the lumen of the esophagus) becomes visible in addition to the gas‐filled trachea. Source: Dr. Søren Boysen, with permission. To perform PLUS, adjust the machine depth setting (according to body condition) so the field of view in the ultrasound image extends to approximately 4–6 cm and the pleural line (P‐line) is visible at the junction between the proximal and middle thirds of the window/field of view. Adjust the gain to maximize appearance of the thoracic wall, while also allowing any far‐field image artifact to be visible (Fig. 18.4). To help differentiate normal from abnormal PLUS findings, operators should become familiar with several PLUS lines and signs that can be identified in healthy animals and know how these lines and signs change in animals with suspected pathology. These include the BAT sign, P‐line, lung sliding, lung pulse, A‐lines, B‐lines, and the ACS. The BAT sign (i.e., gator sign) refers to the characteristic appearance of the P‐line and adjacent ribs [42,54]. It is identified when the probe is positioned over lung, between and perpendicular to the ribs, with the indicator marker directed cranially (Fig. 18.4). If placed between and parallel to the ribs, only the P‐line is seen, which is not interrupted by the ribs [54]. Using the BAT mnemonic – “ultrasound beams do not traverse Bone or Air with the probe Transverse to the ribs” – helps sonographers identify key anatomic structures and understand what is being visualized, as follows: Figure 18.3 Schematic images of pleura and lung ultrasound (PLUS) to assess for pneumothorax, lung pathology, and pleural effusion using sonographically defined landmarks and lung borders with patients in a sternal or standing position. A. Combined comprehensive PLUS protocol often used in more stable patients to assess the pleura, pleural space, and lungs bilaterally. The subxiphoid site is also included (red highlighted probe) to assess the caudal lung and pleural space regions not otherwise accessible transthoracically. B. Abbreviated pleural space protocol to rapidly assess the abdominal curtain sign (ACS) and most gravity‐independent sites for pneumothorax in respiratory‐compromised patients. In the sternal or standing patient (shown), with the probe situated perpendicular to the ribs (probe indicator marker directed cranially), slide caudally from the starting point (just behind the front limb) until the ACS is identified, followed by sweeping the probe dorsally along the ACS until the hypaxial muscles are encountered (loss of the pleural line; curved red arrow). The probe is then swept ventrally off the hypaxial muscles until the pleural line reappears (the most gravity‐independent site; short red arrow). If the patient is in lateral recumbency (not shown), the widest non‐gravity region of the thorax and ACS are assessed. C. Abbreviated lung scanning protocol to rapidly assess multiple lung surface regions in the dorsal, middle, and ventral thirds of the thorax with the probe orientated perpendicular to the ribs using an S‐shaped pattern (blue arrow) in respiratory‐compromised patients. Lung pathology tends to be less affected by position than pleural space pathology, and the same lung ultrasound protocol can be used regardless of patient position, however, given some lung pathology can concentrate locally or regionally (e.g., aspiration pneumonia and positional atelectasis) different regions of the lung can be emphasized. At the pericardio‐diaphragmatic (PD) window (yellow asterisk), the probe can be turned parallel to the ribs (see image D) or maintained in a perpendicular orientation to scan the ventral lung surfaces. Time permitting, the subxiphoid site can also be assessed (not shown). D. Abbreviated pleural space and lung ultrasound protocol to rapidly assess the most gravity‐dependent pleural sites for pleural effusion and ventral lung pathology in respiratory‐compromised patients. Identifying the PD window (red arrow) with the probe situated perpendicular to the ribs helps differentiate pleural and pericardial effusion. In the sternal or standing patient, the probe can be turned parallel to the ribs at the PD window (with the probe indicator marker directed dorsally) and slid ventrally until the sternal muscles fill 1/3 to 1/2 of the ultrasound image. This allows the most ventral pleural regions to be assessed for the presence of smaller volume pleural effusion. With the probe maintained in parallel orientation to the ribs, it can be slid both dorsally and ventrally within each intercostal space (yellow arrows) to assess both the ventral lung regions and the most ventral pleural space regions, respectively, from the ACS caudally to the thoracic inlet cranially. If the patient is in lateral recumbency, the widest gravity‐dependent region of the thorax should be assessed for pleural effusion (not shown). Time permitting the subxiphoid site can also be included (not shown) (Video 18.1). Source: Adapted from Boysen et al. [46]. The pleural line (P‐line) is the bright white line below the soft tissue structures of the thoracic wall (Fig. 18.4), and is either comprised of the parietal and visceral pleura when the lung apposes the chest wall, or is composed of only the parietal pleura when the lung is separated from the chest wall [54,57,58]. Figure 18.4 Labeled and unlabeled still and overlaid schematic images of the “BAT sign” and A‐lines. The proximal rib surface (PRS) of two adjacent ribs forms the wings of the bat while the pleural line (P‐line) forms the body of the bat when the probe is situated perpendicular to the ribs. The gain is adjusted based on the appearance of the thoracic wall (region above the pleural line) while also allowing A‐lines to be visualized in the far field of the image. The depth is at 7 cm to allow far‐field A‐lines and B‐lines (not shown) to be appreciated. The depth could be decreased in this example to put the pleural line at the junction between the proximal and middle thirds of the ultrasound image, which will make lung sliding easier to identify in real time. SQ, subcutaneous tissues; IC, intercostal muscles; PRS, proximal rib surface. Source: Dr. Søren Boysen, with permission. A‐lines are a type of reverberation artifact appearing as hyperechoic (white) horizontal lines that run parallel and equidistant from the skin to the P‐line below a soft tissue‐to‐air interface [54–58,59] (Fig. 18.4). They are created by the reflection of the ultrasound beam between two highly reflective surfaces: (1) the ultrasound probe and (2) the soft tissue–air interface at the P‐line. Figure 18.5 Schematic and still image of a single B‐line originating at the lung surface from the pleural line when the lung is in contact with the chest wall. A single B‐line can be a normal finding, although interpretation should always be within clinical context. RS, rib shadow; B, B‐line; P, pleural line. Source: Dr. Søren Boysen, with permission. The abdominal curtain sign (ACS) is a normal sonographic artifact of healthy animals that orientates the operator to the area of the thorax being viewed (caudolateral lung margin) and can be used to identify pleural space (pneumothorax and pleural effusion) and lung (consolidation) pathology [51,58,70]. It is defined as the sharply demarcated vertical edge artifact that occurs along the caudolateral lung margins where air‐filled lung overlies the diaphragm and soft tissues of the abdomen at the costophrenic recess [46,51,54,70]. The ACS is created by the combination of two factors: (1) the acoustic impedance mismatch between gas (air‐filled lung) and adjacent soft tissue (abdominal structures) that casts the characteristic sharp vertical artifact at the interface between the two; and (2) the anatomical relationship of the thorax with the abdomen, resulting in the costophrenic recess covering parts of the cranial abdomen and the diaphragm, which abuts against air‐filled lung cranially in healthy patients [46,51,54,58,70]. Figure 18.6 Radiographic, schematic, and still images of the location and sonographic appearance of a normal abdominal curtain sign (ACS) artifact. A. Thoracic radiograph of a dog showing the probe location to identify the ACS. The dotted red arrow represents the division where air‐filled lung (left of arrow) overlies the soft tissue abdominal structures (right of arrow) at the costophrenic recess. The dotted white arrow shows the diaphragm curving away from the chest wall, which is not visible due to overlying air‐filled lung. B. Schematic image showing ultrasonographic image with the probe positioned as shown in A. C. Still ultrasound image showing the normal vertical edge artifact (yellow arrows) of the ACS. P, pleural line; GB, gallbladder. Source: Dr. Søren Boysen, with permission. Ultrasound can only be used to interpret characteristics at the surface of a soft tissue–air interface because air/gas is a strong ultrasound beam reflector, making it difficult to visualize structures below the soft tissue–air interface. Therefore, pathology lying deep to the lung surface cannot be visualized. Fortunately, most (> 90%) alveolar–interstitial pathology (e.g., pulmonary edema, contusions, aspiration pneumonia, and fibrosis) results in B‐lines and/or lung consolidations that are detectable at the lung surface with sonography [56,70] (Table 18.3). In human medicine, lung ultrasound has been used to diagnose endobronchial intubation with the advantage of being a rapid modality, compared to confirmation with thoracic radiography [61]. In humans, bronchial intubations occur in 5–15% of intended tracheal intubations in both pre‐hospital and in‐patient settings [71–75], and 6–25% of cases go unrecognized [71,76–78]. The consequences of single‐lung intubation include suboptimal ventilation/oxygenation and barotrauma, which may result in pneumothorax and unilateral pulmonary edema [61,79]. The application of intended one‐lung ventilation in small animals is well‐established for the management of various underlying pathologies [80–83]. Although there are several techniques reported to confirm correct placement of one‐lung devices in dogs and cats [82,84], the use of PLUS to assess bilateral versus unilateral lung intubation has not been studied, despite first being reported in human medicine in 2003 [61,85]. One‐lung ventilation can be confirmed through detection of a lung pulse and the absence of lung sliding on the non‐intubated side of the thorax. This contrasts with the presence of lung sliding (with or without a lung pulse) on the contralateral, intubated, side of the thorax (Fig. 18.8). One‐lung intubation is associated with atelectasis, which makes the lung pulse easier to visualize [57,58,61]. In contrast, endobronchial intubation is unlikely when bilateral lung sliding is present during respiration. Figure 18.7 Ultrasound still images. A. Labeled ultrasound still images. B. and C. Schematic images showing the normal movement of the abdominal curtain sign during inspiration (bottom row) and expiration (top row). The ultrasound probe was positioned over the right paralumbar region at the level of the right kidney, over the liver, between the 11th and 12th intercostal spaces, and incorporates the air/soft tissue interface between the abdomen and the thorax, which appears as a vertical edge (abdominal curtain sign). The position of the abdominal curtain sign (white arrowhead) and abdominal contents (yellow arrowhead) at end‐expiration are shown. During inspiration, the vertical edge of the curtain sign (vertical dotted white line) and the abdominal contents move caudally (white and yellow arrows, respectively). Although the curtain sign may move more than the abdominal contents, the two move in the same direction during the same phases of respiration. Dashed lines represent vertical edges (air/soft tissue interface). Solid line represents center of image; R, rib; RS, rib shadow; P, pleural line; A, A‐line; L, liver; RK, right kidney; Arrow shows direction of movement of the vertical edge during inspiration (also see Video 18.5). Source: Boysen et al. [46], with permission from Frontiers Media S.A. / CC BY 4.0. Table 18.3 Causes of diffuse and localized B‐lines along with common history and clinical findings. Human studies demonstrate a sensitivity and specificity of 87% and 97%, respectively, with lung ultrasound, and 28% and 100%, respectively, with thoracic radiography for identification of pneumothorax [86]. The clinical accuracy of ultrasound to diagnose pneumothorax in companion animals is unclear and determination of sensitivity and specificity is hindered by a failure to compare findings with accepted reference standards, and/or by the inclusion of only small case numbers [6,36,42,46,58,87–89]. For example, recent studies comparing the veterinary bedside lung ultrasound exam (VetBLUE) or TFAST protocols to computed tomography (CT) correctly identified pneumothorax in 3/7, 3/6, and 1/6 cases, respectively [6,87,89]. A well‐controlled experimental study that induced small‐volume pneumothorax (2–10 mL/kg of air) in dogs under anesthesia (n = 9) suggests lung ultrasound is more sensitive (89% versus 67%) and equally specific (89%) to radiographs compared with CT when multiple lung ultrasound criteria are combined to diagnose pneumothorax in dogs; however, translation of these findings to patients with naturally occurring and larger‐volume pneumothorax in the clinical setting has not been performed [88]. There is even less information available regarding lung ultrasound to diagnose pneumothorax in cats, with only two small clinical studies that were combined with findings from dogs, neither of which reported any cats with confirmed pneumothorax [87,89]. The accuracy of ultrasound to diagnose pneumothorax likely depends on the experience of the sonographer, underlying pathology, patient positioning, scanning protocol, and criteria used to diagnose pneumothorax [36,42,78,87,89]. If the pleura is in contact, pneumothorax is ruled out. The following sonographic findings confirm the pleura is in apposition below the probe (Fig. 18.9): (1) detection of lung sliding and/or a lung pulse, and (2) detection of B‐lines and/or lung consolidation (see below) arising from the P‐line [58,88]. Figure 18.8 Schematic image depicting one‐lung intubation and a comparison of the two hemithoraces with regards to the sonographic identification of the lung pulse and lung sliding during spontaneous and positive‐pressure ventilation (PPV). ET, endotracheal. Source: Dr. Søren Boysen, with permission. Figure 18.9 Schematic image of the sonographic finding on either side of the chest when a moderate unilateral pneumothorax is present (pneumothorax shown in black). Each arrow represents different sonographic findings when the probe is placed over an area where there is a pneumothorax, and where there is lung in contact with the chest wall. The location of the abdominal curtain sign (ACS) is also shown. A pneumothorax will often result in an abnormal ACS due to free pleural gas interacting with the costophrenic recess when it displaces the lung lobe cranially. With massive pneumothorax where lung fails to recontact the chest wall, there will not be a lung point and there will be an absence of B‐lines/consolidation, lung pulse, and lung sliding over the entire hemithorax. Source: Dr. Søren Boysen, with permission. Figure 18.10 Still images and schematic of an asynchronous curtain sign at end‐expiration (top row) and end‐inspiration (bottom row). The ultrasound probe was positioned over the right paralumbar region just below the right kidney, over the liver, between the 9th and 10th intercostal spaces, and incorporates the air/soft tissue interface between the abdomen and the thorax, which appears as a vertical edge (curtain sign). During inspiration, the vertical edge of the curtain sign moves in the opposite direction (cranially) of the abdominal contents (caudally). Dashed lines represent vertical edges (air/soft tissue interface). Solid line represents center of image. P, pleural line; A, A‐line; L, liver; asterisk “*”, hepatic vessel. Solid arrow shows direction of movement of the vertical edge during inspiration. Dashed arrow shows direction of movement of the abdominal contents during inspiration. Length of arrow represents degree of movement relative to the image above (also see Video 18.8). Source: Boysen et al. [46], with permission from Frontiers Media S.A. / CC BY 4.0. Figure 18.11 Still images and schematic of a double curtain sign at end‐expiration (top row), mid‐inspiration (middle row), and end‐inspiration (bottom row). The ultrasound probe is located over the liver, about halfway up the thorax, between the 7th and 8th intercostal spaces, over the air/soft tissue interface between the abdomen and the thorax. During inspiration, two vertical edges of the curtain sign are visible in the same sonographic window. The asterisk “*” represents a region where the probe is situated over the diaphragm and associated underlying soft tissue structures at the costophrenic recess. Dashed lines represent vertical edges (air/soft tissue interface). Solid line represents center of image. R, rib; RS, rib shadow; P, pleural line; A, A‐line; L, liver. Arrows show movement of the vertical edges in opposite directions during inspiration in this example. Length of arrow represents degree of movement relative to the image above (also see Video 18.8). Source: Boysen et al. [46], with permission from Frontiers Media S.A. / CC BY 4.0. With smaller volume effusions, fluid occupies the costophrenic angle/recess, causing minimal compressive atelectasis of the surrounding lung tissue. In this scenario, the dynamic motion of the air‐filled lung expanding across the pleural effusion and soft tissue abdominal structures is still seen; however, the ACS does not overlap the costophrenic recess as much as in the absence of effusion [51,54]. The diaphragm can be seen curving away from the chest wall due to the presence of pleural effusion accumulating within the costophrenic recess [51,54]. As the quantity of effusion increases, the lung progressively covers less and less of the costophrenic recess, even uncovering it on inspiration, and the lungs may become atelectatic, appearing as floating tissue‐like structures (termed the “jellyfish” sign in human medicine) [54,70,91]. In contrast to pleural effusion, pericardial effusion is contained and curves away from the diaphragm following the contour of the heart. To identify very small to scant volumes of pleural effusion that might not cause respiratory distress, but might be diagnostically important to sample and analyze, the most ventral pleural regions between the sternal muscles and the lung should be assessed from the diaphragm to the thoracic inlet, with the patient in a sternal or standing position (Fig. 18.13) [46]. The ventral pleural regions can be identified by placing the probe parallel to the ribs in the ventral third of the thorax and sliding the probe ventrally until the sternal muscles become visible within the ultrasound image (Fig. 18.13). Figure 18.12 Photograph, still, and schematic images of the location, normal appearance, and abnormal findings identified at the pericardio‐diaphragmatic (PD) window. (Top) Photograph of a dog demonstrating the location of the probe to find the PD window. (2nd row) Still (left) and schematic image (right) showing the normal appearance of the PD window when the heart and diaphragm are visible within the same image. (3rd row) Still (left) and schematic image (right) of pleural effusion. (4th row) Still (left) and schematic image (right) of pericardial effusion, which is easily differentiated from pleural effusion at this location. PE, pleural effusion; MT, mediastinal triangle; LV, left ventricle; RV, right ventricle. Source: Dr. Søren Boysen, with permission. Figure 18.13 Schematic and still ultrasound images of the lung, pleura, and sternal muscle interfaces. A–C. Schematic, labeled still, and unlabeled still ultrasound image of a healthy patient. D. Labeled still image of pleural effusion (PLE) located ventrally between the sternal muscles and lung. Source: Dr. Søren Boysen, with permission. Figure 18.14 Increased to coalescing B‐lines. Note the absence of ribs above the pleural line and the absence of rib shadows because the probe is orientated parallel and between two ribs. The finding of > 3 B‐lines in any sonographic window is considered abnormal. Source: Dr. Søren Boysen, with permission. Table 18.4 Sonographic findings seen with different pleural space and lung pathologies which, when mapped over the entire hemithorax, create pleural space and lung ultrasound (PLUS) profiles. CVC, caudal vena cava; LA:Ao, left atrial to aortic root ratio; PE, pulmonary embolism Healthy lung comprises roughly 85–95 % air and 5–15 % non‐aerated tissue [55,59,62,63,98]. A change in the ratio of aerated to non‐aerated lung within 1–3 mm of the lung surface results in specific abnormal lung ultrasound findings, mainly increased B‐lines and lung consolidation. When there is sufficient air within the lung tissue at its periphery (>10% lung aeration), the soft tissue–air interface at the P‐line is maintained and the ultrasound beam is reflected from the lung surface, rendering the lung invisible [62]. In this situation, it is only possible to visualize lung pathology through interpretation of lung surface artifacts such as increased B‐lines [55,59,62,63,98]. When the ratio of aerated to non‐aerated lung tissue directly below the visceral pleura falls below 5–10% (i.e., 90% consolidation), the acoustic impedance between the thoracic wall and lung is decreased to a point the lung ultrasound beam is no longer reflected from the lung surface, but is instead transmitted through the underlying lung tissue [97]. Similar to causes of increased B‐lines, lung consolidation can occur as a result of atelectasis, hemorrhage, bronchopneumonia, thromboembolism, lung lobe torsion, neoplasia, and inflammatory conditions such as pulmonary contusions and acute lung injury. Unlike B‐lines, which can occasionally be normal and will increase with even mild increases in lung density, consolidation is always abnormal and only occurs when pathology is severe enough that the ultrasound beam can traverse lung tissue. Consolidation can be translobar (traversing the entire lung lobe from one surface to the other), or partial (partial thickness, where the consolidated region within the lobe encounters air‐filled lung distal to the consolidated region) [59,70]. Although many pathologies can result in consolidation, most will result in three common ultrasonographic appearances (Table 18.5): Table 18.5 Classification of lung consolidation and common differential diagnoses. Figure 18.15 Schematic and still image of air bronchograms. White punctate and linear structures within consolidated lung represent bronchi that still contain air (air bronchograms) and black punctate and linear structures represent fluid filling the bronchi (fluid bronchograms). Note that lung sliding tends to be lost with consolidated lung as the soft tissue–air interface is lost. The still image shows mixed fluid and air bronchograms in the same pathologic lung region. Source: Dr. Søren Boysen, with permission. The “wedge” sign is reported in the human literature and is often associated with pulmonary thromboembolism when identified in patients with concurrent deep vein thrombosis. The wedge sign and its significance have not been well documented in small animals. In veterinary patients, differentiation of the two is challenging as it can be difficult to obtain optimal views. It is possible to find mixed fluid and air bronchograms within the same consolidated region of lung or in different regions of the same lung lobe. In human medicine, air bronchograms help classify the type of consolidation and possibly the underlying cause. When bronchioles within consolidated lung remain patent, communicating with the large airways, changes in air movement (> 1 mm) with tidal volume can create visible movement of air within smaller airways [99,100]. This movement of air within the small airways creates a “shimmering” referred to as a “dynamic air bronchogram,” and appears similar to the shimmer of lung sliding (Video 18.10). Dynamic air bronchograms are most often seen with pneumonia and inflammatory causes of lung consolidation. In contrast, static air bronchograms are often seen with atelectasis but can also be seen with inflammatory causes of lung consolidation, including acute respiratory distress syndrome (ARDS) and aspiration pneumonia. A complete lack of air bronchograms is suggestive of complete atelectasis, although severe pneumonia or lobar hemorrhage cannot be excluded. Veterinary abdominal point‐of‐care ultrasound (APOCUS) has expanded from the original FAST scan in trauma patients and searching for free abdominal fluid to become a clinical tool for the management and diagnosis of any patient with suspected abdominal pathology [1–3,101–112] (Table 18.6). Basic ultrasound knowledge of abdominal organ anatomy is essential; however, a detailed assessment of organs should be performed by a radiologist if abnormal parenchymal organ structure is detected [113–117]. Most veterinary APOCUS questions can be answered by assessing five windows of the abdomen, which is the authors’ preferred general APOCUS approach (5‐point APOCUS, Fig. 18.16). To answer questions rapidly, but thoroughly, at least two sonographic planes (short and long axis), with fanning and rocking probe movements should be performed at each window [1,2,87]. It is important to remember that APOCUS windows and regions assessed should be adapted based on the clinical setting encountered. For example, patient positioning can impact where some pathologies accumulate (fluid falls and gas rises), and APOCUS should be performed and adapted to patient positioning in order to maximize the chances of detecting pathology. The specific goal‐directed questions and key organs to assess at each window are listed in Table 18.6 and illustrated in Fig. 18.16. In human anesthesia, APOCUS has been used to look for free abdominal fluid, particularly in patients with sudden clinical deterioration, such as hypotension [116–118], to stratify patients at risk for pulmonary aspiration (through gastric volume assessment), and to monitor urinary bladder volume. Gastric APOCUS is applied in humans as a limited examination to assess gastric content (empty, anechoic “clear” fluid, hyperechoic “thick” fluid/solid), and volume, with the ultimate goal of assessing pulmonary aspiration risk [119–121]. The findings from gastric APOCUS may help guide airway and/or anesthetic management in the acute care setting to reduce the risk of aspiration [19]. An empty stomach identified on APOCUS carries a low aspiration risk. In contrast, thick fluid content and particulate fluid of solid gastric content are associated with an increased risk of aspiration. In humans, a sonographically determined gastric volume of < 1.5 mL/kg is normal in fasted patients (baseline gastric secretions) and associated with a low aspiration risk. Conversely, volumes > 1.5 mL/kg are uncommon in fasted individuals, and suggest incomplete gastric emptying and possibly higher aspiration risk. Although a strict gastric volume threshold associated with aspiration risk is controversial, clinical data strongly suggest that volumes of up to 1.5 mL/kg are normal and safe in fasted humans [122–124]. A semiquantitative three‐point grading system has also been developed in human anesthesiology to differentiate low from high gastric volume states and the risk of aspiration pneumonia [122–124]. Although gastrointestinal motility and emptying have been evaluated and used as a point‐of‐care assessment in small animals, the role of gastric POCUS to stratify aspiration risk in animals is unclear [103,125–128]. Table 18.6 Clinically driven questions that can be answered in small animals with a 5‐point abdominal POCUS (APOCUS) exam, scanning through multiple planes in both short‐ and long‐axis orientations at each window. Figure 18.16 5‐point abdominal point‐of‐care ultrasound (APOCUS) exam and the binary questions that have been evaluated at each site in small animals. The APOCUS exam should be completed in the position the patient is most comfortable (left lateral recumbency shown in this image). Depending on the question to answer and patient status, the probe is rocked and fanned through 45 degrees in both the short‐ and long‐axis planes at all five sites. Consider the pretest probability of the pathology in question and then modify the protocol to increase the chance of detecting the specific pathology based on how the patient is positioned and where pathology is likely to accumulate (gas rises, fluid falls). CPR, cardiopulmonary resuscitation; CVC, caudal vena cava. Source: Dr. Vivian Leung, with permission. Urinary bladder volume calculation, and estimation of urine production, can help diagnose postoperative urinary retention and reduce postoperative urinary catheterization in human anesthesiology departments [129,130]. Sonographic urinary bladder volume estimation has been reported in veterinary medicine using both 2D and 3D ultrasound. A recent canine study suggests the formula L × W × D × 0.52 may be the most accurate of the urinary volume formulas studied [131–133]. Urinary bladder volume calculation and a potential role in peri‐ and postoperative patient management of veterinary patients have not been reported despite the calculation of urinary bladder volume being easy to perform. With cardiac POCUS (CPOCUS), the sonographer uses a specific imaging protocol (e.g., right parasternal short‐axis left atrial to aortic root [LA:Ao] ratio) to answer a specific question or series of questions by interpreting views of one or a few preselected targets, with responses typically binary (present or absent). Although CPOCUS is less sensitive and specific than comprehensive echocardiography, it provides valuable information to supplement physiologic data and physical examination findings [134–137]. The American Society of Echocardiography has established guidelines for CPOCUS use by acute care providers [138,139]. In human anesthesiology, CPOCUS is useful for the acute assessment of gross cardiovascular function, assessment of causes of hemodynamic instability, and cardiac assessment during cardiac arrest [140–142]. It can also accurately detect a state of low cardiac preload and facilitates rapid, goal‐directed fluid resuscitation [143,144]. More specifically, CPOCUS is used to qualitatively evaluate the size of the cardiac chambers, the thickness of the walls, ventricular function, intracardiac volume status, and the presence of pericardial effusion or air emboli [134,140,145,146]. Although clinical studies are lacking, most of these variables have also been evaluated with CPOCUS in companion animals (Table 18.7). In addition, CPOCUS compliments PLUS in the evaluation of patients with respiratory distress (i.e., the finding of diffuse bilaterally increased B‐lines should prompt CPOCUS evaluation to confirm or rule out cardiac causes of pulmonary edema). In contrast, the absence of B‐lines in a patient with respiratory distress essentially rules out cardiac causes, and other respiratory pathologies should be considered. Finally, it is important to realize that CPOCUS has limited applications in human patients with congenital heart disease and subtle chronic pathology as it focuses on the identification of acute conditions (pericardial effusion and hypovolemia) and assessment of global myocardial function rather than detailed structural diagnostics or measurements [147]. This may limit its application in veterinary anesthesiology to identify the cause and significance of preanesthetic cardiac murmurs identified on physical examination, although research in this area is lacking. If left atrial (LA) enlargement, valve thickening, or subtle changes in wall thickness or contractility are noted, echocardiography performed by a cardiologist is recommended. Applications of veterinary CPOCUS are presented in Table 18.7. Table 18.7 Cardiac POCUS windows, structures of interest, clinically driven (often binary response) questions to answer, and suggested guidelines for interpretation of findings. LA:Ao, left atrial to aortic root ratio; RV:LV, right ventricular to left ventricular ratio. Extensive quantitative calculations are not a goal of CPOCUS. When time and training allow, simple calculations can be performed, but are not necessary. In human and veterinary studies, a good correlation between visually estimated (qualitative) and formally measured (quantitative) cardiac parameters has been reported [148–151]. Although a phased array transducer is advantageous in obtaining echocardiographic images, CPOCUS is often performed using a micro‐convex curvilinear transducer. Although M‐mode recordings allow quantitative measurement of cardiac dimensions and analysis of motion patterns, most CPOCUS questions can be answered using B‐mode alone. CPOCUS incorporates right parasternal short‐axis (Fig. 18.17) and long‐axis views, the subxiphoid view, and occasionally, left‐sided views. Acquiring all views is often unnecessary. For example, the LA:Ao ratio can still have diagnostic value, even if it is the only view obtained [151–153]. Furthermore, although left‐sided views can be helpful in some situations (e.g., identification of intracardiac thrombi in cats), they are not required to answer most CPOCUS questions [154]. There are three key right parasternal short‐axis views that can answer most CPOCUS questions (Fig. 18.18) [152,154,155]: Figure 18.17 Radiographic, schematic, and still images of the different right parasternal short‐axis views starting at the apex of the left ventricle and moving toward the heart base through the papillary muscle level, mitral valve, and finally the left atrial to aortic root (La:Ao). The corresponding cardiac still images are labeled: “left apex,” “mushroom,” “fish mouth,” and “La:Ao,” also referred to as the “Mercedes and the whale.” The heart base image above the left atrial to aortic root is not shown in this figure. LA, left atrium; RA, right atrium; RV, right ventricle; PA, pulmonary artery; LV, left ventricle; MV, mitral valve. Source: Dr. Søren Boysen, with permission. The four‐chamber view is arguably the most common right parasternal long‐axis view assessed during CPOCUS (Fig. 18.19). With this view the sonographer can subjectively evaluate the size of all cardiac chambers [152,158]; left atrium (LA), left ventricle (LV), right atrium (RA), and right ventricle (RV). The RA and the LA should be of similar size (1:1 ratio). The interatrial septum should lie in a relatively neutral position and not bulge into either atrium. The RV internal chamber diameter is approximately one‐third that of the LV internal diameter. The free wall of the RV equals one‐third to one‐half the thickness of the LV free wall. The interventricular septum and the LV free wall are normally similar in thickness. Interpretation of changes in chamber size, wall thickness, and contractility in the right parasternal long‐axis views are similar to changes in the right parasternal short‐axis views. The four‐chamber view may be more sensitive for diagnosing cardiac tamponade compared to the short‐axis views. Tamponade appears as a compression of the RA‐free wall into the atrium, intermittently reducing atrial chamber size (RA wall compressed inwards during systole; Fig. 18.20). However, it should be kept in mind that tamponade is a clinical diagnosis and the finding of shock in a patient with identifiable pericardial effusion should prompt an increased index of suspicion for tamponade. The subxiphoid view provides a useful acoustic window into the caudal thorax, which can identify pericardial and/or pleural effusion and assess caudal lung regions not accessible transthoracically [1,159]. The caudal vena cava (CVC) can also be evaluated at this site (see below). All CPOCUS findings should be interpreted in light of clinical examination and other POCUS findings. Most patients presenting with respiratory distress secondary to congestive heart failure have LA:Ao ratios ≥ 2, well above upper reference limits [151,154,159]. If the LA is enlarged, it suggests increased LA volume and/or pressure, which is often associated with left‐sided congestive heart failure or iatrogenic fluid overload. Alternatively, in cats (not yet studied in dogs), if > 3 times the area of the aorta can subjectively fit within the LA, the LA should be considered enlarged (Fig. 18.21) [152]. Using both of these methods in cats is a good way to help confirm if the LA is truly enlarged [154]. A small LA:Ao (≤1:1) suggests pseudohypertrophy. Pseudohypertrophy results from hypovolemia and decreased ventricular filling, which causes decreased LA and LV chamber size, and the appearance of a thickened interventricular septum and ventricular free wall [160,161]. This resolves with restoration of effective circulating volume. The subjective identification of an enlarged LV lumen may suggest volume overload or conditions causing myocardial dysfunction (e.g., dilated cardiomyopathy) [154,155,158]. Both volume overload and dilated cardiomyopathy can cause an enlarged LA and increased B‐lines on lung ultrasound. Assessing LV contractility is important for differentiating the two conditions (see below). The subjective finding of a decreased LV lumen size with thickened ventricular walls may suggest pseudohypertrophy secondary to volume depletion or true myocardial hypertrophy. To help differentiate pseudohypertrophy from hypertrophic cardiomyopathy, LA size should be evaluated: LA size is normal to small in patients with pseudohypertrophy and often enlarged in patients with cardiomyopathy. A loss of the visible ventricular chamber during systole, known as “ventricular chamber obliteration,” may reflect severe hypovolemia due to markedly decreased ventricular filling pressures and potential impending cardiovascular collapse [33]. In the absence of systemic disease (e.g., hyperthyroidism, systemic hypertension, subaortic stenosis, and acromegaly), increased LV wall thickness is often associated with hypertrophic cardiomyopathy in cats but may also be seen with pseudohypertrophy in both dogs and cats. Figure 18.18 Two‐dimensional short‐axis view, right parasternal window showing still images of the three key views used to answer most cardiac POCUS questions. A. Mushroom view, often the first view obtained, which is located at the midventricular level where the papillary muscles are visible (white arrows). B. Fish mouth view, identified by slowly fanning the transducer dorsally from the mushroom view directing the ultrasound beams toward the base of the heart until the mitral valve (MV) becomes apparent within the left ventricular lumen (LV). C. Mercedes and the whale view, obtained by fanning the ultrasound beam toward the base of the heart from the mushroom/fish mouth view. Image contains a short‐axis view of the left atrium‐to‐aortic root ratio (La:Ao). (La, “whale”) and aortic root (Ao, “Mercedes”). D. Still image demonstrating the objective measurement of the La:Ao (yellow dotted arrows), although this measurement is often made subjectively during cardiac POCUS. IVS, interventricular septum; RV, right ventricle. Source: Lindsey Strang, RVT, VTS (ECC), with permission. Figure 18.19 Two‐dimensional long‐axis view, right parasternal window four‐chamber view of the heart showing the left ventricle (LV), left atrium (LA), right atrium (RA), and right ventricle (RV). Source: Lindsey Strang, RVT, VTS (ECC), with permission. Figure 18.20 Tamponade secondary to pericardial effusion. The right atrium is collapsed and concave (white arrows). Source: Lindsey Strang, RVT, VTS (ECC), with permission. Figure 18.21 Still ultrasound images of a normal‐sized left atrium (left) and a much‐enlarged left atrium (right). In cats, the number of aortic areas that can fit within the left atrium can be used to subjectively decide if the left atrium is enlarged. The image on the left cannot fit more than 2.5 left aortic areas within the left atrium, while is possible to fit more than seven aortic areas within the left atrium in the image on the right. Source: Dr. Søren Boysen, with permission. Ventricular contractility can be subjectively assessed by estimating the relative change in size of the LV lumen during diastole and systole, which should be roughly 30–50% in dogs and 40–60% in cats. With practice, and by scanning healthy animals, a subjective evaluation of ventricular contraction is usually sufficient to determine if contractile abnormalities are present [154,158]. Hyperdynamic/increased contractile states can occur in hypervolemic patients (provided there is no concurrent myocardial dysfunction), high sympathetic drive states (e.g., stress), and in patients that have decreased afterload (e.g., arterial vasodilation) without myocardial dysfunction [33,134]. Decreased contractility can be seen with hypovolemia in the absence of myocardial dysfunction (due to decreased myocardial stretch), conditions associated with poor myocardial contractility (e.g., dilated cardiomyopathy or sepsis), and cases with increased afterload (e.g., severe arterial vasoconstriction) [33,134]. Flattening of the interventricular septum combined with an enlarged RV chamber, and/or increased RV wall thickness suggests increased RV pressures or volume [134,154]. These presentations arguably take more skill to identify and interpret. If this triad of right heart findings is present, causes of pulmonary arterial hypertension should be considered (e.g., heartworm disease), chronic bronchopulmonary disease (e.g., dynamic airway disease/obstruction, chronic bronchitis, or pulmonary fibrosis), and pulmonary thromboembolism [154]. Although controversial in human medicine, the inferior vena cava (IVC) diameter and collapsibility index are often used in the clinical setting as a means of assessing volume status [33,134]. In dogs and cats, the CVC diameter (CVCd) and caudal vena cava collapsibility index (CVCCI) have been described at several POCUS windows, including the subxiphoid site (Fig. 18.22) [162,163]. This change in size of the CVC between inspiration and expiration is referred to as “CVC collapsibility” and is calculated as the CVCCI. The CVCCI expresses the change (%) in the diameter of the CVC during the respiratory cycle:
18
Point‐of‐Care Ultrasound in Anesthesia
Introduction
Perioperative POCUS
Formal ultrasound
Point‐of‐care ultrasound
Consultative; assessing all organs, anatomy, and structures
Focused on key structures to answer specific clinical questions (often binary choice)
Often performed with nonspecific findings and when multiple or an open‐ended list of differential diagnoses are possible
Often performed when specific signs are present and a high pretest probability of a specific diagnosis (es) is likely (Bayes’ theorem)
Requires years of training
Requires minimal experience
Often takes > 30–60 min
Performed in < 5–10 min
Usually performed by specialists (cardiologists, radiologists)
Often performed by non‐specialists (emergency clinicians, general practitioners)
Patients often stable
Patients often unstable
Patient taken to the machine
Machine taken to the patient
Patient scanned in lateral or dorsal recumbency
Patient scanned in the position they are most comfortable, rarely if ever dorsal recumbency
Fur is typically clipped
Fur is rarely clipped
Gel preferred as the coupling agent
Uses alcohol ± gel as the coupling agent
General applications of POCUS
Target system
Diagnostic applications
Airway
Pleural space and lung ultrasound (PLUS)
Abdominal
Cardiac
Cardiac arrest
Hemodynamics and resuscitation
Eye
Ultrasound‐guided interventions
Locoregional anesthesia
Vascular
Physical examination assistance
Application of P‐POCUS
Equipment and patient preparation
Airway assessment
Endotracheal tube placement
Pleural space and lung ultrasound
Pleural space and lung ultrasound (PLUS) is broadly used to search for: (1) two pleural space pathologies (pneumothorax and pleural effusion); (2) alveolar–interstitial syndromes (AIS) through identification of two abnormal sonographic lung findings (increased B‐lines and lung consolidations; see abnormal PLUS findings below); and (3) to assess for evidence of endobronchial intubation (Table 18.2). Numerous pleura, pleural space, and lung ultrasound protocols have been described in human and veterinary medicine, all of which assess different percentages of pleura and lung surface area transthoracically, with or without inclusion of the subxiphoid window and assessment of the abdominal curtain sign (ACS) (see below for definitions) [36,42,46–51]. With the exception of thoracic FAST, which only assesses a single transthoracic lung site over each hemithorax, all veterinary protocols scan multiple lung regions bilaterally [36,42,46–49]. In human medicine, evidence suggests lung ultrasound protocols that evaluate more lung sites (12–28 sites versus 4–6 sites) have a higher sensitivity and specificity for detecting pathology, but take longer to perform [50,52]. The clinician must therefore balance the risk of missing pathology against the time required to perform sonographic examination. Including the subxiphoid window and ACS may identify pathology in dogs otherwise missed with traditional transthoracic protocols [1,42,46], and assessment of the ACS may allow earlier identification of pleural effusion in humans [51]. The lack of research comparing veterinary protocols makes translation of results between studies, and establishment of cutoff values to detect pleura and lung pathology, challenging. Where applicable, a summation of findings of veterinary studies published has been used to provide guidelines to differentiate normal from abnormal PLUS findings. Fortunately, understanding normal sonographic anatomy and physiology of the pleura, pleural space, and lung, factors that change normal findings, and where to look for pathology, should allow the operator to choose the most appropriate protocol within the clinical setting encountered. As a rule of thumb, conditions resulting in marked dyspnea are likely to be diffuse in nature [48,53], and PLUS should be limited to the minimal number of windows and shortest time possible to identify pathology (Figs. 18.1 and 18.3). Once stable, or in less dyspneic animals, more comprehensive scanning protocols can be used, particularly when very localized or focal pleura and lung pathology is suspected (e.g., isolated lung nodules). Depending on the clinical setting encountered, the authors currently use the PLUS protocol outlined in Fig. 18.3 to scan the dorsal, middle, and ventral thirds of the thorax, focusing on different sites depending on the pleural space and lung pathologies suspected (Video 18.1).
Machine settings for PLUS
Normal PLUS findings
BAT sign
Ultrasound beams cannot transmit beyond a soft tissue–air interface (99% of ultrasound beams are reflected from soft tissue–air/gas interfaces), which is present where parietal pleura (inside lining of the chest wall) contacts the visceral pleura (lung surface) and air‐filled lung [55,56]. If the skin and soft tissue structures of the chest wall are identified, they can be traced distally to where a horizontal concave hyperechoic line is visible just below the ribs and joining the rib shadows (the P‐line, “body of the bat”).
Pleural line
Lung sliding (the glide sign)
Lung sliding is the to‐and‐fro shimmer that is visible at the P‐line as a result of the visceral pleura sliding along the parietal pleura during lung inflation and deflation that occurs with the respiratory cycle [54,57,58] (Video 18.2).
Lung pulse
The lung pulse refers to rhythmic movement of the visceral pleura, which is visible at the P‐line, when cardiac vibrations transmit through motionless lung (as occurs in the lung bypassed during endobronchial intubation, see below) (Video 18.3). It confirms that the parietal and visceral pleura are in apposition to one another. Therefore, the lung pulse has significant clinical implications as it can help rule out pneumothorax (see pneumothorax below) and may suggest endobronchial intubation or an obstructive bronchial foreign body when assessed in conjunction with lung sliding (see endobronchial intubation below) [61]. Note that the lung pulse is typically lost when lung sliding is present (lung sliding dominates the lung pulse), and when the visceral pleura are separated from the parietal pleura (e.g., pneumothorax, pleural effusion, and other space‐occupying pleural space pathology) [58,61]. The lung pulse becomes more difficult to visualize in well‐aerated lung tissue and in lung regions located distal to the heart [57,58]. In healthy dogs and cats, it is possible to identify the lung pulse during the respiratory pause between respirations, particularly when the probe is situated over the thorax and lung in proximity to the heart (Video 18.3).
A‐lines
B‐lines
B‐lines (Fig. 18.5) are a type of sonographic lung surface artifact that occur when there is decreased aerated lung (increased lung density) at the lung periphery (Video 18.4). Depending on the quantity identified at a single probe window and over an entire hemithorax, they may be a normal finding or may be representative of alveolar–interstitial pathology [63,64] (see increased B‐lines below). In healthy individuals, they are believed to result from normal interlobar septa and/or regions with microatelectasis at the lung periphery [65]. They are defined by the following five criteria [54–56,58]: (1) vertical white/hyperechoic projections; (2) originating from the lung surface; (3) extending through the far field without fading; (4) swinging to‐and‐fro with lung sliding; and (5) often obliterating A‐lines if present. If lung is in contact with the chest wall, B‐lines originate at the P‐line. Although 10–50% of healthy cats and dogs have isolated B‐lines identified on PLUS, the majority of sonographically scannable lung surface will not contain B‐lines [42,47,49,66–68]. If present, it is more common to see a single B‐line at one to two locations on either hemithorax, or two B‐lines at a single location when scanning the lung surfaces. Although rare, three B‐lines have been reported at a single sonographic window of healthy cats and dogs [47,66]; however, the presence of three B‐lines is rare and should not occur at multiple lung locations over the same hemithorax [47,66]. Finding more than two positive sites on either hemithorax, regardless of the number of B‐lines at each site, should raise concern for underlying lung pathology [69]. Due to the variation in number of B‐lines reported with different lung protocols in healthy animals, and the small number of studies in healthy dogs and cats reported to date, the clinical context must be considered when deciding if identified B‐lines represent a normal finding or if lung pathology is likely (see increased B‐lines below).
Abdominal curtain sign
During inspiration, the ACS and abdominal contents move caudally, and during expiration, the ACS and abdominal contents move cranially, in a synchronous manner [51,54,70] (Video 18.5). Do not confuse movement of the ACS with lung sliding (Fig. 18.7).
Abnormal PLUS findings
Endobronchial tube placement
Multiple diffuse bilateral B‐lines
Focal /unilateral/localized B‐lines
Interstitial pneumonia or pneumonitis (e.g., lymphocytic interstitial pneumonitis, eosinophilic pneumonia, and drug reactions)
Pulmonary edema – cardiogenic or non‐cardiogenic: heart murmur common with cardiogenic causes, may have history of seizures, chewing an electrical cord, upper airway obstruction, or smoke inhalation
Diffuse parenchymal lung disease (e.g., pulmonary fibrosis)
Acute respiratory distress syndrome: may have spared lung regions
Focal pneumonia/pneumonitis, particularly aspiration: often a history of vomiting
Pulmonary contusions: often a history of trauma
Atelectasis: often a history of prolonged recumbency and/or anesthesia
Pleural disease: interpret B‐lines cautiously when pleural effusion is present as compression atelectasis can result in B‐lines
Neoplasia: often associated with sonographic areas of focal lung consolidation, particularly nodules
Pneumothorax
The absence of all these signs should prompt consideration of a pneumothorax (Video 18.6); however, lung sliding is not always easy to detect, the lung pulse may be lost with aerated lung or may not be visible distal to the heart, and B‐lines and lung consolidation are often not seen in healthy animals. In human medicine the positive predictive value of absent lung sliding for the detection of pneumothorax is 87% in the general population, falls to 56% in the critically ill, and to 27% in patients with respiratory failure [51,59,90,91]. If the history and clinical exam are supportive, thoracocentesis is indicated when lung sliding, lung pulse, and lung pathology are not visible, particularly when assessed at locations where free pleural gas is likely to accumulate (widest part of the thorax with a patient in lateral recumbency, caudodorsal site with a patient in sternal recumbency or standing). If the patient is sufficiently stable, the operator may increase the likelihood of diagnosing pneumothorax by finding the lung point (Video 18.7) and/or an abnormal ACS (Video 18.8) [46,58,88]. The lung point is the location within the thorax where the lung re‐contacts the parietal pleura and creates an intermittent or partial glide sign within a section of the ultrasound image when the patient breathes [58,54]. The lung point is located by moving the probe from an area with no perceived lung sliding toward the hilus, where lung sliding reappears within a region of the ultrasound window [54,58]. In the standing or sternal patient, the probe is often moved from the caudodorsal lung regions toward the elbow. The lung point will not be seen if the lung fails to recontact the chest wall (massive/complete pneumothorax), and has an overall sensitivity of 66%, and a specificity of 100% in human patients [54]. An experimental study of anesthetized dogs demonstrated a sensitivity of 20% and 40% and specificity of 100% for the lung point to diagnose pneumothorax with volumes of 2 and 10 mL/kg of pleural air, respectively [88].
Abnormalities of the ACS identified with pneumothorax include asynchronous and double curtain signs [46]. The asynchronous curtain sign is defined as the cranial movement of the vertical edge artifact (created by free pleural gas versus air‐filled lung overlying the diaphragm and soft tissue structures of the abdomen at the costophrenic recess) with concomitant caudal movement of abdominal contents during the inspiratory phase of the respiratory cycle, with the opposite movements occurring during expiration (Fig. 18.10; Video 18.8). This creates the appearance of the curtain sign and abdominal contents moving toward each other on inspiration and away from each other on expiration, the opposite of what is expected with a normal ACS.
The double curtain sign is defined as two parallel vertical abdominal edge artifacts visible in Figure 18.11 and Video 18.8. Of note, all three types of ACS (normal, double, and asynchronous) have been identified over each hemithorax in dogs with pneumothorax, with normal ACS visible in the ventral third of the thorax. However, these abnormal ACSs have only been described in dogs with mild to moderate degrees of pneumothorax, and extrapolation of these findings to larger‐volume pneumothorax is unknown [46]. The accuracy of an abnormal ACS was not reported, and only six dogs have been investigated, suggesting further research regarding the use/presence of an abnormal ACS to diagnose pneumothorax is needed [46].
Pleural effusion
In human medicine, ultrasound has a sensitivity and specificity of 92–100% and 88–100% for the detection of pleural effusion, respectively, when compared to CT [34]. Similar to pneumothorax, the accuracy of diagnosing pleural effusion using POCUS in veterinary patients is variable and limited by a lack of controlled, prospective studies and small sample sizes [6,45,89]. Recent studies comparing VetBLUE and TFAST protocols to CT correctly identified pleural effusion in 2/3 and 3/6 cases, respectively [87,89]. Pleural effusion appears as hypoechoic accumulations between the thoracic wall, diaphragm, and lung surfaces. Its appearance varies with the cellularity and quantity of the effusion, probe location, patient positioning, and the presence of adhesions. In dogs and cats, pleural effusion is generally identified via transthoracic and subxiphoid windows. Locating the pericardio‐diaphragmatic (PD) window transthoracically helps differentiate pleural effusion from pericardial effusion. The PD window is identified when the probe is positioned such that the heart and ACS or diaphragm are visible within the same sonographic window (Fig. 18.12).
Increased B‐lines
If > 3 B‐lines are visible in a single sonographic window, alveolar interstitial syndrome (AIS) should be considered (Fig. 18.14) (Video 18.9). B‐lines are a sensitive marker of injury and appear concurrent with loss of lung aeration; in some instances, before gas exchange deterioration [92]. B‐lines are an early and sensitive finding of increased lung density [53,92–95]. In cases of pulmonary edema, their presence or absence may guide fluid administration [96,97]. It has been proposed that their appearance warrants fluid caution or restriction, particularly if serial monitoring is performed in patients receiving IV fluids. In contrast, B‐lines disappear with successful treatment of pulmonary edema. Monitoring B‐lines serially for progression or resolution may also help determine if B‐lines are a normal variation or if true pathology is present. Multiple individual to coalescing B‐lines (> 3 per sonographic window, or > 2 positive sites on a hemithorax) should raise suspicion of increased lung density at the lung periphery [47,49,66], often referred to as “wet lung” [47,89]. “Wet lung” is, however, a misnomer; although increased extravascular lung water (EVLW) is the most common cause of increased B‐lines, any process that increases lung density, including atelectasis, fibrosis, collagen, neoplastic cells, and inflammatory cells [54,64,92] can result in increased B‐lines. Given atelectasis occurs in recumbent animals and patients undergoing general anesthesia, knowing the cause of increased B‐lines is important, as therapy differs depending on the cause. History, concurrent diagnostic testing, and mapping the entire PLUS profile by scanning multiple lung regions bilaterally help determine the underlying cause (Table 18.4).
Pulmonary pathology
PLUS profile findings
(often combined with CVC and cardiac findings)
Pneumothorax
Absent lung sliding, lung pulse, B‐lines, consolidation
Lung point (often difficult to sonographically identify)
Abnormal curtain signs (asynchronous/double)
A‐lines visible (parietal pleura reflection)
Pleural effusion
Absent lung sliding
Visible separation of the parietal and visceral pleura (may need to extend depth if large pleural effusion is present)
± B‐lines and consolidations arising from the lung surface, deep to pleural effusion
± “Jellyfish” sign
Pneumonia
Shred signs, translobar signs
Thickened irregular pleura
Subpleural consolidation
Unilateral or bilateral B‐lines
Possible small‐volume pleural effusion
Reduced lung sliding due to consolidations in severe cases
Dynamic air bronchograms
± Static air bronchograms
± Fluid bronchograms
Cardiogenic pulmonary edema and fluid overload
Widespread and bilateral B‐lines
Coalescing B‐lines with increasing severity
Normal lung sliding
Possible bilateral effusion depending on the severity and species (e.g., more common in cats)
*Enlarged left atrium present (LA:Ao often > 2:1)
*CVC may be enlarged if right heart also affected
Note: the absence of B‐lines in the dyspneic patient rules out cardiogenic pulmonary edema – search for other causes of respiratory distress
Acute respiratory distress syndrome (ARDS)
Bilateral or unilateral B‐lines
Normal lung sliding
Shred signs, translobar signs
Mixed pattern with spared regions
Feline asthma
A‐lines visible
Reduced lung sliding with overinflation
± B‐lines and consolidation, particularly if right middle lung lobe affected
Pulmonary embolism (PE)
Normal lung sliding
± Wedge sign
*Right ventricular enlargement and a distended CVC may be seen with massive/submassive PE
Atelectasis
Partial and translobar consolidation
B‐lines often visible
± Static air bronchograms
Absence of dynamic bronchograms
Reduced lung volume – easy to detect with compressive atelectasis secondary to pleural effusion
Lung consolidation
Partial consolidation
Ultrasound appearance
Differential diagnoses
Shred
Appears as if “chunks” removed from the lung creating echo‐poor areas; junction from consolidated lung to aerated lung very irregular, hyperechoic, serrated, and blurred, creating a “shred‐like” appearance similar to a “torn” or shredded piece of paper
Pneumonia
Hemorrhage
Inflammatory‐associated increases in extravascular lung water (e.g., ARDS)
Thromboembolic disease
Neoplasia
Nodule
Smooth hypoechoic or moderately echogenic region; well delineated, often has semicircular, oval, to circular deep boundary
Fungal
Primary or metastatic neoplasia
Abscesses
Wedge
Wedge‐shaped and often well delineated
Pulmonary thromboembolism
Not specific and may result from other pathologies causing shred signs
Air bronchograms
The sonographic appearance of lung consolidation, particularly shred and translobar consolidation, varies depending on whether the smaller airways contain air (air bronchogram, Fig. 18.15, Video 18.10) or are fluid‐filled (fluid bronchogram, Fig. 18.15, Video 18.10) [99,100]. Air bronchograms appear as punctate or linear hyperechoic foci visible between the hyperechoic walls of bronchi [99,100]. Fluid bronchograms can be difficult to differentiate from pulmonary vessels, although some may have air visible in addition to fluid, which helps confirm their presence. Doppler imaging, in conjunction with breath holding, is used in human medicine to differentiate vessels and fluid bronchograms.
Abdominal point‐of‐care ultrasound
Gastric volume
APOCUS window
Clinically driven question(s) to answer
Interpretation
Clinical significance
All five sites
Pneumoperitoneum
Reverberation artifact not originating within the lumen of the gastrointestinal tract, enhanced peritoneal strip sign if free gas contacts the peritoneal lining; be sure to assess the most gravity‐independent sites.
Pneumoperitoneum in patients who have not undergone laparotomy is abnormal and hollow organ rupture should be ruled out. Free gas reverberation artifact often obliterates structures below it.
Free abdominal fluid
Free fluid appears as dark (anechoic or hypoechoic) triangles/sharp angles between organs and structures; be sure to thoroughly evaluate each site considering patient position and where fluid will accumulate.
Obtain fluid samples for in‐house analysis (cytology, refractometer, parameters of sepsis or uroabdomen), which may dictate immediate interventions (surgery, antibiotics, transfusion, etc.). Consider sample submission to reference laboratory (cytology, culture) if indicated.
Subxiphoid
Gallbladder halo sign
Thickening of the normally very thin gallbladder wall that forms a classic striated white, black, white pattern due to edema of the gallbladder wall.
Nonspecific findings but in the unstable patient often seen with anaphylaxis, increased right atrial pressures (heart failure, pericardial effusion, fluid overload), and sepsis.
Caudal vena cava (CVC) size and index
Assess for the cardiac pulse, overall size at end‐expiration, as well as the change in diameter during the respiratory cycle.
A small CVC that fully collapses, or that changes more than 50% is often the result of hypovolemia. A distended CVC that loses the cardiac pulse and changes less than 25% suggests increased right atrial pressures, including fluid overload.
Pericardial effusion
Identified beyond the diaphragm as contained anechoic fluid that curves away from the diaphragm and around the apex of the heart.
Confirms pericardial effusion and differentiates it from pleural effusion.
Pleural effusion
Results in loss of mirror image artifact and appears as uncontained anechoic fluid that tracks along the diaphragm.
Confirms pleural effusion and differentiates it from pericardial effusion, although centesis and fluid analysis are required to determine the fluid type.
Caudal lung pathology
B‐lines and lung consolidation can be detected (if present) in the caudal lung lobes, which are not accessible transthoracically.
See increased B‐lines and consolidations.
Gastric motility
Peristaltic movement of ingesta within the lumen or propagation of a wave along the serosal surface.
Often assessed along with the duodenum and residual gastric contents to detect generalized ileus and decide the need for gastric suctioning and/or prokinetics. The mean number of peristaltic contractions of the stomach and proximal duodenum is 4–5 contractions per minute, although this decreases with fasting.
Cardiac activity during cardiopulmonary resuscitation (CPR)
Assess the cardiac muscle for activity during the 10‐s window when changing compressors – can detect ventricular fibrillations.
Presence or absence of sonographic cardiac activity during CPR is a strong predictor of outcome in human patients.
Umbilical
Splenic lesions/masses
Can detect splenic lesions through changes in echogenicity and structure.
The accuracy of APOCUS to detect splenic masses by non‐specialists has not been assessed, and it is often not possible to sonographically determine if splenic lesions are malignant or benign using ultrasonography.
Right paralumbar
Retroperitoneal injury
Anechoic angles and triangles, or an anechoic rim surrounding the kidney, but not free in the abdominal space.
APOCUS only assesses a small, focused site of the retroperitoneal space surrounding the kidney and pathology can be missed.
Renal pelvic dilation
Often easiest to assess in short‐axis orientation.
Shows good diagnostic accuracy for ureteral obstruction in azotemic cats, although overlap with pyelonephritis exists.
Duodenal motility
Normal motility is 3–5 contractions/min, although this decreases with fasting.
Absence of duodenal and gastric motility, in conjunction with residual gastric volume suggests generalized ileus. The presence of luminal content in the absence of gastrointestinal motility is a stronger indicator of ileus.
Left paralumbar
Retroperitoneal injury
Anechoic angles and triangles, or an anechoic rim surrounding the kidney, but not free in the abdominal space.
APOCUS only assesses a focused site of the retroperitoneal space surrounding the kidney and pathology can be missed.
Renal pelvic dilation
Often easiest to assess in short‐axis orientation.
Shows good diagnostic accuracy for ureteral obstruction in azotemic cats, although overlap with pyelonephritis exists.
Urinary bladder/ pelvic
Urinary bladder volume
Uses the formula for the calculation of the volume of a sphere: measurements in cm and answer in mL: L × W × D × 0.52.
More accurate when the urinary bladder is round, the probe is perpendicular to the urinary bladder at the widest diameter. Measuring the depth in long and short axes should be similar if the widest diameter is obtained in both planes. Although not 100% accurate, a lack of change in bladder volume despite appropriate IV fluid in a well‐hydrated patient could be indicative of anuria or oliguria.
Pyometra
Contained fluid‐filled structures dorsal to the urinary bladder with thicker walls than the large intestine.
Accuracy of APOCUS by non‐specialists has not been studied.
Aortic thromboembolism in cats
Hypoechoic structures within the vessel lumen that can be confirmed with Doppler.
Accuracy of APOCUS by non‐specialists has not been studied.
Urinary bladder volume
Cardiac POCUS
Cardiac POCUS window
Anatomic structures assessed
Clinically driven question(s) to answer/physiology to assess
Clinical interpretation/most common causes
Right parasternal short‐axis views
Mushroom view
Papillary muscles just ventral to the mitral valve: assess left ventricular chamber size, contractility, and wall thickness.
Less commonly, right ventricular size and ventricular septal flattening are assessed. Left ventricular contractility is reported to be 30–50% in dogs and 40–60% in cats.
Consider dilated cardiomyopathy if contractions are weak with an enlarged left atrium; consider volume overload if the heart is hyperkinetic with an enlarged left atrium.
Consider hypertrophic cardiomyopathy if the left atrium is also enlarged; consider pseudohypertrophy if the left atrium is normal to small.
Increased contractility can be seen with iatrogenic volume overload and hypertrophic cardiomyopathy.
Fish mouth view
Mitral valve
Not applicable
Landmark to identify correct mushroom and LA:Ao windows
Mercedes and the whale view
Heart base: left atrial to aortic root ratio (LA:Ao). Normal LA:Ao ratios should generally be between 1–1.5 in cats and dogs (slightly higher in cats). Can fit 2.5 aortic diameters into the left atrium of healthy cats.
Congestive heart failure causing respiratory distress (increased B‐lines) usually has an LA:Ao ≥ 2:1 in cats and dogs. Iatrogenic volume overload can also cause an enlarged left atrium.
Hypovolemia should be suspected when the LA:Ao ≤ 1.
Right parasternal long‐axis view
A subjective RV:LV ratio of > 1 is pathologic.
Subxiphoid view
Pericardial space: effusion appears sonographically as an anechoic space adjacent to cardiac structures.
Sensitivity and specificity for detection of a pericardial effusion are high regardless of patient position.
To avoid missing loculated pericardial effusion, multiple acoustic windows should be assessed.
Pericardial effusion appears as a circular accumulation of fluid adjacent to and surrounding the cardiac chambers, with the pericardial sac often being visualized as a hyperechoic (white) line on the side of the anechoic effusion opposite the cardiac structures. Be sure to extend the depth to visualize the entire heart. If it is unclear whether anechoic fluid accumulation is pericardial or pleural in origin assess the pericardio‐diaphragmatic (PD) windows; parasternal transthoracic windows found on both the right and left side of the thorax (see PLUS).
Pericardial effusion may be detected between the diaphragm and cardiac apex via the subxiphoid view
Cardiac POCUS windows and views
Right parasternal short‐axis views
Right parasternal long‐axis views
Subxiphoid view
Interpretation and integration of cardiac POCUS findings
Left atrial to aortic root ratio (LA:Ao)
Left ventricular lumen size and function
Caudal vena cava assessment at the subxiphoid window
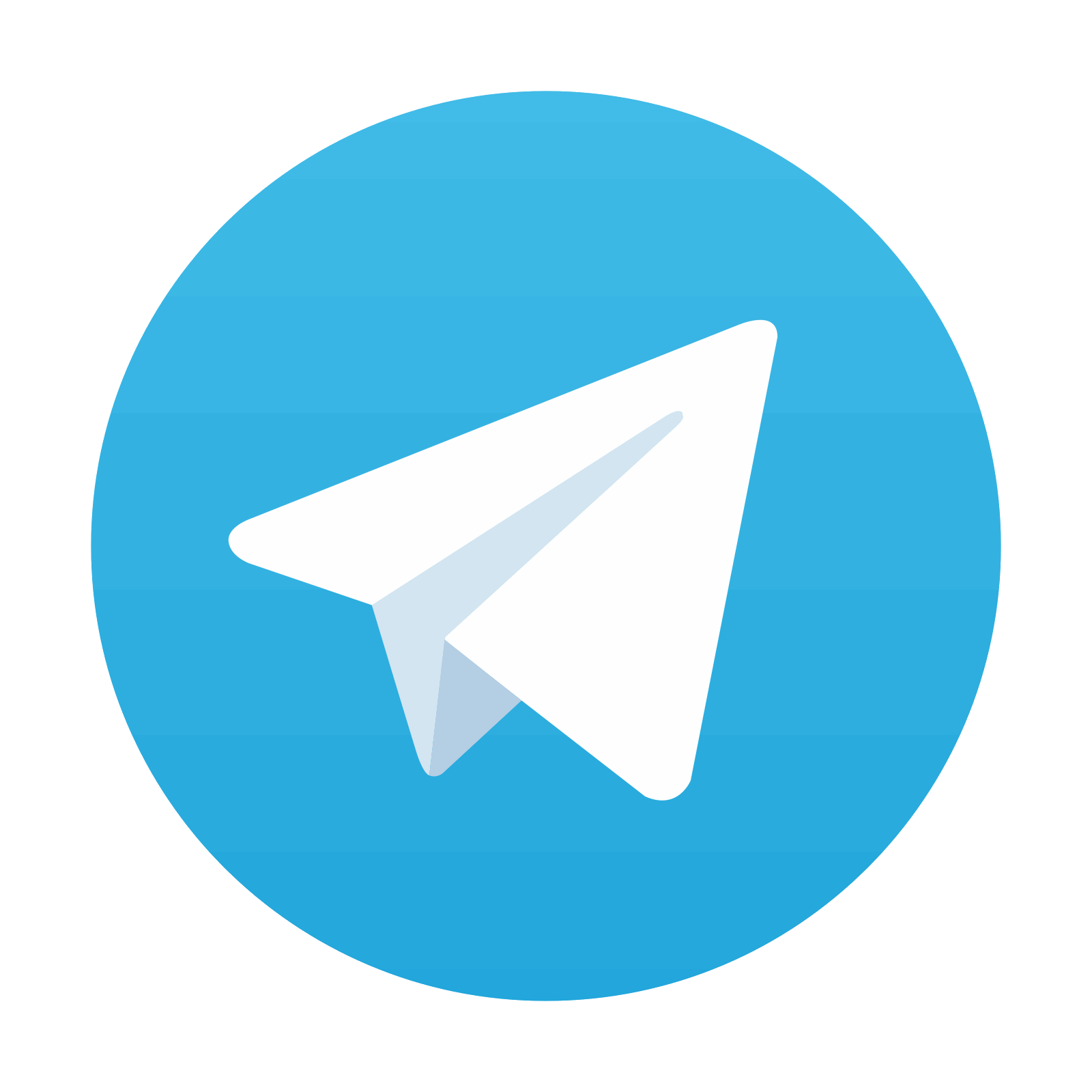
Stay updated, free articles. Join our Telegram channel

Full access? Get Clinical Tree
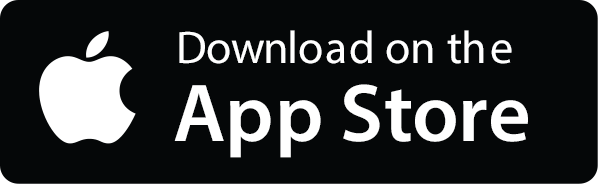
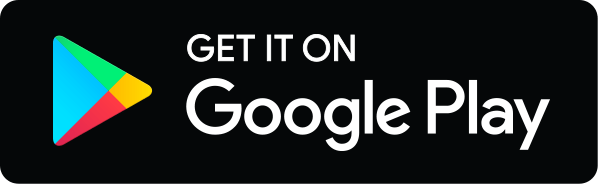