19 Pneumonia Virus of Mice 1Upstate Medical University, Syracuse, USA; Pneumonia virus of mice (PVM) is a member of the Mononegavirales order, family Paramyxoviridae, subfamily pneumovirinae, genus pneumovirus. The 2009 International Committee on Taxonomy of Viruses includes two other agents in this genus, human and bovine respiratory syncytial viruses (hRSV and bRSV). Given the identification of related pneumoviruses from rodents, cows and humans, it is not surprising that related pneumoviruses have also been isolated from the respiratory tract of other mammalian species. Descriptions of caprine (Lehmkuhl et al., 1980), ovine (Eleraky et al., 2003), cameline (Intisar et al., 2010), and canine (Renshaw et al., 2010) pneumoviruses all appear to indicate that pneumoviruses cause respiratory infections, with little or no indication for spread beyond the respiratory tract. PVM was the first of the pneumoviruses to be discovered and described. In 1939, Horsfall and Hahn (1939, 1940) described mice with a transmissible form of pneumonitis. Infection was easily passed from sick to healthy mice using lung suspensions as the inoculum. Recipient mice developed pneumonitis following this inoculum, fulfilling Kochs 3rd and 4th postulates for an infectious agent. The agent was dubbed ‘pneumonia virus of mice’, and more than three decades later, PVM was identified as the first isolated member to be placed in the genus ‘pneumovirus’. Since its discovery, PVM has been reported to infect a broad range of rodents, although data from wild rodents are sparse, so little is known regarding the natural history of infection outside of the laboratory. Results from a small number of field studies, however, indicate that nearly half of wild mice, and approximately one third of wild voles, have been infected with PVM (Kaplan et al., 1980). Following discovery of the virus, seroprevalence studies performed on a broad range of laboratory animals indicated that the virus was widespread in research laboratories. In those early studies, more than 85% of laboratory mice, 65% of guinea pigs, 45% of hamsters and 20% of cotton rats were determined to be serum antibody positive. These observations support the hypothesis that PVM is likely to be ubiquitous in the wild, and that natural infection with the virus confers some degree of immune protection during later exposure. Between the mid-1960s and the early 1980s, studies confirmed that PVM infection was extremely common in laboratory rodents, where symptoms of infection were most often mild (Parker et al., 1966; Gannon and Carthew, 1980). PVM is no longer an endemic pathogen in most rodent research facilities; its decline can be attributed to the introduction of laminar flow hoods, the use of quarantine periods when introducing new rodent or rodent colonies, the use of pathogen-free rodent distributors together with vigilant sentinel surveillance. In laboratories that study rodent virus pathogens, the introduction of micro-isolation cages containing independent HEPA filtration and adherence to strict infection control procedures greatly limits the potential for inadvertent introduction of PVM into other areas of the facility. Early studies on the seroprevalence of PVM suggested that some non-rodent species develop detectable antibody responses against PVM, including laboratory rabbits and nonhuman primates (Horsfall and Curnen 1946; Kagiyama et al., 1986). The early observation that laboratory primates had approximately 25% seroprevalence for specific anti-PVM antibody raised the possibility that PVM might also infect humans. Early indications suggested that humans did indeed have detectable anti-PVM antibody (Horsfall and Curnen, 1946), but it was the work by Pringle and Eglin (1986) that argued that the majority of human adults (75%) were seropositive. More recently, Brock et al. (2012) demonstrated that the anti-PVM antibody was instead a naturally occurring polyreactive IgG, not at all specific to pneumoviruses. Consistent with their findings, no clinical illness has ever been described in response to a PVM exposure in humans, including none among researchers and animal handlers who routinely handle experimentally infected animals under research protocols. Care in describing PVM strains used in laboratory studies has become important since outcomes of studies clearly depend on the strain of virus used. Newly cultured and amplified wild strains of PVM should be similarly described, and comparison of experimental results to historical publications should be undertaken with caution. The prototype virus, PVM strain 15, as archived at the American Type Culture Collection (ATCC) was the first virus to be sequenced. The strain was maintained in tissue culture for nearly 20 years (referred to as PVM strain 15, Warwick) and when used to challenge laboratory mice was found to replicate efficiently but cause little or no clinical illness (Cook et al., 1998). Subsequently, another group obtained PVM strain 15 directly from ATCC (PVM strain 15, ATCC) and showed that it caused significant clinical illness (Krempl and Collins, 2004). A third isolate, designated PVM strain J3666, which has been maintained only through mouse passage, is highly virulent (Thorpe and Easton, 2005). Little is known regarding PVM transmission in the wild, although infection is very common given the findings of the limited number of serosurveillence studies on wild rodents. In the controlled laboratory setting, PVM infection is established via direct inoculation of the respiratory tract, usually by intranasal inoculation under light anaesthesia. In the original studies by Horsfall and Hahn, lung homogenates from the sixth mouse passage were still lethal to 25% of the recipient mice. Inbred mouse strains differ in their susceptibility to PVM infection, with C57BL/6 and SJL mice being more resistant, and DBA/2 and 129/SV more susceptible (Anh et al., 2006). Evidence that PVM was a pneumovirus was first suggested by electron microscopy (Compans et al., 1967; Berthiaume et al., 1974). The enveloped virion is pleiomorphic, with shapes varying from filamentous to spherical, findings that are also typical of the other members of the pneumovirus genus. Unlike hRSV, which is predominantly spherical in morphology, the most typical morphology for PVM virions are the filamentous structures of 100–120 nm in diameter with lengths as long as 3 microns. The significance, if any, of this observation is unclear, and may simply reflect the type of host-cell infected (Berthiaume et al., 1974). PVM is a non-segmented, single-stranded, negative-sense RNA virus of the Paramyxoviridae family. The virus particle consists of an outer lipid bilayer membrane surrounding a helical nucleocapsid. Three proteins are associated with the vial envelope. The F (fusion) protein expands the lipid membrane with its amino terminus located outside of the virion. The G (attachment) protein, by contrast is a type 2 membrane protein with its carboxy-terminus located outside of the virion. Together, the F and G proteins comprise the 10–14 nm spikes that are observed on the virion surface (Fig. 19.1). The small hydrophic protein (SH) is embedded in the membrane. The capsid contains a single copy of the negative-sense non-segmented genomic RNA that is tightly associated with structural proteins to form the helical nucleocapsid structure. The proteins include the nucleocapsid protein (N), the phosphoprotein (P) and the RNA polymerase (L). This nucleocapsid structure is surrounded by the matrix (M) protein, forming a link between the nucleocapsid and the viral envelope. The carboxy-terminus of the F protein and the amino terminus of the G protein interact with the M protein on the internal surface of the lipid envelope. All pneumoviruses have an RNA genome of approximately 15,000 nucleotides (Easton et al., 2004). The complete nucleotide sequences of three PVM strains are available (Krempl et al., 2005; Thorpe and Easton 2005). The negative-sense single-strand RNA genome contains 10 coding regions, NS1, NS2, N, P, M, SH, G, F, M2 and L (Fig. 19.2). Each gene is flanked by a conserved start (AGGAyAArT) and end sequence (tAGTtAnnn(An)) (Chambers et al., 1990). Table 19.1 summarizes the primary known functions of each of the gene products, some of which have been extrapolated from results of basic work on human and bovine RSVs. Fig. 19.1. Schematic of a PVM virion with the nucleocapsid surrounded by M protein. The viral envelope has projections from the F and G proteins. The embedded SH membrane protein is not shown. Fig. 19.2. The PVM genome shown to scale with 10 primary coding sequences and intergenic spacers. Transcript lengths for P2, M2-1 and M2-2 are shown under the genome at loci where transcription is initiated. Table 19.1. PVM strain J3666 genome details, GenBank accession number NC_006579. The non-structural proteins, NS-1 and NS-2, are at the 3′ end of the genome, and thus are transcribed in higher abundance than the other genes. Their function in the virus life cycle appears to be primarily to aid in the evasion of host interferon (IFN) responses (Heinze et al., 2011). The N protein of PVM is believed to play a structural role in the formation of the helical nucleocapsid complex with close association with the viral genomic RNA. Reverse genetics experiments have demonstrated that it is an essential component of the polymerase complex. The P gene product has two open reading frames (ORFs) (Barr et al., 1994), encoding the P protein and several P-like proteins each translated from internal AUG start codons within the P protein ORF. The function(s) of the P-related proteins (including P2) remain unknown, but the phosphoprotein is necessary for genome replication. The product of the L gene is the catalytic component of the RNA-dependent RNA polymerase (Thorpe and Easton, 2005). The mRNA from the M2 gene has two ORFs, encoding two proteins, M2-1 and M2-2. Studies done using the related pneumovirus, RSV, indicate that the M2-1 product is essential for genome replication, playing a role in stabilizing and enhancing the production of full-length RNA. In contrast, reverse genetics experiments using hRSV indicate that expression of M2-2 interferes with virus gene expression (Collins et al., 1995, 1996; Bermingham and Collins, 1999) as it accumulates inside the infected cell. This event is thought to signal a switch from genome replication to virion assembly in preparation for release from the infected cell. The PVM G attachment protein is a type II glycoprotein with its carboxy-terminus projecting from the viral envelope, and from the membrane of the infected epithelial cell (Ling and Pringle, 1989a, 1989b). The protein product is modified extensively post-transcription to include both O- and N-glycosylations. The most substantial genetic difference between the nonpathogenic PVM strain 15 and the highly pathogenic strain J3666 was found in the G protein, where a 35 amino acid membrane anchor was detected only in strain J3666 (Randhawa et al., 1995). The PVM fusion (F) protein is a type I glycoprotein, that like the G protein is easily detected on the surface of infected epithelial cells. The function of the small hydrophobic (SH) gene product is unknown, but reverse genetics experiments performed with hRSV confirm that it is not essential to virus replication. Substantial sequence variation between strains 15 and J3666 indicate a potential role for the SH gene product in pathogenesis, an area that is currently being explored (Thorpe and Easton, 2005). PVM specifically targets the epithelial mucosa of the respiratory tract, including the nose, trachea, bronchi and bronchioles, with minimal involvement of subepithelial tissue. The lung consolidation seen from PVM infection is different from that caused by other respiratory viruses such as influenza, particularly early during the illness when a distinct pattern of bronchiolitis is observed (Fig. 19.3). Intranasal challenge with PVM with as little as 10 plaque forming units/mouse results in robust virus replication with strains 15 and J3666 alike. The main difference between challenges with the two strains is the degree of morbidity. PVM J3666, even at very low inoculum, leads to severe infection. An inoculum of 100 pfu is sufficient to cause 100% mortality in Balb/c mice, and the more resistant C57Black/6 mice will die from a challenge of 300 pfu or more. Mice infected with PVM J3666 become lethargic, anorectic, hunched and ruffled. Weight loss is evident early during infection, as early as day 3 following inoculation. A systematic evaluation of lung function during sub-lethal infection with PVM J3666, as measured by whole body plethysmography, showed that lung function declined in parallel with weight loss and lung virus titre (Bonville et al., 2006). Interestingly, although virus was no longer detectable by day 10 post-inoculation, lung function did not return to baseline until day 28. Clinical illness from PVM strain-15 infection is dependent on the isolate of the virus used. Strain 15 Warwick describes an isolate that has been passed in tissue culture for more than two decades. Challenge with this replication-competent strain 15 virus, at inocula similar to or higher than doses used in severe illness models with the J3666 strain, causes little to no clinical illness (Domachowske et al., 2002). The availability of PVM strains with different virulence and the known differences in susceptibility among various inbred strains of mice have allowed further studies on immunity and pathogenesis, including testing the successful evaluation of mucosal challenge with avirulent PVM as an effective vaccine against later challenge with a lethal inoculum of PVM J3666 (Ellis et al., 2007).
2National Institutes of Health, Bethesda, USA
19.1 Historical Perspective
19.2 Epidemiology
19.3 Transmission and Susceptibility
19.3.1 Virus strains
19.4 Virology
19.4.1 Virion structure
19.4.2 The PVM genome
19.5 Pathogenesis
19.5.1 Clinical features of infection
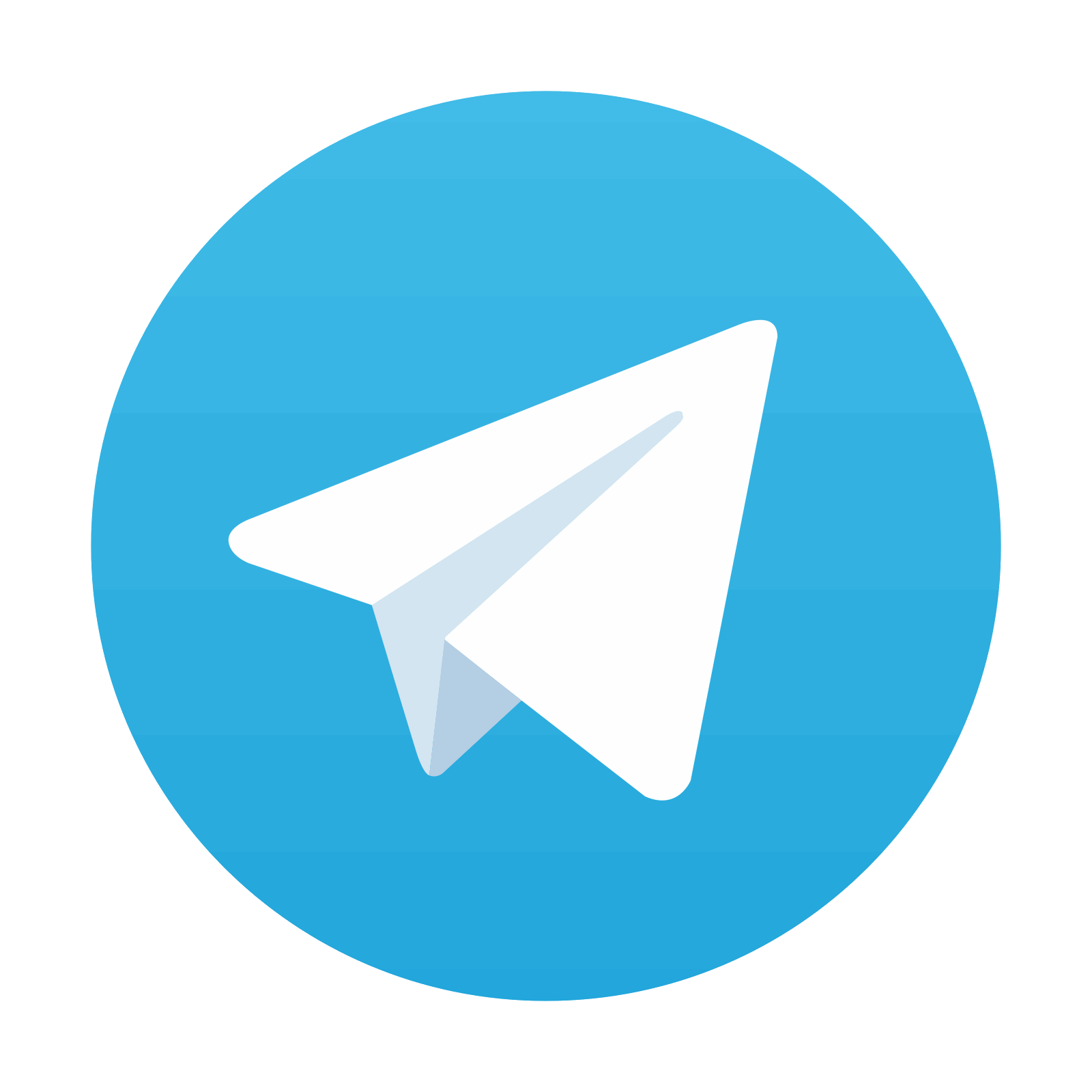
Stay updated, free articles. Join our Telegram channel

Full access? Get Clinical Tree
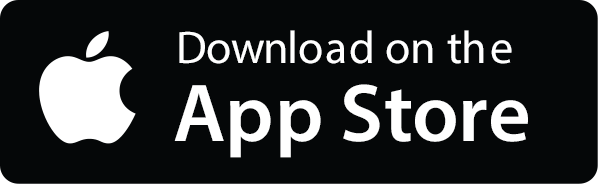
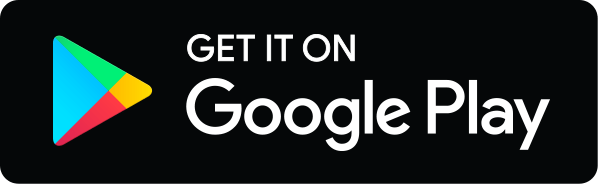