Raquel M. Walton IDEXX Laboratories, Inc., Langhorne, PA, USA Acronyms and abbreviations that appear in this chapter include: NCC, nucleated cell count; RBC, red blood cell; TP, total protein; TPRef, total protein by refractometer; TS, total solids; WBC, white blood cell. Pleural and peritoneal fluids are ultrafiltrates of plasma that reduce friction by lubrication. The constituents of peritoneal fluid are affected by the integrity of the mesothelial lining, changes in vascular permeability and lymphatic flow, plasma oncotic pressure, and capillary hydraulic pressure. Thus, changes in the character of the fluid can be attributed to specific disease processes and may yield information in diagnosis, treatment, and/or prognosis. Pleurocentesis and abdominocentesis are valuable clinical tools whose sensitivity and specificity are highest when all components of fluid evaluation are considered together. Fluid evaluations should include assessment of gross appearance, total protein (TP) concentration, cell counts (or estimation of cellularity), and cytology. If this is not possible, the minimum testing for fluid evaluation should include measurement of TP and measurement (or estimation) of cellularity because effusions are defined by these parameters. An effusion is defined as an increase in the normal volume of peritoneal or pleural fluid, which may or may not have increased protein or cell concentrations. Pleural effusions within North American equids are most often associated with bacterial pleuropneumonia. Other etiologies include neoplasia, penetrating chest wounds, hemorrhage, and cardiac disease. The majority of abdominal effusions are associated with gastrointestinal disorders, especially those causing colic. The main categories of pleural and peritoneal effusions are formulated to provide insight into the general pathophysiological mechanism responsible for an increase in the volume of body cavity fluid. Effusions are caused mainly by transudative, exudative, or hemorrhagic processes (Table 18.1). A fourth category, which is uncommon in horses, is lymphorrhagic effusion caused by leakage of lymph from lymphatic vessels (e.g., chylothorax or chyloabdomen) [1]. Transudates are caused by increased hydraulic pressure or increased hydraulic and decreased oncotic pressure. These effusions have low protein concentrations and nucleated cell counts (NCCs). In horses, normal peritoneal and pleural fluids are distinguished from a transudate only by documenting an increase in fluid volume. The most common causes of transudates are the peracute/acute phase of any lesion causing decreased venous/lymphatic drainage in the portal or pulmonary system (e.g., volvulus/torsion, neoplasia, granuloma), as well as acute uroabdomen, and protein‐losing nephropathies and enteropathies. Diagnosis of uroabdomen in the horse is facilitated by the characteristic presence of calcium carbonate crystals in equine urine, which are present extracellularly and within neutrophils and/or macrophages (Figure 18.1). Calcium carbonate crystals should be distinguished from glove starch crystals, which are commonly seen in cytological preparations (Figure 18.2). Suspicion of uroabdomen should be confirmed by measuring fluid creatinine concentration. Plasma protein permeability of vessels within the hepatic sinuses is higher than that for vessels elsewhere in the peritoneal cavity. Consequently, increases in hydraulic pressure that involve the hepatic sinuses (i.e., sinusoidal or postsinusoidal increases) will produce a high protein transudate with a normal NCC. Congestive heart failure and any lesion that produces postsinusoidal portal hypertension are the most common causes of high protein transudates in the peritoneal cavity. Similarly, the pulmonary vasculature has higher permeability for plasma proteins and high protein transudates may result from increased pulmonary hydraulic pressure. Table 18.1 Classification of pleural and peritoneal effusions. TPRef, total protein by refractometry (g/dL); NCC, nucleated cell count/μL; RBC, red blood cells/μL. Exudates are characterized by increased vascular permeability due to inflammatory mediators and therefore are attributable to inflammation. Inflammation may be caused by infectious or noninfectious processes (see Table 18.1). The absence of microorganisms on cytological examination does not preclude an infectious process. If clinical findings are suspicious of an infectious process, biochemical evaluation of the fluid (for lactate, glucose, and pH) and bacterial culture are warranted. Uniformly bloody specimens or consistently bloody specimens from different sites suggest true body cavity hemorrhage, which may be confirmed via cytology and/or ultrasonographic evaluation of the chest or abdomen. A hemorrhagic effusion is defined by the fluid red blood cell (RBC) count (see Table 18.1); acute hemorrhagic effusions usually have RBC counts >1,000,000/μL and packed cell volumes >3% [1]. Erythrocytes and plasma from hemorrhage will be resorbed by lymphatics so the fluid TP and RBC counts vary with time; in dogs ~65% of RBCs are resorbed within two days of hemorrhage [2]. Because platelets (and coagulation factors) are consumed during hemorrhage, hemorrhagic fluid contains no platelets and does not clot, whereas fluid that contains blood from peripheral or visceral vessels will contain platelets and will clot. In fluids with high RBC counts, the presence of platelets upon cytological evaluation indicates a component of blood contamination since platelets are not present in hemorrhagic effusions. However, the absence of platelets does not preclude peripheral blood contamination if the sample was collected into a nonanticoagulant tube (e.g., red top) and subsequently clotted in vitro. Cytological findings that support an interpretation of hemorrhage include the presence of erythrocytes and/or hemosiderin within macrophages (Figure 18.3). Note that if there is a delay in sample processing (>3–4 hours), RBC phagocytosis can occur in vitro in the transport tube. Conversely, erythrophagocytosis can be absent in samples from true hemorrhage if there has been insufficient time for RBC phagocytosis within the peritoneal cavity (i.e., peracute hemorrhage). In general, it takes several hours for erythrophagocytosis to occur; subsequent RBC breakdown into hemosiderin usually takes at least three days [3]. Thus, cytological interpretations of bloody fluid should be undertaken with knowledge of the type of collection tube used, as well as the amount of time before the sample was processed. Figure 18.1 Calcium carbonate crystals within macrophages in peritoneal fluid. Note the concentric striations characteristic of the crystal (inset). Figure 18.2 Glove talc (starch crystals) in a sample of peritoneal fluid. The crystals are round to rhomboid with a characteristic central divot. Figure 18.3 Hemorrhagic pleural effusion. Macrophages contain a globular green‐black material consistent with hemosiderin (arrows). Note the presence of a binucleated mesothelial cell with hemosiderin (arrowhead). Mesothelial cells with hemosiderin can occasionally be seen in association with chronic hemorrhage. One of the first things to consider when evaluating body cavity fluid is whether the sample is representative. Two common causes of nonrepresentative fluid sampling include blood contamination and enterocentesis (for peritoneal fluid). Blood contamination may occur when a peripheral vein or abdominal organ (usually the spleen) is punctured. Blood contamination becomes apparent during centesis when the initial sample is clear and then becomes bloody or is bloody and then clears. As little as 0.05 mL blood in 1 mL of peritoneal fluid (5% contamination) can result in fluid RBC counts up to 449,000/μL, which is nearly10 times the upper limit of RBC reference values [4, 5]. However, WBC counts and TP concentration remain within reference intervals with up to 17% blood contamination [5]. Inadvertent enterocentesis has a reported frequency rate of 2–5% [4]. The incidence of complications directly related to inadvertent enterocentesis was reported to be 0.5% (four cases in 850 abdominocentesis samples) over a two‐year period at one institution [6]. Enterocentesis may manifest grossly as a green to brown discoloration of peritoneal fluid, but contaminated fluid may also appear grossly normal with contamination only evident upon cytological evaluation. Presence of large ciliated protozoa, plant material, and a mixed bacterial population indicates enterocentesis or intestinal rupture (Figure 18.4). Peritoneal fluid evaluation alone cannot distinguish between enterocentesis and peracute intestinal leakage or perforation. This distinction is best accomplished in the context of the patient’s clinical assessment. Peritoneal fluid volume in health typically ranges from 100 to 300 mL, although it has been estimated that up to 2 L may be present [4]. While the equine peritoneal cavity normally contains a large volume of fluid, abdominal paracentesis typically yields <10 mL of fluid and most commonly 3–5 mL [7]. The pleural cavity often yields a similar volume of normal fluid (2–8 mL), but it may not be possible to retrieve pleural fluid from some healthy horses [8]. The amount of fluid obtained from paracentesis does not necessarily correlate with presence or absence of effusion. In seriously ill horses with effusion confirmed surgically or postmortem, abdominal paracentesis may yield only a small volume [7]. Diagnosis of mild to moderate effusions in equids, especially adults, can be difficult due to the constraints on palpation and large spaces that accommodates even moderate effusion volumes. Ultrasound is often necessary to diagnose effusion. In horses, it is convention to classify abnormal peritoneal and pleural fluid (i.e., increased protein concentration and/or cell count) as an effusion regardless of whether an increased volume is documented. In contrast, a fluid with a normal cell count and protein concentration is only classified as an effusion if there is a confirmed increase in volume. The physical characteristics of body cavity fluid may provide hints as to its cellularity or biochemical composition. Normal body cavity fluid is yellow and transparent. Discoloration of the fluid may reflect hemorrhage (orange to red), enteric rupture (green or brown), bile peritonitis (dark green), infection (yellow‐white), or vascular compromise (orange to reddish brown). Abnormal abdominal fluid color has been used in studies to predict the need for surgical treatment. The sensitivity and specificity of subjectively discolored fluid for predicting the need for surgical intervention vary in different reports: 92% and 74%, 78% and 48%, and 51% and 95%, respectively [9–11]. Weimann et al. [11] used hemoglobin concentration as an objective measurement of discoloration from hemolysis and found that when peritoneal hemoglobin concentration was >0.01 mmol/L (0.02 g/dL), the test was 80% sensitive and 82% specific for selecting surgical treatment. While discolored peritoneal fluid, especially serosanguineous, supports the need for surgical treatment, an interpretation of discolored fluid should only be made when the possibility of enterocentesis or blood contamination has been excluded and should be formulated in the context of physical exam findings. Figure 18.4 Peritoneal fluid with neutrophils containing abundant intracellular bacteria (left panels) and a ciliated protozoal organism (right panel; arrow). The protozoal organism indicates enteric contents are present. Neutrophils with intracellular bacteria suggest intestinal rupture/leakage and peritonitis rather than inadvertent enterocentesis. However, while less likely, these findings could be consistent with enterocentesis if suppurative enteritis were present. The clarity of body cavity fluid in health reflects low cellularity. Increased turbidity is usually reported in terms of cloudy/hazy or opaque, and suggests increased cellularity, presence of plant material, or, rarely, lipid (i.e., chyloabdomen). While turbidity is abnormal, measurement of cell numbers and cytological evaluation of the fluid are necessary to determine its cause. Because body cavity fluid is an ultrafiltrate of plasma, protein concentrations are much lower than in plasma. Protein can be rapidly and accurately measured by hand‐held refractometers. Protein measured by refractometer for body cavity fluids is linearly related to protein measured by biochemical methods and results are accurate to at least 0.6 g/dL [12]. Pleural fluid protein concentration runs slightly higher than for peritoneal fluid (0.2–4.7 g/dL), but the majority of healthy horses have pleural fluid protein concentrations <2.5 g/dL just as for peritoneal fluid [8]. Many studies cite the upper reference value for normal body cavity fluid protein concentration as <2.5 g/dL [4], which is the lowest protein reading on many refractometers. To report values below the lower end of the protein scale, the value measured using either refractive index, refraction, or urine specific gravity (SG) scales can be converted to TP with published conversion tables [13]. When abdominal fluid protein was calculated using conversion tables or measured biochemically, normal values were typically ≤2.0 g/dL in many studies [14–16]. Increases in fluid protein concentration above reference values indicate either increased permeability of the capillaries due to inflammatory mediators or increased hydraulic pressure within hepatic sinusoids or pulmonary vessels, which have higher permeability to protein. An increase in protein concentration due to inflammatory mediators is typically accompanied by an increase in NCCs. Because refractometers measure protein via a total solids (TS)‐based technique, the total dissolved solids in the sample affect light refraction [13]. In addition to protein, TS include electrolytes, glucose, urea, and lipids. While the altered refraction of body fluid is mostly due to protein content, increases in lipid, glucose, or urea content interfere with refractometric protein measurements. However, marked increases in urea or glucose (273 and 649 mg/dL, respectively) are needed to increase protein measurement by 0.4–0.5 g/dL [17]. Unlike glucose and urea, lipid does not freely diffuse into peritoneal fluid from plasma; increased concentrations of lipid in pleural or peritoneal fluid only occur in cases of chylous effusion. Another potential cause of erroneous refractometer readings is the addition of EDTA from K3EDTA anticoagulant tubes. At the standard concentration of EDTA (5 μmol/mL), K3EDTA by itself has minimal effect on the fluid’s refraction (≤0.1 g/dL increase). At higher concentrations of EDTA (10 and 20 μmol/mL), EDTA can increase refractometer total protein (TPRef) by 0.9–1.0 g/dL. Underfilling of EDTA tubes has the effect of increasing the EDTA concentration and will cause spurious increases in the TPRef. Some commercial tubes with K3EDTA anticoagulant may also contain additives to prevent crystallization of the EDTA. Tubes that contain the additive may increase TPRef readings by up to 0.9 g/dL, even when properly filled [18]. It is recommended that TPRef readings should be performed either on fluid collected in serum tubes or in properly filled K3EDTA tubes containing no additives. While sodium heparin anticoagulant has no effect on TPRef [18], heparin has deleterious effects on cellular morphology and is not recommended for samples that will be evaluated cytologically. The term “total solids” has caused much confusion in the reporting of refractometric protein results. TP and TS are not synonymous. Currently, the vast majority of all refractometers incorporate a conversion factor in their design so that the scales report TP and not TS. Contributing to the confusion is the fact that at least one refractometer is named the “TS meter” (AO Corporation) when it is in fact calibrated to report TP [13]. Today, nearly all refractometers report TP and not TS, so comparisons between fluid samples are appropriate. Specific gravity has also been used to estimate protein concentration in equine body cavity fluid. However, use of the refractometer SG scale will yield erroneous results because the SG scale is calibrated specifically for urine. Urea, not protein, is the principal constituent of urine, thus SG calibrated for urine gives falsely high results for body fluids [13]. A caveat applies to low protein fluid (<1.0 g/dL); in this instance, the urine SG scale more accurately estimates true SG because the fluid’s refractive index is more similar to urine [1]. Most biochemical testing of body cavity fluid is performed on peritoneal rather than pleural fluid because of the importance of identifying severely diseased bowel and the ability to assess pulmonary disease via airway evaluation (see Chapter 20). Intestinal ischemia or infarction is one of the most common causes of mortality in colic cases because of subsequent circulatory collapse that can occur. Lactate is the endproduct of anaerobic glycolysis and has been used as a marker for ischemia in colic studies. Normal peritoneal fluid lactate concentrations vary with respect to methodology, but are usually <1.0 mmol/L [16, 19]. Studies of colic cases have shown that peritoneal lactate, TP, NCC, and glucose concentrations were significantly increased compared with peritoneal fluid from clinically normal horses. When the colic cases were subdivided on the basis of strangulating versus nonstrangulating obstructions, peritoneal lactate concentrations were significantly higher in strangulating lesions, with the exception of strangulating small colon obstructions [16, 19]. Increases in fluid lactate, decreases in fluid pH, and abnormal fluid color and/or turbidity were most strongly correlated with ischemic strangulating lesions. Peritoneal fluid lactate concentrations were shown to be more sensitive than blood lactate concentrations in detecting early ischemic lesions. The prognosis becomes progressively poorer for both strangulating and nonstrangulating lesions as peritoneal fluid lactate concentrations increase. One study showed the probability of death in nonstrangulating lesions to be 63% for peritoneal lactate concentrations of 12 mmol/L and 82% for lactate concentrations of 16 mmol/L; the same concentrations of peritoneal fluid lactate had 82% and 92% probabilities of death, respectively, in strangulating lesions [19]. Peritoneal glucose concentrations have been shown to be useful as a relatively rapid test for distinguishing septic from nonseptic exudates [20]. Positive bacterial culture or cytological identification of intracellular bacteria in abdominal fluid is a definitive indicator of abdominal sepsis, but false negatives do occur, especially with antimicrobial therapy. In one study of 36 peritonitis cases, peritoneal fluid glucose concentration and pH were significantly lower in horses with septic versus nonseptic peritonitis [20]. Serum to peritoneal fluid glucose concentration differences >50 mg/dL were most diagnostically useful in confirming sepsis. Mesothelial cells play a significant role in the initiation and resolution of inflammation by secretion of immunomodulatory mediators, which impact on coagulant and fibrinolytic factors such as D‐dimer [21, 22]. D‐dimer concentrations in normal peritoneal fluid are reported to be <88 ng/mL [22, 23], whereas in horses with colic, median peritoneal fluid D‐dimer concentrations ranged from 2023 to 24,301 ng/mL depending upon diagnosis. Amongst colics, median fluid D‐dimer concentrations were highest with enteritis, ischemic lesions, and septic peritonitis (8028, 16,181, and 24,301 ng/mL, respectively) compared with large colon obstructions (2023 ng/mL) [22]. Blood contamination up to 20% does not alter peritoneal fluid D‐dimer concentrations in colic cases [23]. These studies show that peritoneal fluid D‐dimer concentration appears to be a useful indicator of fibrinolytic activity and higher concentrations are correlated with greater disease severity. Cytological evaluation of body cavity fluid can yield important information that may even indicate the effusion’s etiology (e.g., large cell lymphoma, carcinoma, uroabdomen, septic inflammation). Cytology should be interpreted in the context of clinical signs, cell counts, and biochemical evaluation. Erythrocytes are not normally present in body cavity fluid in health, but blood contamination during thoracocentesis may produce RBC counts up to 540,000/μL, although the vast majority of horses have <370,000/μL [8]. Reported counts for paracentesis are lower, ranging from <5000 to <43,200/μL in healthy horses [4, 5, 24]. Visual assessment of discoloration attributable to RBCs was not detected for samples with <40,000 RBCs/μL [25], so typical amounts of blood contamination associated with paracentesis should not change the fluid color. Erythrocyte counts will increase above reference values because of minor blood vessel damage associated with necrosis and/or inflammation; true hemorrhagic effusions are discussed in the section on effusion classifications. Total NCCs for pleural and abdominal fluid are performed with automated blood count analyzers or manually with a hemocytometer. Reference values for pleural and peritoneal fluids are similar. The total NCC for pleural fluid for most horses is <8000/μL [8]. Generally, total NCC reference values in normal peritoneal fluid are reported as <10,000/μL [1, 4], and often <5000/μL [4, 14, 20]. Many equine practitioners and clinical pathologists consider values <5000/μL to be normal, values between 5000–10,000/μL to be ambiguous, and values >10,000/μL as abnormal. Reference values for peritoneal fluid in foals are <1500/μL [15].
18
Pleural, Peritoneal, and Synovial Fluid Analysis
18.1 Pleural and Peritoneal Fluid
18.1.1 Pathogenetic Mechanisms of Body Cavity Effusions
18.1.1.1 Transudates
Classification
TPRef
NCC
RBC
Mechanism
Disorder
Transudate
<2.0
<5 × 103
<4 × 104
Increased hydrostatic and/or decreased oncotic pressure
Nephrotic syndrome
Cirrhosis
Acute uroabdomen
High‐protein
transudate
≥2.0
<5 × 103
<4 × 104
Increased hydrostatic and/or decreased oncotic pressure in postsinusoidal/sinusoidal hepatic or pulmonary vessels
Congestive heart failure
Portal hypertension
Neoplasia
Acute torsion/volvulus
Exudate
>2.0
>5 × 103
>4 × 104 <5 × 105
Inflammation‐mediated increased vascular permeability
Septic:
Bacterial, fungal, or parasitic infection
Nonseptic:
Neoplasia
Ischemic necrosis
Pancreatitis
Bile peritonitis
Chronic uroabomen
Hemorrhage
(acute)
≥2.0
≥ or ≤ 5 × 103
≥1 × 106
Loss of blood from vessels
Trauma
Neoplasia
Hemostatic defects
Uterine artery rupture
18.1.1.2 Exudates
18.1.1.3 Hemorrhagic Effusions
18.1.1.4 Body Cavity Fluid Analysis
18.1.1.5 Volume
18.1.1.6 Color and Clarity
18.1.2 Biochemical Evaluation
18.1.2.1 Fluid Protein Concentration
18.1.2.2 Other Biochemical Tests
18.1.2.2.1 Fluid Lactate, Glucose, and pH
18.1.2.2.2 Fluid D‐dimer Concentration
18.1.3 Cells and Cell Counts
18.1.3.1 Erythrocytes
18.1.3.2 Nucleated Cells
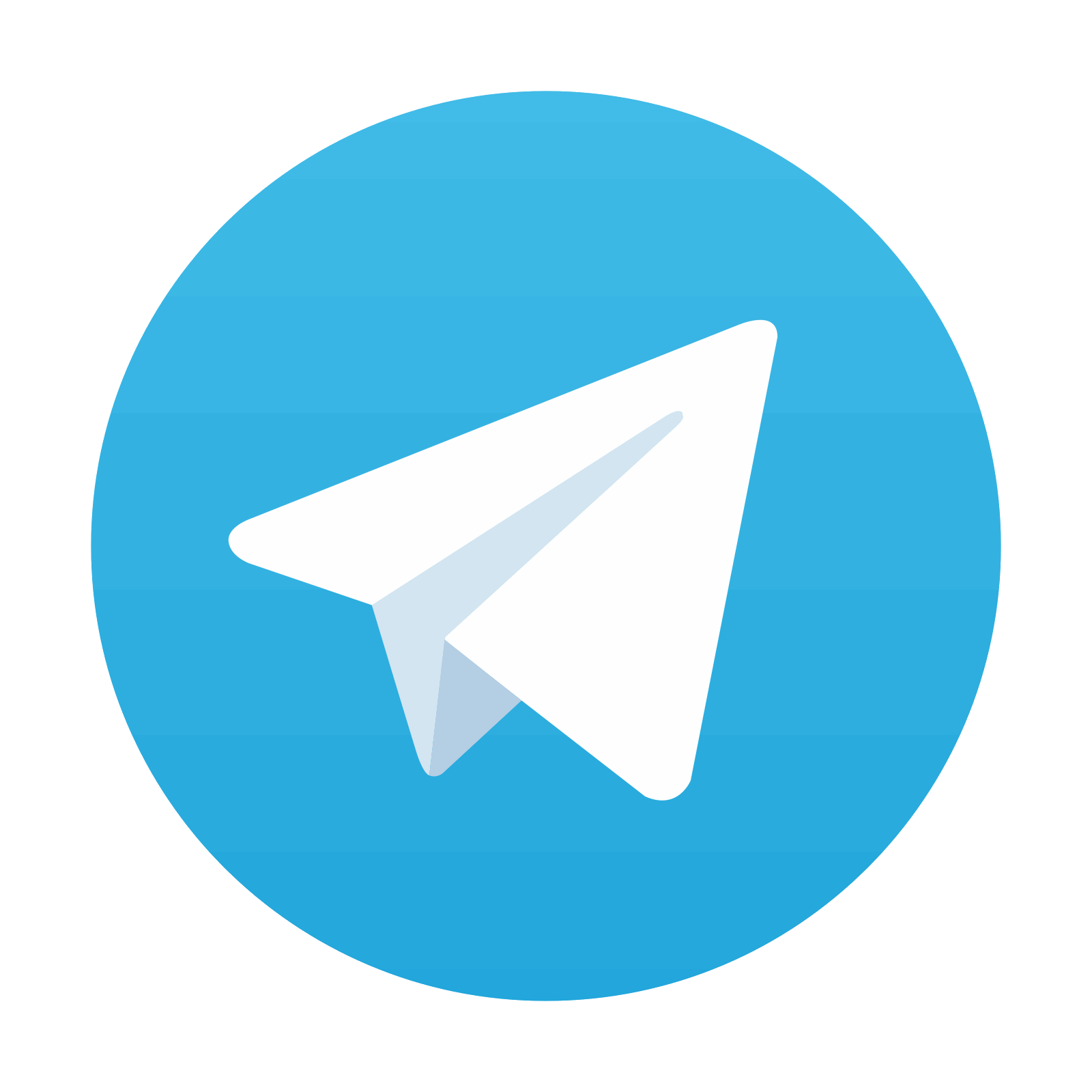
Stay updated, free articles. Join our Telegram channel

Full access? Get Clinical Tree
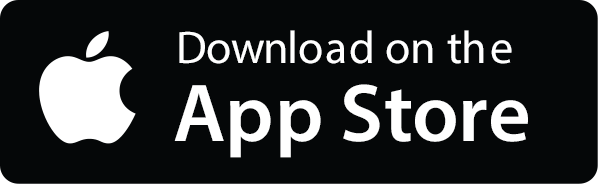
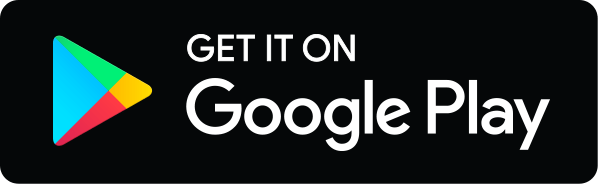