Jennifer G. Adams Hull, Georgia, USA The gastrointestinal tract (GIT) of small animals is a continuous multilayered tube with variations in size, shape, and function for each organ. It includes the oropharynx, esophagus, stomach, small intestine, colon, and rectum. The liver, biliary tract, and exocrine pancreas are also part of the GIT; however, hepatic and pancreatic function and disease are addressed in Chapters 40 and 42, respectively. The intestinal wall has four main layers (from inside to outside): the mucosa, the submucosa, the muscularis with two layers of smooth muscle (an inner circular layer and outer longitudinal layer), and the serosa. The muscular layers of the esophagus are an exception to this basic structure. The entire esophagus of the dog is composed of striated muscle, whereas cats, horses, pigs, and primates have striated muscle in the proximal two‐thirds and smooth muscle in the distal portion, including the esophago–gastric junction. The composition of the specialized epithelium of the mucosal layer varies with each organ, but all organs perform transport, digestive, secretory, and absorptive functions in addition to providing immune protection and surveillance. Controlled rhythmic contractions of the muscular layers mix and transport ingesta throughout the GIT. The external serosal layer is a thin membrane made up of secretory cells that produce and secrete serous fluid. This fluid lubricates the surface, facilitating motility by decreasing friction due to contact with itself and other abdominal organs [1–3]. The design of the control system of the GIT is remarkably large and complex, with a total number of enteric neurons similar to that of the spinal cord [2]. Intestinal function relies on input from both the enteric nervous system (ENS) and the central nervous system (CNS), as well as endocrine and immune support. The ENS has extrinsic and intrinsic components that are supplied with parasympathetic and sympathetic input via the autonomic nervous system (ANS). The intrinsic component includes the myenteric and submucosal plexuses. The myenteric plexus controls intestinal motility and is located between the circular and longitudinal muscle layers. The submucosal plexus coordinates motion of the luminal epithelium and is found between the submucosa and the inner circular muscle layer. Other minor plexuses are also found within the muscle and submucosal layers [1–4]. The extrinsic ENS consists of parasympathetic innervation to the upper and lower GIT via the vagus and pelvic nerves, respectively, and sympathetic innervation via spinal segments T1‐L3. Both afferent and efferent nerve fibers are contained within the same nerves. Preganglionic parasympathetic neurons have long axons that synapse with ganglia of the myenteric or submucosal plexuses within the GIT. Whereas preganglionic sympathetic fibers synapse with ganglia just outside the GIT, the postganglionic sympathetic neurons travel into the GIT itself, synapsing with the intrinsic plexuses and/or directly onto targets in the intestine. Acetylcholine (ACh) is the neurotransmitter released by all preganglionic fibers. Postganglionic parasympathetic neurons may utilize ACh, nitric oxide, adenosine triphosphate, serotonin, or various peptides such as substance P, vasoactive intestinal peptide (VIP), neuropeptide Y (NPY), or gastrin‐releasing peptide (GRP). Postganglionic sympathetic neurons release norepinephrine. In addition to the cholinergic, adrenergic, and peptidergic receptors, many other receptor types are found in the GIT that modify secretory, endocrine, and muscular activity. Receptors for μ‐ and δ‐opioids, several serotonin types, and histamine are just a few. Gastrin, secretin, cholecystokinin, gastric inhibitory peptide, motilin, and ghrelin are hormones with significant secretory and/or motility effects within the GIT [1–7]. Intestinal motility relies on two major patterns of muscular activity, the migrating motor complexes (MMC) seen during fasting, and a digestive pattern that begins when foodstuffs enter the stomach. MMCs are seen in dogs and humans, but motility in cats is controlled by a migrating spike complex pattern that is somewhat weaker than the MMC. The interstitial cells of Cajal, located within the myenteric plexuses, are specialized pacemaker cells that create and maintain MMCs. These are so‐called “slow waves” of depolarization that spread via gap junctions between smooth muscle cells over large sections of intestine but remain below the depolarization threshold for propulsive contractions. This activity provides an effective housekeeping function for the intestine, moving residual fluid, mucus, bacteria, and cellular debris aborally during the interdigestive period. As a food bolus arrives, electrical activity increases, and the digestive pattern begins. Sphincters and sections of intestine in the path of the bolus relax to allow entry as other sections contract in a segmental or propulsive fashion. Ingesta is mixed and moved along the GIT using the circular and longitudinal muscles, respectively. Feedback inhibition also occurs along the tract to “brake” the intestine, providing longer and more thorough contact for digestion. Both the digestive and interdigestive patterns are controlled by the parasympathetic nervous system, with ACh and substance P responsible for contraction and VIP and nitric oxide producing relaxation. Sympathetic input is primarily inhibitory, seen during times of stress, excitement, anxiety, fear, or pain. Numerous neurotransmitters and hormones are involved in the initiation and processing of the digestive phase, affecting motility, secretion, digestion, absorption, and blood flow [1,2,4–7]. In healthy patients, the effects on the GIT of most anesthetic agents are usually short‐lived as normal function returns with the decline of drug levels. Some adjunctive agents may have a longer duration, so decisions about their use should be made with an understanding of the primary GI problem, pre‐existing or concurrent illness or organ dysfunction, and effects of surgery since these may obscure and/or compound the effects of the anesthetic drugs. Prolonged effects into the postoperative period have the potential to affect outcomes. Gastrointestinal disorders during or following anesthesia are not infrequent in veterinary patients. Bloat and regurgitation in ruminants, colic in horses, as well as anorexia, diarrhea, and ileus in rabbits, are common [8–14]. Vomiting, regurgitation, and diarrhea in dogs and cats are not common but do occur perioperatively. Davies et al. [14] identified postoperative regurgitation and/or vomiting (PORV) in 30/244 dogs (12.3%) presenting for non‐elective procedures. GI and neurologic surgery, the American Society of Anesthesiologists (ASA) score of 4, emergency presentation, history of vomiting or regurgitation, use of sevoflurane, and intact gender were significantly associated with PORV. Physiologic abnormalities encountered perioperatively may produce signs of GI dysfunction and can be confused with direct effects of anesthetic drugs. These include dehydration, hypovolemia, hypotension, anemia, protein and electrolyte abnormalities, acid–base derangements, hypoxia, myocardial dysfunction, hypothermia, and changes in ANS tone. Vomiting patients may be alkalotic due to loss of H+ and Cl– but eventually become acidotic with significant volume loss and/or tissue ischemia. As with all patients, preanesthetic evaluation of and treatment for any abnormalities of patient status are necessary to minimize the effects of anesthesia and maximize the potential for a positive outcome. Effects of surgery that may significantly affect perioperative GI function (and often anesthetic management) include tissue manipulation/handling, hemorrhage, and correction of the underlying GI problem (e.g., reperfusion of the stomach following derotation of a gastric dilation and volvulus). The surgeon and anesthetist should work together to minimize the effects of both surgery and anesthesia on perioperative GI function. The effects of anesthetic agents on the GIT include changes in saliva and its production, nausea, vomiting, ileus, regurgitation, gastroesophageal reflux (GER), constipation, reduced secretion or alteration of digestive fluids, and aerophagia (associated with panting). Salivation and panting can lead to fluid loss and contribute to hemoconcentration/dehydration. Although there is considerable overlap of the effects of many drugs (e.g., opioids, α2‐adrenergic receptor agonists, anticholinergics, and inhalants all reduce motility), some effects tend to be more specific (e.g., anticholinergics reduce the volume of saliva but increase viscosity). Ileus can result in tympany, especially if microbial gas production continues at a high rate as seen in ruminants and equine patients. In small animals, some suggest that this could be a contributing factor to the rare occurrence of gastric dilation (GD) or gastric dilation–volvulus, (GDV) seen in dogs postoperatively. Anesthesia is often implicated as a risk factor for the development of GDV, and anecdotal reports are common. GD following sedation [15] or injectable anesthesia [16] are reported, however, published reports of GDV following anesthesia could not be found. Concurrent or chronic illness may complicate the clinical picture. For example, diabetic patients may suffer from gastroparesis and delayed gastric emptying secondary to autonomic neuropathy [17] and may be more likely to develop reflux, esophagitis, and/or vomiting postoperatively. Anemic or hypoproteinemic patients present treatment challenges with fluid therapy, especially if hypotension occurs. Stress of disease, pain, and hospitalization also predispose patients to GI dysfunction, especially anorexia, gastric ulceration, digestive disturbance, and diarrhea [5,7,12]. If anesthetic drugs are the primary cause of GI dysfunction in patients, many of these signs (e.g., ileus, nausea, vomiting) should resolve relatively quickly following cessation of drug administration. If they persist or are severe, further evaluation of the patient is warranted to ensure that another cause is not present (e.g., acid–base or electrolyte abnormalities, hypotension, GI foreign body, GDV, GI ulceration, and internal hemorrhage). The most significant perianesthetic complications associated with GI dysfunction include pulmonary aspiration and/or esophagitis following vomiting, regurgitation or GER, and postoperative ileus (POI). Aspiration may cause pneumonitis, pneumonia, and severe hypoxemia, which can result in cardiac arrest. An episode of general anesthesia is reported to be a very common risk factor for esophagitis and aspiration pneumonia or pneumonitis in small animals. Positioning and duration of anesthesia are reported risk factors for the latter. When severe or unrecognized/untreated, stricture of the esophageal mucosa can develop, which can lead to persistent vomiting, regurgitation, dysphagia, weight loss, and debilitation [17–20]. POI greatly affects patient comfort following surgery and can affect outcomes. A greater incidence of nausea and vomiting, poor wound healing, and delayed oral intake and mobility are seen with persistent ileus. The risk of respiratory and other system complications is increased; longer hospital stays are seen and subsequently, greater financial burden [9,10,20–23]. Nausea and vomiting are common adverse effects of anesthetic agents, especially in human patients. The underlying mechanisms of emesis are complex, as several mechanisms result in vomiting, and two target areas exist in the CNS. A humoral pathway is stimulated by blood‐borne substances that affect the chemoreceptor trigger zone (CTZ), whereas the vomiting center (VC) is activated by a neural pathway. Vomiting occurs when the VC is directly stimulated by one or more neurotransmitters. Histamine, ACh, dopamine, serotonin (5HT and 5HT3), and neurokinin‐1 (NK1 or substance P) are important neurotransmitters in the VC. Dopamine, serotonin, and α2‐adrenergic receptors are found in the CTZ. Located outside the blood–brain barrier, the CTZ is sensitive to low levels of emetic agents in the circulation. Input from the cerebral cortex (anxiety and anticipation), the vestibular apparatus (motion sickness), and local damage to the GIT stimulate the VC. Mucosal irritation and damage or distention of GI tissues produce emesis via the release of serotonin and/or stimulation of vagal afferent receptors [6,24–28]. Vasopressin has also been shown to cause nausea and vomiting, possibly via effects on central opioid receptors [29–31]. While many opioids are associated with a high incidence of vomiting (e.g., morphine and hydromorphone), antiemesis has also been reported. This effect is thought to occur when increased numbers of opioid receptors in the VC are stimulated, as with very lipophilic opioids (fentanyl and congeners) or when given intravenously, especially at higher doses (butorphanol). This may also explain why vomiting is seen primarily after the first dose of opioids and is uncommon following subsequent doses [32,33]. The incidence of vomiting seen with anesthetic drugs is variable and depends on species, drugs used, dosage, route and timing of administration, presence/absence of pain, and concurrent medical problems. Aerophagia and repeated swallowing of blood and saliva (especially following dentistry procedures) can also significantly increase the risk of vomiting during recovery. Most instances of vomiting occur with premedication in dogs and cats. Since vomiting is an active process, it usually does not occur during maintenance of surgical anesthesia but can be seen during light planes or at intubation as the vomiting reflex in dogs and cats is not abolished until Stage 3/Plane II [34]. Inhalant anesthetics are a significant cause of postoperative nausea and vomiting (PONV) in humans [28,35,36], but this incidence is much lower in veterinary species. Vomiting occurs occasionally at induction with propofol [37] and often with etomidate [38] when used alone in dogs, and in recovery following continuous infusion of propofol [39]. A clinical study that compared the incidence of adverse effects in dogs maintained with propofol versus isoflurane after propofol induction reported a very low incidence of vomiting or retching, with 4/91 in the isoflurane group and 1/58 in the propofol group. However, a high incidence of hypersalivation was seen in recovery, affecting just over 20% of both groups. No other signs of nausea were seen, and the dogs did not appear painful. A cause for the salivation was not identified [40]. Another study reported salivation in 7/40 (17.5%) and vomiting during recovery in 6/40 (15%) when propofol was used for induction following four different premedication protocols. Most (12/13) of these were dogs not given acepromazine. The one dog given acepromazine presented for vomiting and had been anesthetized for gastroscopy [41]. Anticholinergics were not used in either study. Although emesis occurred in one dog and one cat during field studies of Alfaxan® [42], it is rarely seen clinically and was not reported in multi‐dose research studies in small animals [43,44]. α2‐Adrenergic receptor agonists and μ‐opioid receptor agonists cause vomiting in dogs and cats [32,45–50]. The incidence of vomiting clinically appears to be more common at higher doses and is generally greater in cats than dogs. Vomiting was not reported with continuous infusions of very low doses of dexmedetomidine or medetomidine in healthy dogs and cats [51–53]. There is minimal difference in the incidence of vomiting between the different α2‐adrenergic receptor agonists; however, a significant difference is seen with different opioids. Vomiting is less frequent with lower dosages and when patients are fasted prior to administration. Morphine, meperidine, and hydromorphone (especially in cats), and to a lesser extent oxymorphone, are associated with the highest risk of vomiting. Vomiting is much less frequent with fentanyl and its derivatives, and is uncommon with methadone, butorphanol, and buprenorphine [32,54,55]. However, two reports investigating the use of methadone in dogs with local analgesia for stifle surgery showed an unexpected incidence of vomiting. Although other mechanisms could not be eliminated, both authors suggested that the analgesia produced by the nerve blocks may have allowed this adverse effect of methadone to predominate, whereas when pain is present, side effects of opioids are less common [56,57]. Acepromazine has a mild antiemetic effect via dopamine antagonism in the CTZ. It decreases vomiting significantly, especially when given prior to opioids in dogs. Its antihistamine effects may also contribute when morphine or meperidine is given concurrently [54,55,58]. The incidence of vomiting/nausea also varies when different opioids are combined with α2‐adrenergic receptor agonists. Eleven of 18 cats vomited after dexmedetomidine with buprenorphine while only one of 19 given dexmedetomidine and butorphanol vomited [59]. Adjunct drugs given during anesthesia may also cause vomiting. Up to 75% of dogs administered a high dose of lidocaine (200 μg/kg/min) as a continuous infusion during sevoflurane anesthesia vomited in recovery. When the dose was reduced (50 μg/kg/min), vomiting did not occur postoperatively [60]. In contrast, decreased nausea and vomiting were seen in humans treated with a lidocaine continuous rate infusion (CRI) for abdominal surgery [61,62]. Metoclopramide is a central dopamine (D2) receptor antagonist that has been used for many years as an antiemetic in dogs. It also has prokinetic effects on the stomach, duodenum, and jejunum, and has some muscarinic and serotonin receptor (5‐HT3 receptor antagonist/5‐HT4 receptor agonist) actions at higher doses [7]. When given subcutaneously 30 min prior to premedication with morphine and dexmedetomidine in dogs, metoclopramide prevented nausea and vomiting and greatly reduced these signs when given concurrently [63]. The serotonin antagonist group (e.g., ondansetron, dolasetron, and palonosetron) and the NK1 receptor antagonist, maropitant, have proven useful to prevent or decrease emesis caused by chemotherapeutic agents [30,64,65], α2‐adrenergic receptor agonists [66,67], and opioids [67–70]. Maropitant affects receptors in both the VC and the CTZ, as well as in the GIT and other organs. It has been shown to reduce perioperative vomiting and nausea very effectively in dogs and cats and is now widely used in clinical practice. There is some evidence it produces inhalant anesthetic‐sparing effects and investigations into potential analgesic and anti‐inflammatory effects are ongoing. The anti‐nausea effect of maropitant is reportedly not as strong as that produced with ondansetron in dogs [30,67,71,72]. Swallow et al. did not find improvement in signs of PONV when given to 24 dogs for ovariectomy although the isoflurane requirement was reduced [73]. However, when given to 40 dogs for ovariohysterectomy, the incidence of pre‐ and postoperative emesis was almost eliminated, fewer patients required rescue analgesia, and improvement in appetite and the quality of recovery was seen; the addition of omeprazole did not provide any further benefit [74]. Hay‐Kraus et al. and Sharun et al. have published reviews of the many actions and uses of maropitant in veterinary medicine [75,76]. Dexamethasone is an effective antiemetic in humans for the prophylactic treatment of PONV [28,36,77]. It decreases the frequency of vomiting in cats induced by xylazine, but only at high doses [78]. The anti‐inflammatory, anti‐immune, and adverse endocrine and GI effects probably preclude its routine use as a prophylactic antiemetic in veterinary patients. Benzodiazepines, phenothiazines, and butyrophenones have been shown to have antiemetic effects in humans. Formerly, droperidol was used frequently in small animals as a sedative, especially in combination with fentanyl (Innovar Vet®). Droperidol is considered a highly effective antiemetic in humans; however, the risk of cardiac arrhythmias precluded its use for a time [28,36]. It has since been determined that the cardiovascular effects are minimized when lower doses are used [28]; however, other adverse effects such as sedation, excitement, extrapyramidal effects, and the availability of better alternatives preclude the routine use of butyrophenones as antiemetics in veterinary patients. Non‐pharmacologic interventions for PONV in humans include acupuncture or acupressure [28,36]. Needling at acupuncture point Pericardium‐6 (PC6) decreased the incidence of nausea and vomiting with hydromorphone in dogs [79], while electroacupuncture at PC6 was shown to prevent emesis induced by vasopressin in dogs. Pretreatment with naloxone prevented antiemesis, suggesting that opioid pathways may be involved [31]. Gastroesophageal reflux disease (GERD) is a common syndrome in humans. It is reported to occur in 5–20% of adults but may be as high as 40% in some areas [80]. The proposed mechanisms of reflux include abnormally low lower esophageal sphincter (LES) pressure (LESP), increased frequency and duration of transient lower esophageal sphincter relaxations (TLSRs), and alteration of the anti‐reflux barrier. TLSRs are normal events that occur with swallowing and vent gas formed in the stomach. They are most often seen following ingestion of food. TLSRs do not occur during general anesthesia in dogs and humans or during deep sleep in people. Relaxation of the diaphragmatic crus and the LES must be present for normal TLSRs to occur [80–85]. Although the specific mechanism of anesthetic‐induced GER is unclear, reflux occurs when intragastric pressure exceeds or equals the LESP, and the barrier pressure normally present between the two areas is lost [80–85]. Intra‐abdominal and intrathoracic pressure also contribute to LESP and the incidence of GER. The anti‐reflux barrier is created by the anatomical arrangement of the LES, the right crus of the diaphragm, and the oblique angle at the junction of the esophagus and the cardia. The LES is actually located above and below the hiatus of the diaphragm and is several centimeters in length. Functionally, it is a high‐pressure zone just above the cardia created by a variable arrangement of muscle layers in the distal esophagus in combination with the angle of the crura of the diaphragm. To prevent retrograde flow of gastric contents, the LES remains in a tonically contracted state until stimulated to relax via relaxation of the upper esophageal sphincter and/or waves of peristaltic contractions higher in the esophagus [80–85]. Table 41.1 Neurotransmitters and hormones that affect lower esophageal sphincter (LES) tone. Source: Compiled from Washabau [1]; Hershcovici et al. [83]. Although primarily controlled by the parasympathetic nervous system, numerous neurotransmitters and hormones are involved in regulation of LES tone (Table 41.1) [1,83]. Clinical GERD is less common in dogs and cats than in humans but has been identified in both species [86–89]. Brachycephalic dog breeds are predisposed to GER [90–96]. Functional and anatomical abnormalities of the esophagus and LES, and inflammatory lesions of the upper GIT have been identified in brachycephalic breeds [19,91,92]. Eivers et al. evaluated 26 dogs for esophageal dysfunction using videofluoroscopy and identified esophageal dysmotility, GER, and hiatal hernia; 77% were brachycephalic [92]. GERD is likely underdiagnosed since mild cases may not result in clinical signs [86,88,89]. “Silent reflux” events are known to occur in people without consequence [80]. These have recently been identified in healthy dogs using nuclear scintigraphy [97]. Numerous anesthetic drugs and adjunct medications, including sedatives, analgesics, induction agents, inhalants, and anticholinergics, have been shown to decrease LESP in humans, dogs, and cats, predisposing anesthetized patients to GER (Table 41.2) [82,85,98–106]. Perioperative GER is common; incidence varies from 0 to 71% of cases. Numerous studies have investigated the occurrence of GER under many conditions and with many anesthetic and/or adjunct agents (Table 41.3) [107–127]. GER is more frequent with intra‐abdominal and orthopedic procedures in dogs and may be more common in dogs than cats [12,101,104,116–118]. GER occurred in 9/27 cats anesthetized for dental procedures [120], but was not more common during intra‐abdominal procedures in 44 cats compared to 30 controls [121]. Vomiting associated with premedication prior to anesthesia was not associated with increased GER in dogs [123–125]; however, the occurrence of GER under anesthesia is variable and unpredictable. In spite of numerous investigations, the mechanism of GER seen with general anesthesia has not been proven. Loss of the barrier pressure (gastric pressure – LESP) between the distal esophagus and the stomach must occur [80–85], but it is not clear what specifically triggers this phenomenon. Most cases of anesthesia‐related GER in dogs and cats develop fairly soon following induction, usually before 30 min [116,117]. Choice of premedication plays a role in some cases [126] but not in others [127]. GER is frequent with opioids [112,127] especially μ‐opioid receptor agonists such as morphine and hydromorphone [116,123,126]. Dosage may also be a factor, as higher doses of morphine cause more GER than lower doses [123]. Induction with propofol has resulted in greater incidence of GER in dogs [107,119] but not in cats [121]. Pharyngeal stimulation may be a factor as it decreases pressure at the LES. Minor pharyngeal stimulation elicited relaxation of the LES more than 50% of the time in opossums anesthetized with pentobarbital [128], while subthreshold stimulus of the pharynx produced longitudinal esophageal muscle contraction and LES relaxation in humans [129]. Interestingly, GER was seen in 50% of kittens managed with an LMA versus 14% with an endotracheal tube [130]. Perhaps the combination of pharyngeal stimulation at induction combined with the effects of multiple anesthetic drugs during the transition into deeper levels of anesthesia is significant; however, this does not explain episodes of reflux that occur later during anesthesia or in those without endotracheal intubation [121]. GER is seen with virtually all inhalants; no difference was seen in dogs anesthetized with halothane, isoflurane, or sevoflurane [125]. Numerous factors are certainly involved in the mechanism of GER; specific triggers may be multiple and vary with patients, drugs used, procedures, ASA status, and other circumstances. Table 41.2 Effects of various anesthetics, analgesics, sedatives, adjunct medications, and miscellaneous factors on lower esophageal sphincter pressure (LESP) reported in dogs, cats, and/or humans. Source: Compiled from Cotton and Smith [85]; Ogunnaike and Whitten [98]; Open Anesthesia [99]; Strombeck and Harrold [100]; Hall et al. [101]; Waterman and Hashim [102]; van der Hoeven et al. [103]; Hashim and Waterman [104]; Hashim and Waterman [105]; Hashim et al. [106]. NMBA, neuromuscular blocking agent. a Conflicting results concerning nitrous oxide. b Reported with Saffan®, not Alfaxan®. c Ketamine decreased LESP compared with awake values, but it remained much higher compared to other drugs. Table 41.3 Anesthetic protocols, procedures, and other factors reported to impact the incidence of regurgitation or gastroesophageal reflex (GER). Source: Compiled from Savvas et al. [107]; Savvas et al. [108]; Savvas et al. [109]; Galatos and Raptopoulos [110]; Savvas and Raptopoulos [111]; Wilson and Evans [112]; Tsompanidou et al. [113]; Viskjer and Sjöström [114]; Anagnostou et al. [115]; Wilson [116]; Galatos and Raptopoulos [117]; Wilson et al. [118]; Raptopoulos and Galatos [119]; Garcia et al. [120]; Sideri et al. [121]; Galatos et al. [122]; Wilson et al. [123]; Wilson et al. [124]; Wilson et al. [125]; Costa et al. [126]; Flouraki et al. [127]. LMA, laryngeal mask airway; ET, endotracheal; NSAID, non‐steroidal anti‐inflammatory drug. In spite of the high frequency of GER detected using pH or impedance monitoring in dogs and cats, the incidence of visible regurgitation (i.e., oral drainage of fluid) is much lower. In a large study of 4257 dogs at a referral practice over a 2‐year period, regurgitation was visually confirmed in only 27 or 0.63% of cases, with large dogs and orthopedic procedures at highest risk [131]. Regurgitation was observed in 75 or 1.3% of 5736 dogs in another large study [132], where greater risk was seen with an ASA status ≥ 3, abdominal or imaging procedures, long anesthetic duration, and larger size. Dogs anesthetized for all types of procedures regurgitated less than 1% of the time, even though GER was identified in 16.3 and 17.4% of cases in two other reports [110,117]. During orthopedic procedures in dogs following administration of various anesthetics agents, regurgitation occurred in 0–17% of the cases, while GER was identified in 25–71% of the cases [114,118,123–125]. In several studies involving cats, regurgitation was reported in 0, 2%, 0, and 0 cases with an incidence of GER of 18, 16, 33.3, and 50% [120–122,130]. Prevention of GER in anesthetized dogs has been investigated with H2 receptor antagonists, proton pump inhibitors (PPIs), and prokinetic drugs. Although H2 receptor antagonists increase gastric pH in dogs, they are not as effective at maintaining increased pH as PPIs; and even with PPIs, multiple doses are required [133–137]. Omeprazole given preoperatively to 47 dogs decreased the frequency of acidic reflux (4 with omeprazole versus 13 without); however, as lower esophageal pH was the only parameter measured, some reflux events may have been missed [136]. When two doses of omeprazole were given preoperatively to dogs, the incidence of GER was not different, but a much higher pH of the reflux, 6.3 ± 1.5 versus 4.1 ± 1.5 versus 3.8 ± 1.1, was seen [137]. When dogs were given maropitant, omeprazole, or both, no difference was seen in the occurrence of GER or the pH of the reflux [138]. The incidence of GER was significantly lower in cats given two doses of omeprazole, but some treated cats still had multiple episodes of reflux [120]. A study in 61 dogs using both impedance and pH monitors found that esomeprazole alone increased pH, and the addition of cisapride decreased the incidence of reflux significantly from 8/21 with placebo to 2/18 in the combination group [139]. When an infusion of cisapride was given preoperatively to dogs anesthetized for surgical treatment of laryngeal paralysis fewer cases of aspiration pneumonia were seen, which is thought to be caused by GER [140]. Metoclopramide alone decreased the incidence of GER, but did not completely prevent it in dogs. This effect was seen only when given via continuous infusion at a high dose [141]. Intravenous metoclopramide, and/or ranitidine prior to anesthesia followed by a metoclopramide infusion, did not decrease the incidence of GER in dogs anesthetized for ovariohysterectomy [142]. Maropitant given prior to induction in combination with an infusion of metoclopramide for 24 h did not prevent clinical signs of GER in the postoperative period [143]. The effect of several palliative treatments for GER has been examined. Esophageal lavage in 32/48 dogs with reflux pH < 4 using water, or two bicarbonate solutions increased the pH to > 4 in 78.6% with water and 100% with bicarbonate. Interestingly, the pH improved in 16/48 dogs without treatment [144]. Removal of reflux fluid from the esophagus via suctioning in 10 dogs did not significantly change the esophageal pH, lavage with tap water increased the pH to > 4.0, and the infusion of a small volume of sodium bicarbonate increased the pH to > 6.0, an effect that lasted from 1.5 to 3 h [145]. Repeated suctioning and tap water lavage followed by bicarbonate therapy were also evaluated in dogs [146]. Lavage was determined to be unnecessary as the final esophageal pH did not differ once bicarbonate was applied. Bicarbonate therapy may not prevent further reflux/regurgitation and may not help with non‐acidic reflux. However, it could be beneficial with acidic reflux to reduce the risk or severity of esophagitis. Suctioning and lavage can be useful in cases with a large volume of regurgitation. Several authors have examined the effects of fasting duration and types of food on the incidence of GER, gastric volume, and pH in dogs, with somewhat variable results. Some have shown that a short fast utilizing small volumes of canned food results in decreased incidence of GER [108–111], higher gastric pH, and lower gastric volume [106]. Others have not seen a difference with shorter fasting times [112,113] and another found a greater incidence of GER and regurgitation with a shorter fast and a higher pH with a longer fasting time [114]. Actual fasting times are often much longer than recommended in humans and animals. In people, fasting is associated with hunger, thirst, decreased satisfaction, and sometimes nausea, vomiting, or dehydration postoperatively. Because research has not shown a decreased incidence of aspiration pneumonia with long fasting times, the guidelines used by the ASA were revised in 2017 for healthy patients [147]. The minimum fasting guidelines for adults and children with a low risk of aspiration include: (1) clear liquids, 2 h; (2) breast milk, 2 h; (3) milk‐type liquids, 6 h; and (4) a light meal 6 h prior to anesthesia. Eight hours or more is recommended if meat or a fatty meal is ingested [147]. In 2023, further revision included the recommendation that healthy adults should drink a carbohydrate‐type clear liquid up to 2 h prior to the procedure, and clear liquids in children be allowed/encouraged up to 2 h prior to anesthesia as well [148]. In 2020, the American Animal Hospital Association (AAHA) published revised guidelines on fasting in a similar fashion. Water is not withheld, and food is allowed up to 4–6 h prior to the procedure in healthy adults. Very young (< 8 weeks) or small (< 2 kg) patients should have food withheld for no more than 1–2 h [149]. Prevention of GER is difficult; however, vigilant monitoring to identify when it has occurred and appropriate intervention will minimize complications. General management recommendations to treat GER in the perianesthetic period are summarized in Box 41.1. Esophagitis can occur when the esophageal mucosa is exposed to caustic substances for prolonged periods and/or when esophageal defense mechanisms are impaired or overwhelmed. Esophageal defense mechanisms include a superficial mucus/bicarbonate barrier on the mucosal surface, tight junctions between epithelial cells, and an intracellular and interstitial buffering capacity that is dependent on blood flow. Lack of clearance of esophageal contents via intermittent swallowing is also a factor in anesthetized patients. Saliva is often decreased under anesthesia and, of course, anesthetized patients cannot swallow. Saliva provides dilution, bicarbonate to neutralize acid, and volume to flush the lumen of the esophagus. Resistance to 30 min of exposure to acid has been demonstrated; however, pepsin, trypsin, bile salts, and possibly other irritating or caustic substances may be as important as acids in causing esophageal damage. Exposure to acidic reflux also induces release of inflammatory mediators that contact and damage nerve endings resulting in decreased LESP, which predisposes to further GER and perpetuates the cycle [18,19,81,150]. Esophagitis and subsequent esophageal stricture are secondary illnesses, caused by damage from exposure to gastric/intestinal fluids. GER, vomiting and/or regurgitation, ingestion of foreign bodies or caustic substances (including some medications such as doxycycline and clindamycin), motility disorders, congenital or anatomic abnormalities (e.g., hiatal hernia and megaesophagus), trauma, neoplasia, infection, neuromuscular disease, and any cause of altered mentation are contributing factors. Hypergastrinemia with resultant hyperacidity, delayed gastric emptying due to pyloric outflow obstruction, pancreatitis, or diabetes are also reported causes [18,19, 87,88,151–156]. An inflammatory cause of eosinophilic esophagitis known to occur in people has been reported in a dog and a kitten [157,158]. Cats with chronic gingivostomatitis have also been found to have concurrent esophagitis [159]. A history of recent general anesthesia is very common in dogs and cats diagnosed with esophagitis, with perianesthetic GER as the most likely underlying cause. Retrospective studies have examined the incidence, risk factors, and outcome of esophagitis/stricture [87,89,151–158]. Leib et al. [89] identified 18 of 28 (64%), Adamama‐Moraitou et al. [160] found 13 of 20 (65%), and Kushner and Shofer [161] reported 25 of 30 (83%) patients with esophageal stricture diagnosed soon following general anesthesia. Overall mortality in these reports was 21, 30, and 30%, respectively. Greater risk of esophagitis was seen in patients anesthetized for intra‐abdominal procedures, especially ovariohysterectomy [160]. Manipulation of abdominal structures is presumed to increase gastric pressure, while LESP is decreased by anesthetic agents. Progesterone levels in intact females were thought to contribute to decreased LESP; however, a study of barrier pressure and GER in female dogs anesthetized during four different phases of their reproductive cycle showed no effect of hormone levels on barrier pressure, the incidence of GER, or esophagitis [162]. In contrast to dogs, a study of esophageal disease in cats over a 7.5‐year period found anesthesia to be involved in only one of 33 cases [154]. This may not reflect the true incidence in cats since signs may be readily overlooked. When all cases presented for anesthesia over a 10‐year period were examined retrospectively, a very low incidence of postanesthetic esophageal dysfunction was reported. Twenty‐five patients with esophageal stricture following anesthesia were identified at one institution (0.1% of 23,295 cases) [161], 3 cases of esophagitis and 10 cases of esophageal stricture (13 total cases, 0.07% of caseload) occurred over 8 years at another [163]. Mortality associated with the esophageal complications was 30 and 23%, respectively. Vomiting and regurgitation occurred postoperatively in most or all of these patients. Four of the 13 dogs also had aspiration pneumonia. Weight loss, debilitation, and chronic cough were seen in some dogs (Fig. 41.1). A case report of two young cats that had exhibited vomiting and/or regurgitation, and dysphagia soon after elective general anesthesia developed rupture of the esophagus and were euthanized, one at 6 days, the other at 14 days postoperatively [164]. Figure 41.1 Patients with esophageal stricture may be debilitated due to dysphagia and aspiration pneumonia. Source: Dr. Jennifer Adams, with permission. Although esophageal dysfunction following anesthesia is uncommon overall, the history of many cases includes an episode of general anesthesia. This is a devastating complication with a high mortality. Any patient that exhibits vomiting, regurgitation, nausea, salivation, dysphagia, and/or anorexia postoperatively should be monitored closely. When signs persist, these patients should be thoroughly evaluated for esophageal disease. Aspiration of GI contents can occur perioperatively following GER, vomiting, and/or regurgitation. It can also occur during heavy sedation that impairs protective airway reflexes. Respiratory complications following aspiration include hypoventilation and/or hypoxemia, pneumonitis, bacterial pneumonia, and sometimes cardiac arrest. The extent of airway pathology depends on the volume and type of fluid aspirated. Three phases of damage have been identified. Stage 1 is immediate and is caused by direct damage to the epithelium. This injury to the lung is actually like a chemical burn due to the effect of gastric fluid on tissue, with greater damage seen with low pH, even if the volume is small. Varying degrees of atelectasis, decreased compliance, ventilation–perfusion mismatch, and decreased oxygenation develop at this point. Stage 2 is an inflammatory reaction that follows within 4–6 h, causing pneumonitis. If not severe, this lesion may resolve. However, with more significant injury, alveolar macrophages and Type II pneumocytes release cytokines that stimulate the influx of neutrophils, which release other inflammatory mediators, causing acute lung injury even without bacterial involvement. Surfactant is also altered by inflammation, contributing to impairment of gas exchange. Stage 3 is seen when bacteria invade damaged tissue, producing aspiration pneumonia (AP) [19,165–167]. Incidents of aspiration are not commonly witnessed, but early recognition and intervention are paramount to limiting the severity of pneumonitis/pneumonia. Signs of aspiration range from “silent” with no apparent abnormalities to obvious airway obstruction following visible regurgitation of gastric contents. Increased risk is seen with anesthesia, especially in patients with laryngeal and esophageal disorders, vomiting, neurologic disease, and GER [19,20,164–174]. People with AP more often have altered mental status, dysphagia, and esophageal disorders as common pre‐existing conditions [167]. Brachycephalic breeds are predisposed to aspiration due to an increased incidence of GER, vomiting, and regurgitation that is common in these breeds. The underlying cause is likely an increased negative pressure that is caused by the upper airway obstruction secondary to their brachycephalic anatomy [19,93,96,167,175]. Clinical signs in anesthetized patients include unexplained oxygen desaturation, hypercarbia, tachypnea, dyspnea or irregular respiratory patterns, auscultable abnormalities, and blanching of mucous membranes. Oxygen (100%) should be administered and the patient immediately positioned with the head down for drainage of aspirated material; removal should be instituted as soon as possible. Suction of the airways can be used for liquid aspiration, but bronchoscopy is often required when particulate matter is present. Bronchodilator therapy and mechanical ventilation with positive end‐expiratory pressure (PEEP) may be needed to improve oxygenation. Prophylactic antibiotics are not usually recommended for pneumonitis cases owing to the potential for emergence of resistant bacteria [19,165,166,170]. Since bacterial colonization occurs later in the process, in humans antibiotics are recommended only in those with confirmed infection, especially in otherwise healthy patients. However, patients with GI obstruction or on chronic antacid therapy may be an exception owing to the potential for enteric organisms to be found in reflux fluid [165,166,170]. It can be difficult to document the presence of infection quickly in veterinary patients, so antibiotic therapy is often instituted early in the course of treatment [167,168]. A very large multicenter study evaluated the anesthesia caseload at six veterinary institutions over an 11‐year period to determine the incidence and risk factors for AP following anesthesia or sedation in dogs [20]. The criteria included radiographic or necropsy evidence of AP within 72 h of sedation or general anesthesia. Multivariate analysis of numerous patients, procedures, and anesthetic factors was performed for 240 cases along with 488 controls. The incidence varied significantly between institutions, from 0.04–0.26%, with an overall incidence of 0.17%. Of 12 dogs who were given sedation only, three developed AP. Multivariate analysis revealed the following to be associated with anesthetic‐related AP: (1) patient factors including megaesophagus (ME) and pre‐existing respiratory or neurologic disease; (2) procedural factors including upper airway surgery, endoscopy, thoracotomy, laparotomy, and neurosurgery; and (3) anesthetic events, including regurgitation during or after anesthesia and hydromorphone given intravenously at induction. Some factors were significantly associated with AP but did not remain following the multivariate analysis (odds ratio, OR < 2). These included the male gender and increasing ASA patient score, age, and body weight. Use of continuous infusion of analgesics or anesthetic agents was associated with a greater incidence of AP (OR 1.8) when utilized during anesthesia but not when given postoperatively. ME, in spite of a low occurrence, had the highest OR of 22.3, and upper airway surgery was next with OR 9.2. No specific breed was identified. Time of day, extubation during anesthesia, and the use of anticholinergics, positive‐pressure ventilation, or epidural analgesia were not associated with AP. Regurgitation was witnessed in only two cases with AP. Forty‐six of the 240 dogs (19.2%) were euthanized or died prior to discharge. However, an association of death with AP could not be identified from the available data, so mortality was not determined [20]. A smaller retrospective study found a 16% incidence of anesthesia‐associated AP in 125 dogs diagnosed over a 3.5‐year period. They also found AP following sedation in 16% of these cases as well [176]. A retrospective case‐control study examined AP within 48 h of anesthesia in dogs with intervertebral disk disease. Significant risk factors for postoperative AP included preanesthetic tetraparesis, a cervical lesion, MRI study, multiple anesthetic episodes, longer duration of anesthesia, and postanesthetic vomiting or regurgitation [171]. Although AP appears to be less common in cats, it does occur. Levy et al. evaluated the cases of 28 cats diagnosed with AP that presented over a 12‐year period. Vomiting was the most common predisposing factor identified. Anesthesia was important in 18%; enteral nutrition (18%), neurologic disease (7%), and laryngeal disease (3.6%) were also identified [177]. In 33 cats with AP, Dear et al. found that GI disease was the most common risk factor (25/33); 27% (9) of these cats had been anesthetized within 3 days of the diagnosis [178]. Dogs with laryngeal paralysis often develop AP, especially following surgical intervention [174]. Even though the overall incidence of aspiration occurring perioperatively is low, predisposing factors are common and AP carries a high risk of mortality. Vigilant monitoring for signs of aspiration is necessary, especially since most cases are subclinical, similar to GER and esophagitis. Perioperative management to prevent these complications with appropriate intervention as soon as possible is always indicated. These strategies are described in the GER section. Cisapride may be useful as a preventive treatment for AP. The incidence of AP was significantly less in dogs given a 90‐min infusion of cispride prior to laryngeal surgery [140]. Anesthetic management with attention to preventive measures has shown good success. Baetge et al. altered several aspects of the anesthetic protocol for 149 patients that were repeatedly anesthetized for radiation therapy. The incidence of pneumonia in 146 cases performed prior to the modifications was 10 times greater than in the study group. They kept patients in sternal as much as possible and always in recovery, avoided anticholinergics, did not use μ‐opioid receptor agonists, kept the head elevated above the shoulders, maintained endotracheal tube cuff inflation until just before extubation, and aseptically handled all anesthesia supplies [179]. Of 105 geriatric dogs given famotidine, maropitant, and fentanyl for anesthesia, none vomited, four showed nausea, two regurgitated, and one developed AP postoperatively [180]. Rabbits, horses, ruminants, and humans appear to be especially sensitive to the effects of anesthetic agents on GI motility [21,22,181–185]. Postoperative nausea and vomiting (PONV) and ileus are very common in humans, and postoperative ileus (POI) is one of the most common reasons for prolonged hospitalization in humans [21–24,185]. Although most frequent following GI surgery, POI is seen with other procedures in humans [21] and with many types of surgery in horses. It is also seen in critical care patients that have not had anesthesia or surgery [5,7,186]. POI is associated with increased mortality following colic surgery in horses [23,183], while colic in general and cecal impaction, in particular, are potentially serious complications seen following routine anesthesia and surgery in horses and may be associated with the development of ileus [9,184]. Rabbits are prone to GI stasis following stressful situations, including anesthesia [13,181,182]. Dogs and cats certainly suffer from disruption of normal motility during and after anesthesia; however, related clinical problems are not frequently reported. Numerous researchers have used the dog as a model for the investigation of POI, but there are few specific reports of the incidence seen in clinical patients [186]. In fact, POI is rarely mentioned in veterinary surgery textbooks or reviews of abdominal surgery in small animals. With the advent of intensive multimodal pain management protocols and more diverse and challenging surgical procedures, the potential of these interventions to negatively affect motility has only increased. Prolonged gastric emptying time has been identified in dogs during hospitalization using a wireless capsule to measure intestinal motility. Median emptying time was 17.6 h at home versus 71.8 h in hospital. Other parts of the intestine were not affected in this study [187]. Decreased motility in dogs that were fasted for 12–24 h was identified using ultrasound [188]. Intestinal function is temporarily disrupted by most anesthetic/analgesic agents, including anticholinergics, μ‐opioid receptor agonists, α2‐adrenergic receptor agonists, inhalants, nitrous oxide, and induction agents other than ketamine [24,26,32,189–195]. Xylazine and medetomidine can inhibit motility of the stomach, small intestine [191,192], and colon [193] for hours in the dog. Anticholinergic drugs profoundly reduce GI motility in most species [196–203]. The effects of opioids on motility are many and varied. μ‐Opioid receptor agonists given systemically delay gastric emptying, increase sphincter tone, and variably affect intestinal smooth muscle contraction. Propulsive motility is inhibited and segmental contractions are enhanced, especially in the colon; absorption is increased while secretion is decreased. Constipation is a frequent complication with systemic opioids, from the combined effects on fluid transport and prolonged transit time [32,195,203]. In contrast, when morphine is administered epidurally, GI motility returns much faster in both dogs and humans [204,205]. Following general anesthesia in humans, motility generally returns to the small intestine first, then the stomach, and eventually the colon, which may require 5 days or more [206]. In experimental dogs with ileus, motility of the distal intestine and colon recovered first, followed by the proximal intestine and the stomach [207]. Torrente et al. evaluated 237 dogs that were anesthetized for diagnostics or surgery and found that 33.4% of these developed GI issues post‐anesthesia [12]. 17.3% had GER, 5.5% vomited, and 10.5% developed diarrhea. Duration of anesthesia, intra‐abdominal surgery, and positioning changes were significantly associated with GER; treatment with colloids for hypotension, anesthetic duration, and altering the mode of ventilation increased the risk of vomiting postoperatively. Dogs that were given acepromazine for premedication vomited less frequently. Diarrhea was seen more often with longer anesthetic duration, body position or mode of ventilation changes, and hypoxemia. A recent report of 82 dogs anesthetized for emergency abdominal surgery found that 50% were anorexic, 21% developed diarrhea, and 26% had decreased motility postoperatively [208]. A 2014 investigation examined the effect of laparoscopy and prolonged sevoflurane anesthesia on propulsive motility and transit time in dogs anesthetized for ovariohysterectomy [209]. Following oral administration, a wireless sensor continuously measured intraluminal pressure, pH, and temperature as it traversed the GIT. The pressures created by gastric and small intestinal contractions and their frequency were recorded, and a motility index and emptying or transit time were calculated. The frequency of contraction was not different from awake controls; however, changes in motility were seen within 20 minutes of induction. The motility index and the amplitudes of contraction of both the stomach and small bowel decreased significantly. Emptying and transit times were prolonged, with means of 49 and 11.5 hours and maxima of 59 and 14 h, respectively. Laparoscopy and ovariohysterectomy were performed in all the dogs, so it is unclear what specific effects these procedures may have had on GI function. All dogs were given hydromorphone and cephalexin for recovery, which could have affected the postoperative results, especially the opioid. The results of this study found a decrease in the force of intestinal contraction, inhibition of propulsive motility, and prolonged gastric emptying and small intestinal transit time. The mechanism of POI is multifactorial, with neural, hormonal, inflammatory, and pharmacologic components, but the inflammatory response seen in the muscularis externa is considered the most important and likely the primary cause of prolonged POI. Activation of the sympathetic nervous system via a reflex arc between splanchnic afferents and adrenergic neurons in the spinal cord inhibits motility very soon following the surgical incision, but this effect is short‐lived without other insult to the GIT. Handling of the intestine stimulates neural pathways that involve the brainstem and the hypothalamus to inhibit motility with activation of the hypothalamic–pituitary–adrenal axis. Corticotropin‐releasing factor (CRF), calcitonin gene‐related peptide, and substance P are released, resulting in production of multiple inflammatory mediators. CRF release in the hypothalamus affects function of the ANS in the brain, resulting in decreased activity of vagal efferents, which inhibits GI motility. Tissue handling also incites a local inflammatory response within the intestine that is proportional to the intensity of intestinal damage. Mast cells, primarily located in the mucosa and submucosa are activated as well as the resident macrophages that are located near the myenteric plexus of the muscularis layer. Activation of macrophages is an important step in the inflammatory phase as influx of other leukocytes follows and release of inflammatory mediators is stimulated. Enteric glial cells are very sensitive to mechanical stimuli with direct handling of intestine, pressure from distension, and tissue damage caused by edema and inflammation. These cells are normally involved in modulation of motility and glial dysfunction has been identified in many GI diseases, including POI. Alteration of the intestinal microbiome also occurs and contributes to the inflammation and loss of barrier function seen with POI. Surgically induced peritoneal inflammation also occurs that contributes to the local and systemic effects seen. POI is further exacerbated by the effects of anesthetic agents on motility, the use of opioids, and also the presence of peritonitis and sepsis [195,206,210–214]. Numerous interventions have been attempted in people to reduce or ameliorate the incidence of POI. The Enhanced Recovery Society has published guidelines for most every body system based on current best practice protocols to prevent or minimize the occurrence of POI following elective and emergent procedures. Good success has been seen, with improved outcomes, shorter hospitalization, decreased costs, and improved patient well‐being and satisfaction (Table 41.4). Enhanced recovery after surgery (ERAS) protocols involve all members of the surgery/anesthesia/recovery team and address all aspects of the perioperative period. Use of less‐invasive surgical techniques whenever possible, short fasting times, preanesthetic carbohydrate loading, avoidance of opioids, epidural anesthesia–analgesia, careful fluid therapy to avoid overhydration, early ambulation, and enteral intake are just some of the components of an ERAS protocol. Detailed descriptions can be found at https://erassociety.org. Campoy has reviewed the application of ERAS protocols in veterinary medicine with an emphasis on local anesthesia–analgesia [215]. Table 41.4 Recommendations for prevention and treatment of postoperative ileus (POI). Source: Compiled from Whitehead et al. [5]; Grocott et al. [21]; Mythen [185]; Boysen [186]; DeHaven‐Hudkins et al. [203]; Nakayoshi et al. [204]; Pöpping et al. [205]; Lubawski and Saclarides [206]; Sido et al. [210]; van Bree et al. [211]; Vather and Bissett [212]; Hussain and Park [213]; Mazzotta et al. [214]; Campoy [215]; Yang et al. [216]; Kaye et al. [217]; Chen et al. [218]; Cooke et al. [219]; Leslie et al. [220]; Rimbäck et al. [221]; Malone et al. [222]. FIO2, inspired concentration of oxygen; NSAID, non‐steroidal anti‐inflammatory drug; COX‐2, prostaglandin synthase‐2. a COX‐2 inhibitors are avoided in patients where risk of anastomotic leakage or sepsis is present. Many patients that require anesthesia for GI disease are emergent and/or may be critically ill. Thorough preanesthetic evaluation and preparation prior to anesthesia help to minimize complications; however, this is not always possible in extremely emergent patients. Recommendations for a basic workup include measurement of hematocrit, total protein, blood urea nitrogen (BUN), creatinine, serum alkaline phosphatase (ALP), alanine aminotransferase (ALT), aspartate aminotransferase (AST), bilirubin, albumin, electrolytes, and total carbon dioxide. Blood gas analysis and lactate are important in sick patients. Additional diagnostics appropriate to the problem at hand should be evaluated, such as radiographs or abdominal ultrasound. Computed tomography or MRI is sometimes used in stable patients for determination of diagnosis and prognosis prior to surgery. General guidelines for the management of anesthesia for abdominal procedures are similar to those for other disease conditions. Preservation of tissue oxygen delivery through the maintenance of blood flow and avoidance of hypoxemia are paramount. Additional consideration should be given to predictable complications (e.g., endotoxemia with damaged intestine, reperfusion injury following derotation of twisted segments of intestine, cardiac dysrhythmias during splenectomy, or GDV), and appropriate treatments planned/prepared. Finally, administration of adjunctive drugs to provide pain management, speed return of GI function, and limit adverse effects such as nausea and vomiting will improve patient care and outcomes. The plan for an exploratory laparotomy varies more with the patient’s condition than with the primary GI problem. All levels of patient status using the ASA I–V +/‐ E classification may be seen. Some patients are healthy (e.g., gastropexy) and their management is similar to that of other elective abdominal procedures (e.g., ovariohysterectomy). Other patients may be severely ill and require laparotomy as a life‐saving intervention. Fluid deficits, electrolyte abnormalities, and acid–base imbalance should be corrected as much as is reasonable prior to induction. All equipment for anesthesia and monitoring should be assembled and tested for proper function prior to induction. Some patients may have pre‐existing organ dysfunction that may or may not be related to the reason for exploratory laparotomy. Ketamine, especially as a prolonged continuous infusion, should probably not be used in patients with renal dysfunction, especially cats since they excrete ketamine and its metabolites to a significant extent in the urine. Where available, thiopental should be avoided in debilitated patients and those with severe liver dysfunction. It should also not be used in sighthounds. Both thiopental and propofol can sensitize the myocardium to catecholamine‐associated arrhythmias and should be used cautiously in patients with pre‐existing dysrhythmias or cardiac disease, or when arrhythmias are highly likely such as with GDV or splenic masses. In both research and clinical studies, alfaxalone has not been reported to cause arrhythmias [43,44,223]. Etomidate is often preferred for patients with pre‐existing myocardial dysfunction (e.g., poor contractility) who are otherwise healthy as it has minimal effects on cardiovascular function. A dose of midazolam or diazepam, 0.2 mg/kg IV, just prior to etomidate will improve muscle relaxation and facilitate intubation. Historically, etomidate was not recommended in sick patients as it causes adrenal suppression and has been associated with greater mortality in humans [224–227]. Some reports have not found greater mortality when used only for induction [228,229], however, a tendency toward adrenal insufficiency or hospital‐acquired pneumonia and sepsis has been identified [227–229]. Qin et al. found significantly decreasing levels of cortisol and aldosterone during a 3‐h infusion of etomidate in dogs [230]. Also, a recent clinical study of etomidate for induction in healthy dogs was stopped due to the development of pigmenturia [231]. Inhalant induction is not recommended for exploratory laparotomy, especially in patients with frequent emesis. Following effective premedication, healthy patients and those in good overall condition (ASA I, II) can be induced with titration of usual doses of ketamine, propofol, and alfaxalone separately or in combination [232]. In healthy dogs, ketamine increases heart rate, blood pressure, and cardiac output, while these parameters are decreased by propofol and thiopental in a dose‐dependent manner. Propofol interferes with baroreceptor responses and is more often associated with bradycardia and hypoxemia [226,233]. Alfaxalone increased heart rate and decreased cardiac output, arterial blood pressure, and systemic vascular resistance in healthy cats and dogs without premedication [43,44]; however, in clinical settings, hypotension following induction is not common. No differences were found when induction with alfaxalone was compared to diazepam–fentanyl with or without propofol in ASA III–V dogs [223]. Ketamine is often suggested for induction due to its cardiovascular‐stimulating effects. However, since this is due to centrally mediated increases in sympathetic tone, the opposite effect can be seen in very ill patients with poor sympathetic reserve [233–235]. Intramuscular premedication can be used in healthy patients anesthetized for elective procedures; however, intravenous premedication is preferred for higher‐risk patients. Combination of a benzodiazepine and an opioid is most often utilized. Midazolam or diazepam with hydromorphone, methadone, oxymorphone, or fentanyl (or its congeners) are titrated intravenously to effect. Since the incidence of vomiting is greater with hydromorphone, other opioids should be used in patients with frequent emesis. The pharmacokinetic profile of remifentanil makes it a good choice for compromised patients. It is used in dogs and cats [236,237]; however, it is quite expensive and not available in some areas. To avoid traditional induction drugs entirely in compromised patients, additional doses of premedicants (opioid and benzodiazepine) can be titrated IV until intubation is possible. Bradycardia and respiratory depression may be significant following high doses of opioids used for induction, so supplies and equipment for intubation, ventilation, and monitoring should be immediately available. Isoflurane or sevoflurane are preferred for maintenance of anesthesia for exploratory laparotomy over halothane as the latter sensitizes the myocardium to arrhythmias and decreases tissue blood flow to a greater extent. Desflurane can be useful for its rapid induction and recovery characteristics, however, it is not commonly used and is unavailable in some countries. It requires a special heated vaporizer to produce consistent concentration of vapor and is more expensive. Nitrous oxide (N2O) is not recommended since it diffuses readily into intestinal organs, causing or exacerbating distention and potentially compromising ventilation. N2O also has significant side effects in humans, including interference with vitamin B12 metabolism, damage to the CNS, and abortion [238]. All the halogenated anesthetics used currently have deleterious effects on the environment; however, those associated with N2O and especially desflurane are much greater. The “carbon footprint” of N2O is reportedly 310 times that of CO2, while desflurane is 2500 times. N2O also contributes to depletion of the ozone layer in the atmosphere [238,239]. Use of desflurane was recently banned by the National Health Service in Scotland [240]. N2O is not commonly used in veterinary medicine and its use in people has greatly decreased. The respiratory and cardiovascular effects of all the inhalants are more pronounced in debilitated patients; hypoventilation and hypotension are common. Pre‐existing cardiovascular depression decreases the minimum alveolar concentration (MAC) required to maintain anesthetic depth. Vaporizer settings should be kept as low as possible to minimize hypotension. Occasionally, discontinuation of inhalant anesthesia and transition to injectable maintenance is necessary to maintain blood pressure in patients with severe sepsis that do not respond to fluid and/or inotropic and/or vasopressor therapy. Patients with a distended GIT, especially those that are obese, are more likely to become hypoxemic, especially in recovery when oxygen is discontinued, due to hypoventilation and atelectasis. Non‐invasive monitoring of blood pressure should be initiated prior to induction, arterial catheterization should be considered soon following induction. Monitoring of blood pressure and oxygen saturation should be continued into recovery; oxygen supplementation is often required postoperatively [172], and cardiovascular support may sometimes be needed as well. To minimize the amount of inhalant needed or if anesthetic depth is inadequate, additional sedatives and/or analgesics are given intermittently or as a CRI. Continuous infusion provides a more constant level of drug delivery, and greatly reduces MAC. Infusions are initially set at a specific rate and the inhalant setting is varied to maintain the desired anesthetic depth, although infusion rates may be varied as well. An opioid, lidocaine, and/or ketamine can be administered in combination or separately as CRIs [241–247], though lidocaine is not recommended for use in cats [248]. α2‐Adrenergic agonists can also be given as infusions. Dexmedetomidine has been evaluated both during and after anesthesia in healthy small animals and found to be useful and without serious side effects, although close monitoring of cardiovascular parameters is warranted [247–249]. Recent reviews of anesthesia of septic human patients do not mention use of dexmedetomidine intraoperatively [229], however, it is used routinely for sedation in critically ill patients, and has been associated with improved outcomes in some cases [250]. In patients without hypovolemia, sepsis, coagulopathy, or dermatitis in the lumbosacral area, epidural administration of a local anesthetic with or without morphine can also provide intra‐ and postoperative analgesia. Local anesthesia using transversus abdominis plane blocks and/or intercostal blocks can be very helpful to provide analgesia and minimize opioid use, especially in the postoperative period [251–253]. Fluid therapy is continued during anesthesia; the type of fluids and the rate necessary will vary depending on the patient’s status. Regular assessment of fluid status is necessary as fluid overload has deleterious effects on the efficacy of fluid exchange in the interstitium, resulting in edema, dysfunction of the glycocalyx, and further damage to tissues [254–257]. Hypotension is common in exploratory laparotomy cases and has been consistently associated with greater mortality in patients with sepsis, peritonitis, and emergency abdominal surgery [226,258–260]. The GIT lacks an autoregulatory response to blood pressure as seen in the brain and kidney and prolonged hypotension can be more damaging to intestinal tissues and should therefore be vigorously treated [226]. If hypotension persists despite correction of volume deficits, infusions of inotropes such as dobutamine and/or dopamine may increase cardiac output and improve blood pressure. Vasopressors are considered when decreased systemic vascular resistance is a significant component of hypotension. Ephedrine is a sympathomimetic which can be given as a bolus; it is effective primarily for mild transient decreases in blood pressure. Phenylephrine, norepinephrine, vasopressin, and epinephrine are given as infusions and titrated to effect. These drugs are potent vasoconstrictors and should be used at the lowest effective dose to minimize tissue ischemia despite improved blood pressure [245,255,260–263] (see Chapter 21 for dosages). Monitoring patients for exploratory laparotomy should include the usual parameters of anesthetic depth, mucous membrane color and refill time, heart rate and rhythm, respiratory rate/effort/pulse strength, and arterial blood pressure. Invasive blood pressure measurement is preferred by anesthesiologists for most laparotomies since changes in cardiac output and pressure can be rapid and/or severe. Pulse oximetry and capnography provide valuable information on patient status with continuous real‐time evaluation of ventilation and gas exchange and are commonly used. Arterial blood gas analysis is used to document respiratory and metabolic status and to periodically evaluate the results of therapy. Lactate measurement is an indirect estimate of tissue perfusion and oxygenation status [264]. Changes in lactate lag behind alterations of tissue metabolism, but measurement at presentation and over time is of value in the evaluation of therapy and prognosis of patients with severe disease [208,265–269]. Because of the variability of blood pressure measurement in hypovolemic, unstable patients, measurement of cardiac output would be very helpful in the assessment of cardiovascular status; however, this is not commonly available for clinical patients. Monitoring of the variation of the pulse pressure or the systolic arterial pressure waveform during mechanical ventilation has been found to be a reliable parameter that infers the presence of hypovolemia [254,270,271], suggesting a need for fluid/volume therapy (Fig. 41.2). Close attention to volume loss and the actions of the surgeon during laparotomy helps to avoid hemodynamic compromise by enabling the anesthetist to anticipate and treat problems in a timely fashion. For example, cardiovascular function is greatly affected by blood loss or removal of large volumes of effusion, and with handling of large masses. Manipulation and derotation of ischemic bowel leads to the release of numerous inflammatory mediators that cause vasodilation and possibly cardiac dysfunction (Fig. 41.3). Monitoring should continue throughout anesthesia and into recovery. Signs of anesthetic depth, heart and respiratory rate, mucous membrane color and capillary refill time, temperature, blood pressure, and electrocardiography should be periodically evaluated. Postoperative pain management is important for the comfort and the outcome of patients with GI disease. Opioids are continued as intermittent boluses intravenously or intramuscularly, or via continuous infusion. Infusions of lidocaine and/or ketamine are also effective. Lidocaine causes nausea and vomiting in dogs at high infusion rates [60,272] but was associated with improved outcomes in humans following abdominal surgery [61,62,218,219], and in dogs with GDV [273] and peritonitis [274]. Lidocaine given intraoperatively has also been shown to decrease postoperative pain in humans [61,62,218,219]. Higher dosing and use of multiple modes of analgesia are often required in the first 24 h postoperatively. These should be decreased as soon as possible so that enteral feeding and ambulation can be instituted and to minimize the incidence of ileus. Infusions of low doses of α2‐adrenergic receptor agonists can be useful, especially in patients with high analgesic requirements or opioid dysphoria. Valtolina et al. found that dexmedetomidine infusion provided effective postoperative analgesia similar to morphine without significant adverse effects in ill dogs [275]. However, infusions of α2‐adrenergic receptor agonists can have significant cardiovascular side effects, so their use should be carefully considered in the individual patient [249]. Figure 41.2 Patient monitor showing systolic pressure (or pulse pressure) variation of the arterial waveform in an anesthetized mechanically ventilated dog. The presence of systolic pressure or pulse pressure variation is associated with hypovolemia and fluid responsiveness. Source: Dr. Juliana Figueiredo, VCA West Coast Specialty and Emergency Animal Hospital, Fountain Valley, CA. Figure 41.3 Mesenteric torsion in a dog presenting for acute onset of abdominal discomfort/pain and profound depression. Manipulation and derotation of ischemic bowel leads to release of inflammatory mediators resulting in vasodilation and potential cardiac dysfunction. Source:. Dr. Jane Quandt, Department of Small Animal Medicine and Surgery, College of Veterinary Medicine, University of Georgia, Athens, GA. Non‐steroidal anti‐inflammatory drugs (NSAIDs) are used in human, ruminant, and equine patients following GI surgery with minimal complications [220,276–283], although a greater risk of dehiscence of anastomosis was associated with NSAID use in humans [284]. Dogs and cats are relatively sensitive to the GI side‐effects of NSAIDs such as ulceration and hemorrhage [280–282]. Although useful in healthy patients anesthetized for elective procedures such as gastropexy, the risks and benefits should be considered and it may be best to avoid NSAIDs in patients with GI disease, renal compromise, hypotension, liver disease, coagulopathy, and other conditions that could be exacerbated by NSAID administration [283]. Regurgitation, food retention, and defective peristalsis of the esophagus are seen in patients with primary esophageal dysmotility without dilation or persistent megaesophagus (ME) [285]. ME is a persistent dilation caused by damage to the esophageal musculature or defective peristalsis. Classified as congenital or acquired, regurgitation is the most frequent clinical sign, with weight loss and poor body condition in adults, intolerance of solid food, and failure to thrive in young patients. Aspiration pneumonia (AP) is common, and cough and abnormal lung sounds may be present. ME is rare in cats but not uncommon in dogs. However, cats with dysautonomia, an acquired abnormality of autonomic ganglia that leads to GI dysfunction, usually have ME. Congenital idiopathic ME has been reported in several breeds of dogs and a few cats. A lack of vagal sensory input is presumed to be the underlying mechanism. Peristalsis of the esophagus does not occur because the presence of a food bolus is not detected. Acquired ME is idiopathic, caused by esophageal obstruction, and secondary to other diseases. Vascular ring anomaly, esophageal stricture, hiatal hernia, tumor, granuloma, and foreign bodies cause obstruction. With time, muscular damage caused by dilation proximal to the lesion becomes irreversible. Idiopathic ME is the most common cause of the acquired form in adult dogs. The mechanism is thought to be similar to the congenital form; with loss of normal motility, ingesta remains in the esophagus and eventually results in dilation. Acquired ME is secondary to or associated with peripheral neuropathy, neuromuscular disease, laryngeal paralysis, severe esophagitis, lead poisoning, lupus myositis, and hypoadrenocorticism. Hypothyroidism is reported but has been discounted by some authors [285–287]. Chronic or recurrent gastric dilation with or without volvulus was also identified as a risk factor in a retrospective evaluation of 136 dogs diagnosed with ME. Evidence for concurrent hypothyroidism in these cases was evaluated but was not found [287]. Myasthenia gravis (MG) is a very common cause of ME, reported to occur in 25–30% of cases. ME is often the first clinical sign of MG in dogs [285,288]. MG is reported in cats, but is more common in dogs likely due to the higher proportion of striated muscle in the canine esophagus. ME is only rarely seen in cats with MG [289]. A recent retrospective study of 99 dogs with ME not associated with a structural lesion found a high proportion of idiopathic cases; 10 were congenital, 89 were acquired, with 42.7% of the latter idiopathic, and 38.2% due to MG [290]. Transient ME was seen in three dogs with coral snake envenomation. All three dogs had respiratory difficulty, two developed AP, and two required ventilation [291]. An unusual outbreak of ME occurred in 398 dogs in Latvia and Australia from 2014 to 2018. The feeding of two different brands of dry dog food was incriminated in each country, although a specific ingredient could not be identified and the underlying physiologic defect was not determined [292]. Patients with ME may be anesthetized for diagnostics or treatment, such as endoscopy, computed tomography, electromyography, nerve conduction studies, muscle/nerve biopsy, bougienage, foreign body removal, and vascular ring anomaly correction. ME may also be a concurrent disease in patients anesthetized/sedated for unrelated procedures such as dentistry or radiographs. Since ME is often associated with neurologic disease, these patients should be closely monitored for esophageal dysfunction and possibly respiratory insufficiency [290]. GER, regurgitation, and aspiration are the primary concerns when anesthetizing patients with ME. Prolonged fasting is not advised as the dilation prevents complete emptying of esophageal contents and an increased incidence of GER has been seen with long fasting times in normal dogs [110,111]. Some patients with chronic disease may be thin or debilitated due to malnutrition and some may be dehydrated if unable to retain adequate fluid intake. Many have repeated episodes of AP and are at greater risk of hypoxemia perioperatively. Patients should be stabilized prior to elective anesthesia with IV fluid therapy and appropriate treatment for pneumonia. A dedicated anesthetist is important for patients with ME, as airway management and constant monitoring for leakage of esophageal contents are necessary. Suction of esophageal contents should be performed immediately after intubation and just before discontinuation of anesthesia to reduce the risk of aspiration. Sternal recumbency and elevation of the neck with the nose tipped down may also help decrease the incidence. If regurgitation occurs, the nose and head should be lowered immediately to allow drainage. Preoxygenation is recommended for those with active pneumonia; these patients should be restrained cautiously to avoid struggling and excessive stress. Anesthetic drugs should be chosen based on the patient’s status, with rapid IV induction and intubation necessary to secure and protect the airway as quickly as possible. Avoidance of drugs that cause vomiting such as some opioids (e.g., morphine or hydromorphone) and α2‐adrenergic receptor agonists is also recommended. Reversible and shorter‐acting agents are preferred. In recovery, extubation should be delayed as long as possible, and oxygen should be supplemented in recovery until oxygen saturation is consistently normal. Gastroprotectants, antacids, and antiemetics given preoperatively are recommended. Patients with ME due to MG may be treated with pyridostigmine bromide, which increases levels of ACh by inhibiting anticholinesterase that would normally break down ACh. Cholinergic crisis is therefore possible, but this is rare. The effect of neuromuscular blocking agents (NMBAs) is unpredictable in myasthenic patients and possibly others with idiopathic ME. They do not respond to depolarizing agents but are quite sensitive to nondepolarizing agents. Very low doses of NMBAs should be utilized if paralysis is necessary. Specific monitoring of the extent of blockade must be available. Sugammadex is effective as a reversal agent for NMBAs [293]. The incidence of gastric dilation–volvulus (GDV) at 50 emergency clinics in the United Kingdom over a 17‐month period was 0.64% (492/77,088 cases) [294]. Approximately, 10 cases per clinic per month is an underestimation, however, as it does not include cases presenting during regular hours at day clinic practices. Acute GDV is life‐threatening, seen most often in adult large or giant breed dogs, especially those with a deep‐chested conformation. However, it can occur in small breed dogs and puppies, and has been reported in five cats, three with concurrent diaphragmatic hernias [295–297]. Although an underlying motility disorder is presumed to be important in the pathogenesis and altered motility patterns have been identified after GDV in patients and research subjects with created GDV, the actual pathogenesis of the condition has not been elucidated [298–303]. The etiology is multifactorial with numerous risk factors identified, including large/giant breed; deep‐chested conformation; “slim” body condition; middle to older age; close relative that has experienced GDV; multiple diets, feeding, and exercise routines; aerophagia; stress; hospitalization; nervous, anxious, or aggressive demeanor; and gastric foreign body [294,295,304–307]. Recent studies have identified specific genetic immune system variants in Great Danes with GDV [308]. Inflammatory bowel disease and dysbiosis of the GI microbiome may also be associated with increased risk of GDV [305,309]. Mortality has decreased since the 1980s but is still high. Studies have reported mortality rates of 10–26.8% [294,304,306,310–316], but these refer to dogs treated surgically and do not reflect the actual case numbers seen. Unfortunately, many cases are euthanized at presentation because of prognosis, cost, or other factors [314,315]; clinical practices aimed at prevention and early recognition would likely have the most significant effect overall [306,315]. Gastropexy has proven to be preventive in dogs at high risk and those following GDV. Reported recurrence rates are 0–5% with most developing dilation but not volvulus [306,316,317]. Improved survival is likely associated with improvements in medical therapy, especially fluid resuscitation, early decompression of the stomach whenever possible, and timely surgical intervention. Mortality is correlated with damage to the stomach and surrounding organs, gastric perforation, sepsis, peritonitis, the presence of cardiac dysrhythmias pre‐ or postoperatively, both hypo‐ and hyperthermia at presentation, postoperative acute renal failure, hypotension at any time during hospitalization, the duration from onset to presentation or surgery, the time from presentation to surgery, and the severity of systemic compromise at presentation [306,310–314]. Reperfusion injury has also been implicated as a significant factor associated with mortality with GDV [318–320]. GDV is always an emergency. Blood flow to the stomach and surrounding organs is compromised as abdominal veins become obstructed, greatly decreasing venous return to the heart, and producing severe relative hypovolemia and distributive shock. Distention of the stomach also restricts ventilation via interference with diaphragmatic excursion. Early clinical signs include restlessness and anxiety, followed by hypersalivation, vomiting and/or retching, and distention of the abdomen (Fig. 41.4) Eventually, depression, weakness, and dyspnea develop if distention is not relieved. Vomiting, salivation, and sequestration of hydrogen and chloride ions that occur with gastric outflow obstruction initially cause metabolic alkalosis. Metabolic acidosis develops later, secondary to the effects of ischemia and decreased hydration, with increased lactate production and the release of inflammatory mediators. Endotoxemia can also develop with damage to the portal system. Reperfusion injury occurs when ischemia is reversed with gastric decompression/derotation and redistribution of fluid volume [306,318–320]. Figure 41.4 Preparation of a Great Dane with gastric dilation–volvulus, (GDV) for anesthesia and surgery. Intravenous access has been secured and fluid resuscitation initiated, ECG leads have been attached to evaluate cardiac rhythm, and the degree of abdominal distension is assessed. Note the salivation and depressed mentation of the dog. Source: Dr. Jennifer Adams, with permission. Intravenous fluid therapy and gastric decompression should be initiated as quickly as possible. Baseline laboratory evaluation should include packed cell volume (PCV), total solids (TS), electrolytes, creatinine, and acid–base status. A clotting profile and venous lactate are useful to evaluate prognosis and guide therapy but generally should not delay surgical intervention if the owners would proceed regardless of the results. Gastric necrosis is likely present when more than one hemostatic test is abnormal and when lactate is greatly elevated at presentation [321–323] and/or fails to decrease significantly with fluid resuscitation. Increased lactate at presentation [265,321–324] and decreased clearance were associated with higher mortality [265,325]. Radiography to distinguish between dilation and dilation–volvulus, should be delayed until cardiovascular resuscitation is well under way. Anesthetic personnel, equipment, and supplies should be made ready prior to arrival of the patient so that surgical intervention may commence as soon as possible. Previous emergent therapy was to administer “shock” doses (80–90 mL/kg) of crystalloid solution via large‐bore catheters, using multiple venous access points. However, use of goal‐directed resuscitation guidelines to achieve appropriate clinical endpoints rather than a specific volume avoids hypervolemia and tissue edema and may improve outcomes [254,255,326–330]. Portions of the estimated deficit are given over 15–30 min and the patient’s response is determined. Clinical parameters to monitor include heart rate, arterial blood pressure, mucous membrane color and perfusion, tissue oxygenation, and mentation. PCV, TS or total protein, blood gases, and venous oxygen saturation should be measured as needed. Use of balanced crystalloid solutions is useful; however, faster and longer‐lasting resuscitation is achieved with the addition of small volumes of hypertonic saline 7.5% at 2–4 mL/kg over 15 min, or colloids at 5–10 mL/kg over 30 min. Alternatively, a combination of colloid and hypertonic saline is given at 4–6 mL/ kg [328]. This simultaneous use of hypertonic saline and colloid has been shown to improve cardiovascular status in both experimental and clinical cases of GDV [331,332]. As previously mentioned, acute kidney injury has been seen in people following treatment with colloids, so their use is not recommended in human medicine. Thoracic auscultation and electrocardiography should be performed soon following presentation, as cardiac arrhythmias are common with GDV. Most are ventricular in origin, although sinus tachycardia, atrial fibrillation, and atrioventricular block can also occur, and the “R on T” phenomenon is sometimes seen (Fig. 41.5) [304,306,311,333]. Specific antiarrhythmic therapy may not be necessary if the dysrhythmia is of minimal cardiovascular consequence or correction of hypovolemia, hypoxemia, hypercarbia, acid–base status, and/or electrolyte abnormalities provide resolution. Treatment of ventricular tachycardia is often needed when extrasystoles are numerous or multifocal, the sustained rate is very high (> 160 beats/min), extrasystoles are very early such that an “R on T” phenomenon is possible, and always when hemodynamic status is affected by the arrhythmia. Lidocaine bolus is given slowly IV at 1–2 mg/kg followed by CRI at 25–100 μg/kg/min [334]. Postoperative therapy for cardiac arrhythmias is often necessary. A lidocaine CRI is particularly useful as it decreases anesthetic requirements in dogs by as much as 37–43% [60,335] and may have positive effects on outcome. When lidocaine was given preemptively in dogs with GDV, significantly fewer cases with cardiac dysrhythmias, less renal impairment, and shorter hospitalization time were found as compared to a historical control group [273]. Lidocaine has also been shown to have numerous anti‐inflammatory effects [336–338] and may have prokinetic effects [61,218,221,222]. Improved survival has also been seen in dogs with septic peritonitis [274] and horses treated surgically for small intestinal colic [340]. Figure 41.5 Ventricular tachycardia seen postoperatively in a dog anesthetized for correction of gastric dilation–volvulus (GDV). Source: Dr. Jennifer Adams, with permission. Despite much research devoted to the effects of ischemia/reperfusion injury and its prevention or treatment, few therapies have been adopted in clinical practice. Avoidance of hyperoxia may be useful since greater damage was seen following reperfusion in cardiac arrest patients maintained at higher‐than‐normal PaO2 levels [341]. Inhibition of oxygen‐free radical formation with antioxidants and iron‐chelating drugs such as deferoxamine has been shown to reduce reperfusion injury in dogs with GDV [318,342]. Ethyl pyruvate has been shown to preserve, protect, and even improve cellular function in many tissues and species with ischemia/reperfusion injury and may prove useful as a treatment for dogs with GDV or other ischemic lesions [343]. Gastric lavage is sometimes necessary during correction of a GDV. Considerations for lavage are similar to those for GER, upper GI endoscopy, and ME. Leakage of gastric contents is common and the endotracheal tube can be displaced. The head should be tipped downwards to direct gastric contents away from the pharynx. The pharynx should be examined and cleaned of any fluid or debris that may have leaked around the nasogastric tube prior to recovery. Postoperative dysmotility is reported in GDV patients; they should be monitored closely in recovery for regurgitation, vomiting, ileus, and aspiration [299,303,305,320]. Causes of hemorrhage into the peritoneal cavity are traumatic or atraumatic. Trauma is most often due to injury from impact with motor vehicles or penetrating objects. It can also be caused by a fall and during fights with other animals. Abdominal organs, especially liver and spleen, vasculature, and/or the abdominal wall are affected. Atraumatic lesions include hematoma or neoplasia of abdominal organs, organ displacement or torsion, and coagulation abnormalities (e.g., rodenticide toxicity and autoimmune thrombocytopenia) [344–348]. Anaphylaxis was also recently reported as a cause of hemoperitoneum in 11 dogs [349]. Coagulopathy can also result from acute trauma and has been suggested to contribute to 25% of deaths due to uncontrolled hemorrhage in people [350]. Initially, a hypocoagulable state caused by the inflammation produced by tissue injury and shock develops, followed later by hypercoagulation with thromboembolism and organ failure. Hypothermia and acidemia are often present and contribute to coagulopathy. Acute traumatic coagulopathy is diagnosed using routine clotting profiles and viscoelastic measures such as thromboelastography [344–346,350]. It was identified in 11/33 dogs with severe trauma [351] and in 1/18 dogs and 1/19 cats with lesser injury [352]. Hemorrhage due to neoplasia is common, especially in dogs. Potential sites include the liver, spleen, mesentery, and adrenal glands, with the spleen being most often affected. Hemangiosarcoma is the most common abdominal neoplasm in both dogs and cats and usually affects the spleen and/or liver. Long‐term prognosis is guarded to poor [347,348,353], but a high percentage of canine cases with abdominal hemorrhage survive anesthesia and surgery to discharge [353]. A retrospective investigation of spontaneous atraumatic hemoperitoneum in 65 cats found that 46% had abdominal neoplasia and 54% had non‐neoplastic disease; hepatic necrosis and coagulopathy accounted for most of the latter cases. Only eight of the cats (12.3%) survived to discharge [354]. Hemorrhage into the abdomen can be gradual or sudden, and can quickly become life‐threatening. Clinical signs are similar to blood loss from any cause with progressive signs of hypovolemic shock such as increasing tachycardia, pale mucous membranes, weak pulses, weakness and/or lethargy, decreased mentation, and cold extremities. Compensatory mechanisms such as splanchnic vasoconstriction and reflex tachycardia may initially mask clinical signs of hypovolemia; however, as blood loss approaches 30% of the blood volume, these mechanisms are exhausted [345]. Hypothermia is seen in dogs and is common in cats at presentation. Abdominal distention, a palpable fluid wave, abdominal pain, and subcutaneous discoloration at the umbilicus and scrotum are also sometimes seen. Radiography may show loss of abdominal detail with a “ground‐glass” appearance. Ultrasound will reveal the presence of free fluid and may reveal a mass or metastatic disease. Abdominocentesis produces a bloody sample that does not clot and that has a PCV similar to the systemic PCV is diagnostic for hemorrhage [344]. Peritoneal lavage may be necessary with smaller volumes of blood loss or when ultrasound is not available [347,348]; a PCV of 2–5% in lavage samples is indicative of hemorrhage [347]. Depending on the severity of volume lost, the systemic PCV, TS/TP, and platelet count may be normal or decreased, and, as lactate increases, base excess becomes more negative, and central venous oxygen tension decreases [345]. Anesthetic considerations for hemoabdomen include hypovolemia, anemia, respiratory compromise associated with large masses or fluid volumes compressing the diaphragm, and any organ dysfunction that may be present. Ideally, the patient is stabilized prior to surgery with intravenous fluids and sometimes transfusion. The rate of blood loss is important, and some prefer to replace erythrocyte losses after the source of bleeding is stopped if the patient is stable enough to delay transfusion. It is not necessary to correct the entire RBC deficit preoperatively; however, the patient should be treated to achieve normovolemia and a PCV ≥ 30% in dogs or 20% in cats, as oxygen delivery is usually adequate above these levels. “Hypotensive” resuscitation to an endpoint less than ideal, such as systolic arterial pressure of 90 mmHg or mean arterial pressure of 60 mmHg, is sometimes pursued when control of the source of hemorrhage can be achieved quickly [346]. Lower PCVs are often tolerated during anesthesia when they are a result of chronic blood loss rather than acute hemorrhage. Patients with anaphylaxis require treatment with an antihistamine and/or epinephrine and may not require surgical intervention [349]. The volume of prior and ongoing blood loss must be estimated and replaced to limit effects on cardiac output. Estimating loss is often difficult; techniques include weighing bloody sponges and towels, tracking the volume of lavage fluid used, and estimating the volume of blood and fluid collected in suction bottles. Isotonic crystalloids are used for initial volume replacement. The specific volume required is unknown and depends on the speed of loss, but multiples of the volume lost are recommended (e.g., 1.5–3 times the loss for acute hemorrhage and up to eight times for gradual loss affecting the intracellular volume). When intracellular volume is affected, replacement can proceed more slowly than when acute hemorrhage is present. Boluses of 10–20 mL/kg for dogs and 5–10 mL/kg for cats are given, the patient is reassessed, and boluses are repeated as needed up to appropriate endpoints. Colloids were considered a rational replacement for plasma loss, given at a ratio close to 1:1, if PCV and protein levels were adequate. However, concerns about renal damage with colloid therapy have decreased their use [355–357]. Losses greater than 20–30% of the patient’s total blood volume should be replaced with whole blood, plasma and packed RBCs, or an RBC substitute such as Oxyglobin®, if available, to ensure adequate oxygen delivery. Autotransfusion is useful in some cases but not recommended with septic peritonitis, urinary rupture, and neoplastic lesions. Catecholamine and inotrope infusions are used for other procedures when needed, but vasopressin may produce better results in patients with hemorrhagic shock [358]. TS or total protein levels should be maintained at greater than 4–4.5 g/dL. PCV and TS are measured intermittently to monitor the efficacy of therapy and the speed of blood loss, realizing that hemodilution will be present and that colloids will interfere with refractometer readings. Response to volume restoration and increased oxygen‐carrying capacity (e.g., normalization of heart rate and blood pressure) are useful guides to fluid therapy during anesthesia. Improvement of variation of the systolic arterial pressure waveform or pulse pressure may also be seen. Lactate is a useful indicator of tissue oxygen delivery as it increases with severe hypovolemia and decreases as volume, cardiovascular function, and perfusion improve [264]. Although rapid volume replacement is sometimes necessary for extremely hypovolemic patients, large volumes cause hypocoagulability and result in “dilutional coagulopathy” [345,346,353]. Evacuation of blood and manipulation of organs or abdominal masses can cause significant hemodynamic instability. Sequestration of a portion of the blood volume in the exteriorized tissues and/or redistribution of blood volume affects cardiac output and peripheral perfusion. In humans, anesthetized for abdominal surgery, a syndrome of tachycardia, hypotension, and cutaneous hyperemia has been reported, called “mesenteric traction syndrome” [98,358,359]. This has not been described or perhaps not investigated in animals, but prostacyclin, histamine, and other vasoactive substances are released, causing fluctuations in hemodynamic status when tension is placed on mesenteric vasculature. The review of 38 studies with 1102 patients by Olsen et al. found mesenteric traction syndrome developed in 76% of patients anesthetized for major abdominal surgery that included procedures on the GIT, aorta, and large organs. Cardiac arrhythmias are common with splenic masses and other functional neoplasms (e.g., pheochromocytoma). Ventricular premature contractions and tachycardias are seen most often in dogs. The cause is uncertain, and they may persist during recovery. Predisposing factors such as hypovolemia, hypotension, hypoxemia, hypercarbia, and electrolyte abnormalities are other causes of dysrhythmias under anesthesia. Anesthetic and analgesic drug choices are based on patient status. Those with shorter duration and reversibility are preferred. The MAC of inhalants is decreased with volume loss; vaporizer settings should be as low as possible and decreased in anticipation of this when sudden hemorrhage occurs. Although the use of dexmedetomidine in hemorrhaged dogs did not compromise global perfusion significantly [360], it did prevent the pulse pressure variation normally associated with hypovolemia [361]. This effect could interfere with monitoring and volume resuscitation of hemorrhaging patients. Dogs in this study were also given atropine to block the cardiac effects of dexmedetomidine and allow vascular effects to be identified. Atropine is not recommended when dexmedetomidine is used in clinical patients, so the effects of dexmedetomidine alone are not known when hemorrhage is present. Consequently, this drug should be avoided or used very judiciously in those with less significant blood loss. Overall, cancer of the GIT is not common (< 10%) when compared to all types of neoplasms in dogs and cats. Most patients are middle‐aged dogs or older cats, and many are male. Lymphoma, carcinoma, leiomyoma, and gastrointestinal stromal tumors are found in the GIT of dogs and cats and malignancy is very common. Adenocarcinoma is most common in the lesser curvature and pyloric area of the stomach and large intestine (especially the rectum) of dogs. Lymphoma is most frequent in the small intestine but adenocarcinoma and sarcomas, including GI stromal tumors and leiomyosarcoma, also occur. Gastrointestinal stromal tumors are also seen in the cecum and large intestine. In cats, lymphoma is the most common intestinal neoplasm, primarily found in the jejunum and ileum, but adenocarcinoma and mast cell tumors also occur. Feline adenocarcinoma is an aggressive neoplasm that frequently metastasizes to lymph nodes and lungs and sometimes results in carcinomatosis [362–367]. Specific etiologies of GI neoplasia in animals have not been determined. Risk factors for GI lymphoma in cats include exposure to cigarette smoke, the presence of Helicobacter spp on biopsy, and infection with feline leukemia (FeLV) and feline immunodeficiency (FIV) viruses. Unlike other types of lymphoma, cats with GI lymphoma usually test negative for FeLV and FIV, but underlying retroviral infection is still suspect. Chronic inflammation as seen with GERD and inflammatory bowel disease, disruption of hormonal pathways as with gastrinomas, and chronic inflammation caused by infection (Spirocerca lupi, Helicobacter spp) are proposed underlying mechanisms. Hereditary predisposition to intestinal carcinoma is reported in Chow, Staffordshire Terrier, and German and Belgian Shepherd dogs, as well as in Siamese cats [363,364,366,368]. Esophageal neoplasia is rare in small animals; squamous cell carcinoma, fibrosarcoma, osteosarcoma, and leiomyoma are seen. Squamous cell carcinoma is most common in cats. Infection with S. lupi is reported to cause fibrosarcoma, osteosarcoma, and other undifferentiated sarcomas. Melanoma is seen in the esophagus; it is more common in canine breeds with black mucous membranes [362,363,369]. Rare neuroendocrine tumors (or carcinoids) arise from enterochromaffin cells found in the mucosa of the GIT. Previously called “amine precursor uptake and decarboxylation tumors” (APUDomas), carcinoids are rare in animals. Carcinoid syndrome occurs occasionally in humans; one report of ventricular tachycardia and melena in a Boxer dog was associated with an intestinal carcinoid [370]. Serotonin, histamine, substance P, various kinins, catecholamines, and prostaglandins are just some of the chemicals secreted by carcinoid tumors. Usually, these substances are transported to the liver and metabolized; however, if they gain access to the circulation or the lumen of the bowel, clinical signs of hemodynamic instability and pulmonary and GI dysfunction follow. Arrhythmias, hypo‐ or hypertension, vasodilation, bronchospasm, diarrhea, and hypersecretion of intestinal fluid may occur. Anesthesia for such tumors should avoid the use of drugs that encourage histamine release such as morphine, meperidine, atracurium, and succinylcholine. Dopamine, ephedrine, epinephrine, norepinephrine, histamine, and isoproterenol have been associated with carcinoid episodes in humans. Pretreatment with antihistamines and serotonin antagonists may be beneficial. Somatostatin receptors are found in some carcinoid tumors; the somatostatin analog octreotide is used to decrease secretion of serotonin and other substances. This has minimized clinical signs in humans and allowed the use of sympathomimetics under anesthesia [98,362]. Clinical signs of GI neoplasia usually reflect the area affected; malaise, weight loss, and anorexia may be the only signs. Vomiting, diarrhea, and melena are also common with gastric and small intestinal lesions. Regurgitation is frequent with esophageal disease. Tenesmus, hematochezia, and constipation occur with lesions of the rectum. Abdominal pain, distention, hemorrhage, obstruction, perforation, and intussusception also occur with GI neoplasia [362,363,365–367,369,370]. Mechanical effects of neoplasia may be seen with large tumors potentially compromising diaphragmatic excursion and causing or contributing to hypoventilation. Esophageal masses may affect respiration via pressure on the trachea. Anemia, hypoproteinemia, increased alkaline phosphatase, and increased BUN are the most common laboratory abnormalities seen with neoplasia of the GIT. Anemia can be due to multiple mechanisms including blood loss, lack of red blood cell production, or increased destruction of red cells. Protein‐losing enteropathy is common, causing hypoproteinemia due to hypoalbuminemia. BUN increases with chronic intraluminal blood loss due to recycling of protein [371]. Other enzyme changes are variable. White blood cell counts vary from normal to extremely elevated in patients with lymphoma and leukemia. Abdominal palpation may suggest the presence of neoplasia. Lesions are identified most often with radiography and ultrasonography. Aspirates and/or biopsies needed for definitive diagnosis may be obtained in conscious patients with ultrasound guidance; however, endoscopy and/or laparotomy under general anesthesia are sometimes necessary. Paraneoplastic syndromes (PNS) sometimes occur with GI neoplasia and can affect anesthetic management. PNS are caused by production of substances that affect tissues distant to the primary tumor. Numerous PNS exist, but not all patients exhibit signs of PNS. Some PNS are associated with a few particular types of neoplasia, others may be seen in many. A PNS may be the initial sign of cancer in some patients and lead to the diagnosis. Improvement of the PNS suggests a positive response to treatment, while recurrence is seen with loss of remission [372,373]. In addition to general clinical signs of GI dysfunction, weight loss is often seen with GI neoplasia. Although anorexia is an important cause of weight loss, cachexia is also significant. Cachexia is a loss of body condition in spite of a reasonable intake of calories. Decreased appetite is due to direct effects of neoplastic tissue but is also from inflammation that causes release of several cytokines, including interleukin‐1 (IL‐1) and interleukin‐6 (IL‐6), that affect the balance between appetite stimulation and inhibition. Cachexia is actually a destructive syndrome that alters fat and carbohydrate metabolism and interferes with growth and anabolic pathways to produce muscle wasting commonly seen in cancer patients. Effects are again produced by mediators of inflammation including tumor necrosis factor‐α, IL‐1, IL‐6, nuclear factor κB, and others. Even though some patients may actually be overweight at diagnosis, muscle wasting is often present and loss of body condition and weight continues with time. Both body weight and low body condition score have been associated with non‐survival in cancer patients [372,373]. Hypercalcemia of malignancy in dogs is common with T‐cell lymphoma (35–55%) and anal sac apocrine gland adenocarcinoma (25%) but is less frequent with GI adenocarcinoma. It is most common in cats with lymphoma, squamous cell carcinoma, and multiple myeloma. Secretion of parathyroid hormone (PTH) or a PTH‐related‐peptide affects pathways in bone and kidney, resulting in increased serum calcium and eventually mineralization of tissues, especially the kidney. Numerous other factors have also been identified that contribute to hypercalcemia of malignancy, including inflammatory cytokines and calcitriol. Osteolysis that occurs with lesions of bone also increases serum calcium [372,373]. Hypoglycemia is seen with leiomyoma/leiomyosarcoma, lymphoma, and GI stromal tumors. It can be due to secretion of insulin, but it is often from excessive use of glucose by neoplastic tissue, and interference with gluconeogenesis and glycogenolysis. Thrombocytopenia and/or coagulopathy are seen with hemangiosarcoma, lymphoma, and mast cell tumors. Histamine release from mast cell tumors and gastrinomas can lead to gastric ulceration and hemorrhage. Histamine release can also occur under anesthesia, causing vasodilation and hypotension. Although most common with renal tumors, erythrocytosis is reported with lymphoma and hepatic tumors. Mucous membranes are erythematous and polydipsia and neurologic signs can be seen. The elevated PCV complicates fluid therapy management, as patients are not necessarily hypovolemic or dehydrated and the PCV does not decrease as expected with fluids. Hyperglobulinemia is seen with all types of myelomas. Both erythrocytosis and hyperglobulinemia can cause hyperviscosity syndrome, which alters blood flow in tissues, resulting in inadequate delivery of oxygen and nutrients. Coagulopathy and damage to heart, brain, and ocular tissues may occur. Hypertrophic osteopathy is sometimes seen with esophageal tumors, thought to be caused by increased periosteal blood flow; the effects on bone can be extremely painful [363,369,372,373]. Anesthetic management for patients with GI neoplasia is similar to that described for laparotomy and hemoabdomen with attention to therapy for hypoproteinemia and PNS. Plasma and/or colloid administration may be needed when total protein is < 4.5 mg/dL or albumin is < 2.0 mg/dL. Infusion of human albumin has been used but severe side effects, including death, have been seen in some dogs [374–378]. Although not without side effects, canine‐specific albumin is available and is a better choice than human albumin [379]. Hypercalcemia may cause muscle tremors or weakness and arrhythmias, and can affect blood pressure. Correction of hypercalcemia in animals displaying clinical signs is beneficial prior to anesthesia; however, treatment is not pursued in asymptomatic animals with minimal hypercalcemia. Therapy to decrease calcium levels relatively quickly prior to surgery includes dilution via fluid therapy using 0.9% sodium chloride, which also encourages renal excretion, diuresis with furosemide, and calcitonin to inhibit osteoclastic activity in bone. Glucocorticoids can also be given; bisphosphonates are used for long‐term management [372,380]. Glucose should be monitored in patients with hypoglycemia and dextrose administered as needed to maintain serum glucose above 80 mg/dL. Intermittent monitoring of glucose is facilitated by use of an embedded monitor. This modality has been used under anesthesia to evaluate the completeness of surgical excision of functional tumors such as insulinoma [381]. See Chapter 42 for further information on glucose and calcium therapy. Treatment of anemia is described above in the hemoabdomen section. Patients with mast cell tumors should be premedicated with antihistamines and avoidance of drugs that stimulate histamine release, such as morphine and meperidine, is recommended. Mechanical effects on ventilation can be seen with obstructive intestinal lesions or large abdominal masses due to compromise of diaphragmatic excursions. Esophageal tumors can affect intubation and ventilation via pressure on the trachea. Paraneoplastic fever is not uncommon, especially with cancer of hematopoietic cells. The immune response to antigens presented by neoplastic cells as well as inflammation from necrotic tumors produces fever. Fever due to secondary infection may also occur in damaged tissue and must be distinguished from paraneoplastic fever. Effects of chemotherapy may also produce fever. Sepsis can occur with immunosuppression and peritonitis caused by GIT cancers [372,373]. Hemorrhage is common in patients with neoplasia and management is described above in the hemoabdomen section. Several authors have reviewed septic peritonitis in dogs [382–384], cats [267,385–389], or both [258,390–393]. It is categorized as localized or diffuse, and primary, secondary, or tertiary depending on the extent of disease and source of infection, respectively. Tertiary peritonitis is the recurrence or persistence of infection following treatment via surgery and antibiotics for an initial episode. Secondary peritonitis is most common in animals, due to perforation/leakage from the GIT. Foreign bodies, ulcers, obstruction, ischemic lesions, abscess/infection of organs or masses, trauma from motor vehicle impact, bite wounds, other penetrating injuries, and sometimes blunt trauma are causes. Some injuries may not break the skin but still damage intestine enough to cause leakage and induce septic peritonitis. Iatrogenic causes include dehiscence of intestinal anastamoses or incisions, inadvertent puncture of intestine, leakage around feeding tubes, and contamination of the abdomen at surgery. Spontaneous perforation of the GIT occurs occasionally with steroid or NSAID therapy. Aseptic secondary peritonitis is seen with leakage of urine, bile, gastric fluid, or pancreatic secretions. In primary septic peritonitis, an intra‐abdominal source of infection cannot be identified [386,387,390,391,393]. Variable mortality has been reported in small animals (31–69%) [267,383,387–389]. Patients with secondary peritonitis treated surgically have higher survival rates than those treated medically or those with primary peritonitis [267,392]. Pre‐existing peritonitis, low albumin and protein levels, and intraoperative hypotension were risk factors for postoperative septic peritonitis and death in dogs [383]. A study of mortality and dehiscence in dogs following GI surgery found that the rate of dehiscence was low at 5.3% (9/170); however, ASA status ≥ 3 and high lactate concentration were factors associated with dehiscence, septic peritonitis, and non‐survival [384]. A higher blood glucose level was associated with increased mortality in one study [389]. Early use of antibiotics was found to be associated with greater survival in a report of 83 cats with septic peritonitis [388]. Anorexia, lethargy, and vomiting are the most common clinical signs in dogs and cats. Diarrhea and abdominal pain are also seen in dogs; discomfort is reported but often vague or inapparent in cats. Signs of sepsis relate to the severity of abdominal contamination in most cases. Unlike dogs, bradycardia and hypothermia are frequently seen in cats with sepsis [385–387]. Diagnosis is suggested by history and physical examination, while abdominal radiographs and ultrasonography may reveal a specific lesion with or without effusion [394]. Pneumoperitoneum is diagnostic for intestinal leakage or infection with gas‐forming bacteria [391,392]. A septic infiltrate seen on cytology of peritoneal effusion or lavage fluid confirms the diagnosis of septic peritonitis. Increased lactate can be seen in serum and/or in the abdominal fluid. Peritoneal lactate and its comparison to serum lactate was diagnostic in ≥ 90% of dogs, but not in cats [395]. Although factors identified with prognosis vary, hypotension, hypoalbuminemia, and hyperlactatemia are consistently associated with mortality [382–384,386,387,392,393,395,396]. Patients with peritonitis are some of the most challenging cases presented for anesthesia. Clinical signs may suggest the presence of a septic focus, and confirmation may be obtained prior to laparotomy via diagnostics such as abdominocentesis, radiographs, and/or ultrasound. However, exploratory laparotomy can be a diagnostic as well as a therapeutic procedure. Septic patients are more sensitive to the depressant effects of anesthetic agents, so a thorough evaluation of the severity of cardiovascular compromise should be performed. Many cases are urgent or emergent, which may not permit a complete diagnostic evaluation; however, clinical signs and laboratory parameters have been evaluated in combination to allow early identification of sepsis. In 1991, human critical care physicians sought terminology to better define and describe inflammation and sepsis in critically ill patients. They agreed that systemic inflammation may occur with any severe insult, including trauma, hemorrhage, ischemia, and immune disease, as well as infection, and called it the “systemic inflammatory response syndrome” (SIRS). Sepsis was defined as SIRS in response to infection, most often bacterial, but fungal, protozoal, and viral infections also occur [397–400]. Criteria developed to identify SIRS included heart rate, respiratory rate or PaCO2, and temperature, combined with total white blood cell count (Table 41.5); evaluation of the mucous membranes was added later as an estimate of tissue perfusion. Sepsis was identified when two or more SIRS criteria were present in response to infection. Severe sepsis was present with poor perfusion, hypotension, and organ dysfunction. Septic shock was described as persistent hypotension and elevated lactate in spite of appropriate fluid resuscitation, requiring vasopressor therapy [398–405]. Some patients may be normotensive but exhibit signs of decreased perfusion such as cool extremities, poor capillary refill time and abnormal mucous membrane color, lactic acidosis, decreased urine output, and depressed mental status. While definitions have changed somewhat over the years, terminology has returned to more simplified versions in some respects. According to the “Sepsis 3” consensus conference in 2016 [401], “severe sepsis” has been abandoned, while sepsis is now defined as “life‐threatening organ dysfunction caused by a dysregulated host response to infection.” Septic shock remains as “persisting hypotension requiring vasopressors to maintain MAP ≥ 65 mmHg with a serum lactate level > 2 mmol/L despite adequate volume resuscitation” [398–402]. Table 41.5 Criteria for systemic inflammatory response syndrome (SIRS)/sepsis in dogs, cats, and humans. Source: Compiled from Bone et al. [397]; Nunnally [399]; Singer et al. [401]; Rank and Hansen [403]; Purvis and Kirby [404]; Hardie [405]. The pathophysiology of sepsis and septic shock is quite complex, and the knowledge base has vastly expanded since SIRS criteria were first developed. The body’s response to an insult is designed to ensure/restore oxygen delivery to vital tissues. Multiple systems participate in the inflammatory response, and although some cases may progress from SIRS to sepsis to multiple organ dysfunction syndrome (MODS), most do not proceed along a continuum. Early accounts describe an immune response triggered by organisms, their byproducts, and/or the resultant tissue damage, that then injures the patient as well. The primary components that trigger an inflammatory response are now called “pathogen‐associated molecular patterns” (PAMPs) and/or damage‐associated molecular patterns (DAMPs). PAMPs include the classic endotoxins and exotoxins from gram‐negative or gram‐positive bacteria and many other substances that alert innate immune cells to a foreign presence. DAMPs are endogenous structures/substances derived from damaged host cells and can arise from non‐infectious sources such as injury and toxins. Pattern recognition receptors interact with PAMPs/DAMPs to initiate the immune response, and are located inside or outside cells, in the bloodstream or body fluids. Pattern recognition receptors are located on neutrophils, macrophages, dendritic cells, platelets, complement, natural killer cells, fibroblasts, some epithelial cells including those of the GIT, and many others. Signaling pathways are triggered that result in activation of multiple genes to produce pro‐ and anti‐inflammatory effects. If the source is eliminated, the immune response subsides and saves appropriate information for future insults. However, with sepsis, “dysregulation” develops. Normal intrinsic responses are uncontrolled and proinflammatory effects overwhelm anti‐inflammatory activities; actual suppression of the immune system also occurs [406]. A myriad of inflammatory mediators is involved, including tumor necrosis factor‐α, interleukins, leukotrienes, prostaglandins, cytokines, various peptides, the potent vasodilator nitric oxide (NO), oxygen radicals, and platelet‐activating factor. Any/all organ systems may suffer, but in addition to the source location, the cardiovascular, respiratory, neurologic, and hemostatic systems are most profoundly affected. The net result is hypotension caused by vasodilation and a relative or absolute hypovolemia with shifting of volume to the venous side of the circulation. Damage to the glycocalyx occurs, causing loss of endothelial barrier function. Fluid leaks into cells and the interstitium, resulting in edema, cellular damage, and protein loss. Normal or increased cardiac output is present initially, but myocardial contractility is eventually impaired. Coagulopathy also develops that contributes to tissue ischemia. Adrenal insufficiency and decreased endogenous vasopressin levels also exacerbate hypotension. The net result is septic shock, also called “vasoplegic” or “distributive shock,” with hypotension in spite of appropriate fluid resuscitation. Vasopressor therapy is required as well as inotropic support for cardiac dysfunction [255,398–400,402,407]. The GIT is the most common source of sepsis in animals and is also the “shock organ” in dogs. However, it plays a role in the generation of SIRS/sepsis even when the intestinal serosa is intact or the primary source is elsewhere. Inflammatory damage to the barrier functions of the GI mucosa allows absorption of non‐microbial factors into the lymphatic system, which then drain into the venous system via the thoracic duct. Ongoing SIRS is magnified, and respiratory dysfunction/distress and multiple organ failure may ensue via this pathway. Translocation of bacteria and bacterial products also occurs in some patients and is also thought to occur via lymphatics rather than the portal system. However, bacterial translocation is not necessary for the development of SIRS and MODS [408,409]. There is also evidence that alteration of the GI microbiome contributes to sepsis pathophysiology as well [410]. Although early identification of SIRS/sepsis is vital to survival, SIRS is a clinical diagnosis. Signs are not always obvious in animals, especially cats, and may overlap with non‐septic illness. Anaphylaxis causes similar signs with abnormal mucous membrane color, hypotension, altered mentation, and so on and must be differentiated from sepsis [349,411]. In humans, the SIRS criteria were ultimately considered inadequate for many patients, and other scoring systems were developed. The Sequential Organ Failure Assessment (SOFA) score is used to screen patients for organ dysfunction. It includes measures of neurologic, cardiovascular, renal, hepatic, and hematologic function. A shorter variation, the quick‐SOFA (qSOFA), eliminates laboratory data and focuses on mental status, low systolic blood pressure, and increased respiratory rate. The Acute Patient Physiologic and Laboratory Evaluation (APPLE) and its modification, fastAPPLE, were developed to be used in hospitalized patients, and include more laboratory parameters [402,412]. Other scoring systems exist that apply to specific patient categories, but all are designed to help identify sepsis, assess the severity of illness, and infer risks of morbidity as well as mortality. SIRS criteria, SOFA, APPLE, and modifications have been adapted and used in critically ill dogs and cats [385,398,402,413–419]. They have all been shown to perform well in some groups but no scoring system is 100% reliable. It is not recommended to rely on one scoring system alone to infer the presence of sepsis [402,403,417,418,420]. However, their use prompts clinicians to look more closely when a septic source is not obvious and enables discussions of prognosis and survival with owners and other veterinarians. In 2002, the Surviving Sepsis Campaign (SSC) [421] was launched globally to educate medical professionals and governments about the increasing incidence of sepsis and offer guidelines for identification and treatment. It also recommended methods for early diagnosis and provided guidance for management of these difficult cases. Updates have been published periodically [421,422]; veterinary recommendations are similar [326,404], but further study is needed to determine specific protocols for animals. The SSC promotes guidelines as care “bundles.” According to 2021 SSC guidelines [420,421], the initial bundle should be instituted within 1 h of the recognition of sepsis (Box 41.2). Collection of samples for blood cultures is performed prior to administration of broad‐spectrum antibiotics, which should also be given within the first hour. Fluid resuscitation should begin immediately with intravenous isotonic crystalloids. Diagnostics to identify an infective source should begin as soon as possible, and lactate should be measured at admission and repeated intermittently to evaluate response to therapy. Goals of initial therapy should be achieved within 3 h (Table 41.6), with central venous oxygen saturation (ScvO2) > 70%, urine output > 0.5 mL/kg/h, central venous pressure of 8–12 mmHg, and mean arterial pressure (MAP) of > 65 mmHg. This MAP is lower than previous recommendations, as no difference in outcome was seen when a higher pressure was used. Measurement of mixed venous oxygen saturation (Sv̅O2) requires catheterization of the pulmonary artery, but the use of the central venous oxygen saturation (ScvO2) from the cranial vena cava is an acceptable alternative [423]. Lactate can also be used to estimate oxygen delivery when central catheterization is not available [420,423].
41
Physiology, Pathophysiology, and Anesthetic Management of Patients with Gastrointestinal Disease
Anatomy of the gastrointestinal tract
Effects of anesthetic agents on gastrointestinal function
Nausea and vomiting
Antiemetic therapy
Gastroesophageal reflux
Decrease LES tone
Increase LES tone
Nitric oxide and nitrates
Prostaglandin E
Vasoactive intestinal peptide
Muscarinic M2, M3 receptor agonists
Nicotine
Gastrin
β‐Adrenergic receptor agonists
Substance P
Dopamine
α‐Adrenergic receptor agonists
Cholecystokinin
Prostaglandin Fα
Secretin
Motilin
Calcitonin gene‐related peptide
Adenosine
Decrease LESP
Increase LESP
No Change
β‐Adrenergic agonists
Acepromazine
Alfaxalone–alfadoloneb
Aminophylline
Atropine, glycopyrrolate
Benzodiazepines
Calcium channel antagonists
Change from lateral to dorsal recumbency
Cisapride
Cricoid pressure
Dexmedetomidine (high doses)
Dorsal versus lateral recumbency
Fentanyl–droperidol
Hiatal hernia
Inhalant anesthetics
Ketaminec
Laryngeal mask airway
Meperidine
Morphine
Nitroprusside
Nitrous oxidea
Obesity
Oxymorphone
Pregnancy
Propofol (high doses)
Residual neuromuscular blockade
Thiopental
Tricyclic antidepressants
Xylazine
Xylazine–ketaminec
α‐Adrenergic agonists
Acetylcholine
Antacids
Anticholinesterases
Domperidone
Edrophonium
Gastric acidification
Histamine
Increased gastric or abdominal pressure
Metoclopramide
Neostigmine
Pancuronium
Succinylcholine
Vecuronium (small increase)
Atracurium
Dexmedetomidine
Histamine (H2) antagonists (cimetidine, ranitidine)
Metoprolol
Nitrous oxidea
NMBA reversal with neostigmine and glycopyrrolate
Propofol
Propranolol
Proton pump inhibitors
Remifentanil
Increased incidence of GER
No difference
Decreased incidence of GER
Abdominal procedures
Brachycephalic breed
Dorsal recumbency
Esophageal disorders,dysmotility
Gastric acidity
Hiatal hernia
Imaging studies
Large deep‐chested dogs (spinal surgery)
LMA compared to ET tube (kittens)
Longer anesthetic duration
Medetomidine compared to acepromazine–opioid
Morphine (increased incidence with higher doses)
Neurologic surgery
Older dogs, sicker dogs (ASA ≥ 3)
Orthopedic surgery
Prolonged fast
Propofol compared to thiopental
Shorter fasting
Decreased fasting times/small meal
Halothane versus isoflurane versus sevoflurane
Recumbency or head tilt
Standard fast compared to short fast (17.3 h versus 4.7 h)
Vomiting following premedication
Dexmedetomidine–hydromorphone compared to acepromazine–hydromorphone
Diazepam compared to atropine–propionylpromazine
High‐dose metoclopramide infusion
Meperidine compared to morphine
Short fast (3 h versus 10 h)
Factors associated with increased incidence of GER in humans
Altered consciousness
Full stomach
Late pregnancy
LMA (some types)
NSAID use
Other factors
Dogs that reflux early more often regurgitate
Increased gastric pH, no difference with gastric volume
Prolonged fasting increases gastric acidity
Type, volume of food
Esophagitis and esophageal stricture
Aspiration
Gastrointestinal motility/postoperative ileus
Goal
Interventions
Maintain intestinal blood flow and oxygenation
Fluid therapy – goal‐oriented, restricted (avoid excessive volume)
Avoid saline, use balanced solutions ± colloids
Prevention, prompt treatment of hypotension
Prevention, treatment of low protein, albumin
Maintain oxygen therapy, use higher FIO2 throughout
Perioperative analgesia
Systemic opioid‐sparing protocols
Thoracic epidural with local anesthetic – block sympathetic input
Epidural morphine – less inhibition of motility
Peripheral nerve blocks – transverse abdominis plane block
NSAIDs – COX‐2 inhibitorsa – reduce inflammation
Lidocaine infusion – anti‐inflammatory, less/shorter POI
Dexmedetomidine infusion postoperatively
Electroacupuncture at ST36
Surgical techniques
Minimize handling of intestine
Laparoscopy whenever possible – less inflammation, shorter POI
Postoperative care
Maintain oxygenation and blood volume/arterial blood pressure
Enteral nutrition and ambulation as soon as possible
Avoid nasogastric tubes
Laxative therapy to treat, prevent constipation
Prokinetic medication/therapeutics
Alvimopan,
Methylnaltrexone
Peripheral opioid receptor antagonists
Erythromycin,
Azithromycin
Motilin/ghrelin receptor agonists
Metoclopramide
Dopamine (D2) receptor antagonist, serotonin receptor agonist (5HT4)
Domperidone
Dopamine (D2) receptor antagonist
Ranitidine,
Nizatidine
H2 receptor antagonists
Mosapride,
Prucalopride,
Cisapride
Act on serotonin receptors
No longer used in people but is used in animals, compounded
Anti‐inflammatory and prokinetic
Daikenchuto – Japanese herbal preparation; ginger, ginseng, zanthoxylum fruit
Gastrointestinal conditions requiring anesthesia
Laparotomy
Megaesophagus
Gastric dilation–volvulus
Hemoabdomen
Intestinal neoplasia
Septic peritonitis
Parameter
Dog
Cat
Human
Heart rate (beats/min)
> 120
< 140 or > 225
> 90
Respiratory rate (breaths/min)
> 40
> 40
> 20
or PaCO2 (mmHg)
< 30
< 32
Temperature (°F)
< 100.4 or > 104.0
< 100 or > 104
< 96.8 or > 100.4
Leukogram white blood cells/μL
or % immature band cells
> 18,000 or < 5000
> 19,000 or < 5000
> 12,000 or < 4000
> 5%
> 5%
> 10%
Prolonged CRT, abnormal mucous membrane color
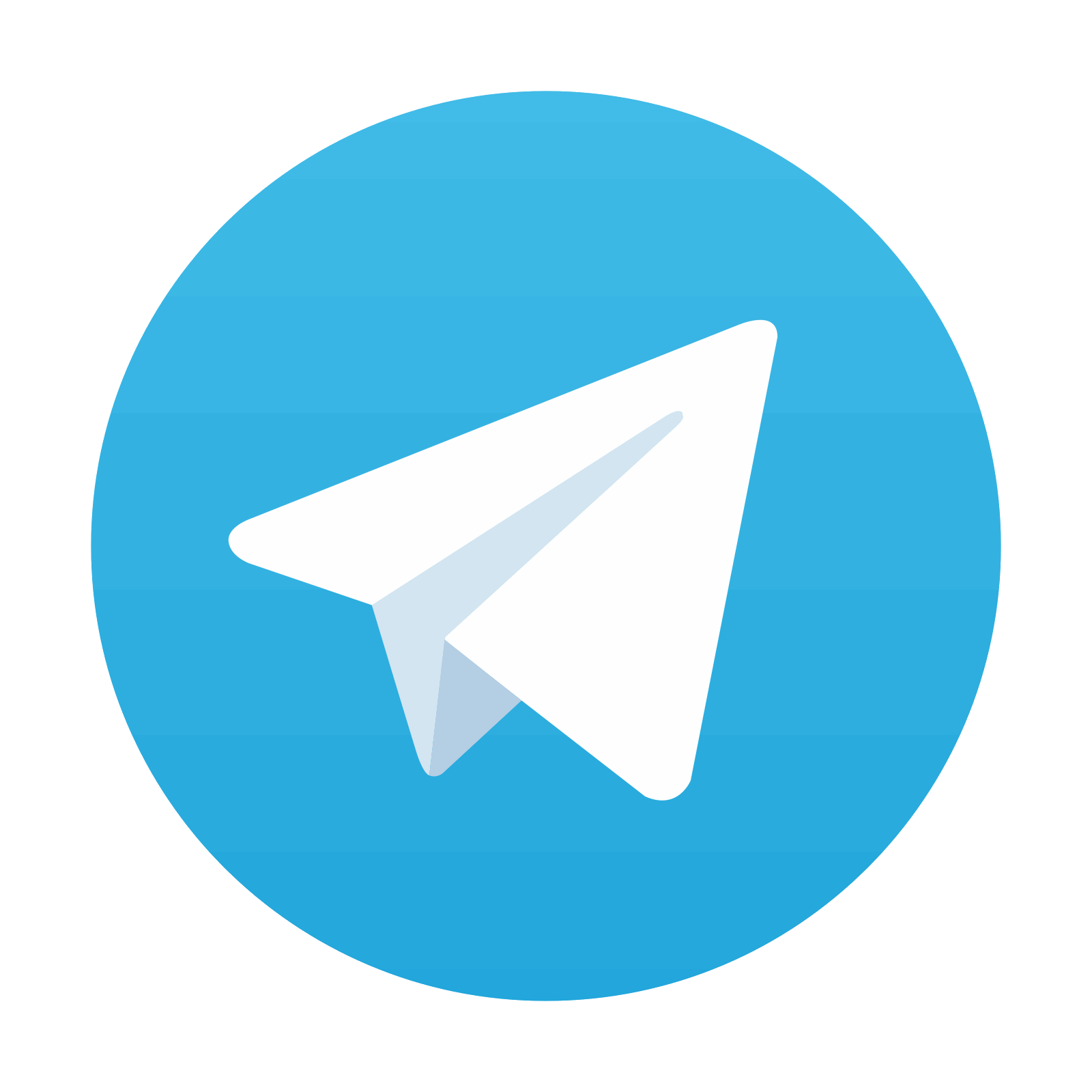
Stay updated, free articles. Join our Telegram channel

Full access? Get Clinical Tree
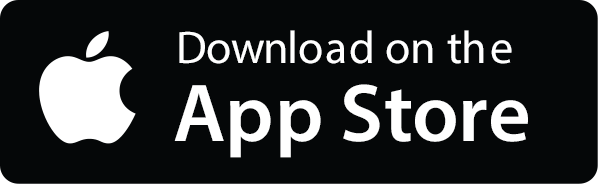
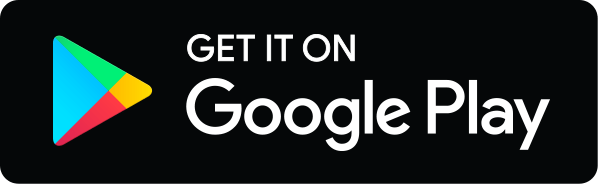