Parameter
WKY
SHR
Body weight (g)
513 ± 15
396 ± 4*
Mean blood pressure (mmHg)
116 ± 2
171 ± 3*
PH
7.47 ± 0.02
7.46 ± 0.02
pCO2 (mmHg)
37 ± 1
37 ± 3
pO2 (mmHg)
98 ± 5
92 ± 5
Blood glucose (mg/dl)
155 ± 7
169 ± 6
Body temperature (°C)
37.3 ± 0.1
37.0 ± 0.1
Hematocrit (%)
47 ± 1
50 ± 1
Osmolality (mOsm/kg)
288 ± 3
295 ± 4
Over the last decade, the “classic” physiological techniques, such as hemodynamic monitoring, blood flow analysis, and others have been challenged by molecular approaches to physiology as well as large-scale “functional” techniques, such as FDG-PET or NMR spectroscopic studies of metabolism. We agree that these are excellent tools and they shed light on important basic and clinical phenomena. However, although such techniques are being increasingly utilized in both basic and clinical research, the generated data is not a surrogate for standard physiological variables. Furthermore, given their limited practicality and limited availability in most clinical and basic research settings, these highly specialized techniques are usually limited to few specialized academic centers and rarely impact the standard care of stroke patients. This chapter focuses on those common physiologic techniques used in both basic science studies and in the clinical care of ischemic and hemorrhagic stroke. We cover techniques of blood pressure and heart rate monitoring, cerebral blood flow, temperature monitoring, and a collection of basic laboratory studies.
2 Blood Pressure Measurement
Blood pressure monitoring during the induction of experimental stroke in animal models is critical due to its direct impact on cortical infarct size, development of edema, and the overall neurologic outcome. The monitoring and treatment of blood pressure changes are an area of specific focus in the American Heart Association and American Stroke Association guidelines for both acute ischemic infarction and intracerebral hemorrhage (15, 16). Thus, determining blood pressure throughout an experimental stroke study (before, during, and after stroke induction) is a key component of reducing infarct variability and also maintaining ecological validity.
Blood pressure fluctuates throughout the day in most laboratory animals due to diurnal variations (Table 2). In most nocturnal rodent species, the peak arterial blood pressure is typically observed both early and late during the dark period, with nadir occurring mid-way through the light period (17). This is likely due to the systemic adjustment to metabolic demands of foraging activity and food intake. The effect of ambient temperature on cardiovascular parameters, including blood pressure, must also be considered. In mice, as the temperature decreases by 1°C, blood pressure and heart rate increase by 1.6 mmHg and 14.4 bpm, respectively. Rats have a similar response with an increase in blood pressure and heart rate of 1.2 mmHg and 8.1 bpm for every 1°C decrease in ambient temperature (18).
Table 2
Heart ratea and blood pressure# measured during various activities in mice
Activity | Heart rate | Systolic | Diastolic | Mean arterial |
---|---|---|---|---|
Sleeping | 350–450 | 102–112 | 70–80 | 86–96 |
Resting (awake) | 450–500 | 110–117 | 75–85 | 93–103 |
After light activity | 600–650 | 126–138 | 94–109 | 110–124 |
During weighing | 700–750 | 140–155 | 105–120 | 123–138 |
During restraint | 750–800 | 140–155 | 105–120 | 123–138 |
After placement in new cage | 750–800 | 140–155 | 105–120 | 123–138 |
Light | 344–358 | 119–122 | 86–88 | 97–99 |
Dark | 402–418 | 124–125 | 91–92 | 102–104 |
Experimental stroke models, in contrast to the usual management of human stroke, must account for the effects of anesthesia and any trauma that is incurred due to the technique used in each particular stroke model (19). Blood pressure often fluctuates due to type and depth of anesthesia as well as the secondary effects on other parameters, such as core body temperature (17). The effects of anesthesia may also blunt the normal physiologic response to stroke, which includes elevation of blood pressure in order to maintain cerebral perfusion pressure. The alteration of systemic blood pressure impacts regional cerebral blood flow, especially in the peri-infarct area where autoregulation is impaired. The impact of physiologic parameters, especially blood pressure, on cerebral blood flow is related to outcomes in experimental stroke studies as well as in human clinical trials (20).
In experimental stroke studies with small rodents, the two most commonly used techniques for blood pressure monitoring are the indirect tail cuff method and direct arterial pressure measurement through an exteriorized catheter and pressure transducer. The use of an implantable radiotelemetry system has been validated and has gained popularity over the past few years, offering a third option (21, 22).
2.1 The Tail Cuff Method
The tail cuff method offers an indirect and noninvasive approach to blood pressure measurement. The method is often very efficient and computerized applications that accompany commercially available systems can measure and record numerous data points over time with minimal training and technical understanding. The technique has been validated in comparison to radiotelemetry (23) and carotid artery catheterization with some minor discrepancies. For example, tail cuff measurements tend to be less reliable at the extremes of both hypotension (<110 mmHg) and hypertension (>180 mmHg) in the mouse (23, 24). Further studies in rats suggest that the variability is greater than that seen in mice, with at least one study demonstrating a 37 mmHg difference between tail cuff and arterial cannulation with significant variability from reading to reading (17, 25). Additional drawbacks to this approach include the need to minimize stress and stimuli which induce movement (by training animals in the restrainers) as well as the need to maintain tail vasodilatation. Devices that minimize movement and provide a warmed surface are available with most systems. The need to maintain vasodilatation does present limitations in studies of hypothermia as well as those experiments involving vasoconstricting pharmacologic agents.
Technique
Animals can be either awake or anesthetized
Holders are of different sizes and can hold animals weighing anywhere between 8 and 950 g. Up to eight animals can be recorded at the same time with some devices. Some investigators prefer to train animals in the restrainers a few days prior to the experiment day to reduce stress.
Gently place animals in the restrainers and properly fix their noses on the nose cone holders at least 10–15 min before measurements to allow time for acclimatization
The tail should be fully extended and should exit through the rear hatch opening of the holder
Secure the cuff around the tail
A computerized system will record and display several pressure parameters like systolic, diastolic, and mean BP, heart rate, tail blood volume, and blood flow
2.2 Direct Arterial Blood Pressure Measurement Using Exteriorized Catheters
Exteriorized catheters present a direct interrogation of arterial (or venous) blood through direct cannulation of the vessel. The two most common methods are the use of indwelling fluid-filled catheters and transducer-tipped catheters. Exteriorized catheterization is reliable in most experimental approaches and offers a route through which pharmacologic agents can be delivered and blood samples collected. This is an invasive procedure that has significant risk for mobidity, depending on the vessel that is cannulated. It also requires microsurgery skills and can prove quite difficult in smaller rodents where vessel cannulation is a challenge. Additionally, there is the increased risk for infection and complications of catheter thrombosis. The effects of this invasive procedure on the physiology of the animal must also be considered, especially in studies requiring consciousness (26).
Technique
Appropriately anesthetize the animals
Place catheters (PE 10 tubing for mice and PE 50 tubing for rats) in the femoral artery for arterial pressure measurements and in the femoral vein for blood sampling and infusions
Tunnel catheters subcutaneously and exteriorize through a spring which can be attached to the back of the animal and eventually to a swivel device at the cage top
Give animals 5 days to recover before collecting any measurement and check continuously for signs of distress, pain, or infection at the catheter site
Arterial pressure is measured with a pressure transducer and amplified. Data is collected and displayed on a computer program
2.3 Radiotelemetry
Radiotelemetry offers a direct approach to measure blood pressure as well as other physiologic parameters like temperature in a conscious and freely moving animal. This technique has been validated (21) and measurements correlate well with other direct methods. This approach offers the additional benefit of overcoming the effects of anesthetics and continued stress induced by restricting movement in order to maintain externalized catheters. Radiotelemetry does require microsurgical skills and have a small risk of morbidity associated with surgery as well as the continued presence of a foreign object retained within the animal. For more detailed technical descriptions of this technique, please refer to refs. (26) and (27).
Technique
Appropriately anesthetize the animals
Advance the catheter of a telemetric pressure transducer to the aortic arch through the common carotid artery
Secure the transmitter in the abdominal cavity
Give animals 5 days to recover before any measurement and check continuously for signs of distress, pain, or infection
Place receiver close to the animal cages
Measurements are collected and displayed using specialized receivers and software as determined by the manufacturer
3 Temperature Measurement
The regulation of body temperature in experimental stroke models is necessary to limit the neurologically damaging effects of hyperthermia. Hyperthermia is common after stroke and worsens outcomes. In contrast, hypothermia has a well-established neuroprotective effect and serves as a potential confounder if it is not accounted for during experimental studies. Transient hypothermia is also common after stroke, although more so in experimental studies as a transient side effect of anesthetics used for the surgical procedure. Currently, maintaining normothermia in experimental stroke models and in human stroke care is the standard (15, 16). In human stroke care, normothermia is typically maintained with acetaminophen or ibuprofen by their downregulation of the cyclo-oxygenase pathways (28) or with the application of surface cooling or warming as needed.
In small animals, thermoregulation is more challenging due to their large body surface area to mass ratio. As basal metabolic rates are inversely proportional to body mass, small animals tend to heavily depend upon their basal metabolic rate to maintain normothermia. A typical laboratory mouse with a mass of 30 g has a basal metabolic rate approximately 13 times greater than a 450 kg thoroughbred horse per gram of tissue (17). Thus, attention must be paid to maintain body temperature in the laboratory setting, where the often anesthetized animal is not able to meet the metabolic demands needed to maintain normothermia.
Most experimental stroke models involve surgical procedures with an anesthetized animal. The perioperative period involving removing the animals from their cages, administering anesthesia and the postoperative period often introduce opportunities of temperature dysregulation. These alterations in temperature are often unintentional and typically go unrecorded. Monitoring temperature before, during, as well as after the procedure ensures that there are no unexpected extremes in temperatures which may confound the results of the experiment.
In experimental stroke studies, monitoring core body temperature is often taken as a surrogate for the value of most interest—brain temperature. Monitoring rectal temperature in this setting assumes that there is a high degree of correlation between the brain and body temperatures—whether measured by rectal or intra-abdominal recording. Colbourne and colleagues (29, 30) have described the discordance between brain and core body temperatures, indicating that rectal temperature does not clearly and faithfully predict brain temperature. In a review of 15 studies comparing core to brain temperatures, all 15 studies found that brain temperature was higher than all measures of core temperature with a mean difference of 0.39–2.5°C (31). Moreover, there is evidence that the use of rectal temperature probes actually induces a rise in body temperature most likely due to stress-induced mechanisms (32). Therefore, the decision to monitor temperature in experimental stroke studies must determine whether core body temperature monitoring will suffice or if monitoring brain temperature is a necessary aspect to increase validity. Experiments in which temperature is a component of therapy, in hypothermia studies for example, may have a lower threshold for including brain temperature recordings in their experimental design.
There are generally two aspects to temperature regulation in experimental stroke research: monitoring and maintaining temperature.
3.1 Monitoring Temperature
Various methods are available for monitoring body temperature in experimental stroke models. The most common and simplest approach is the use of a rectal temperature probe. A wide variety of model and size variation exists and most modern thermistor probes can be connected to computers for continuous real-time monitoring and data collection. Radiotelemetry devices offer a solution to many of the drawbacks of conventional temperature monitoring. These implantable data collecting devices are implanted intraperitoneally or subcutaneously and allow collection of temperature data in freely moving animals, free of the effects of anesthetics (25). Brain probes also exist to directly monitor the temperature of the brain parenchyma (29).
3.1.1 Rectal Temperature
Temperature monitoring by a rectal probe is easy and efficient. There are few drawbacks or difficulties with this method. If the technique is standardized, repeated measurements show little variability. However, repeated measurements can actually increase body temperature, likely due to a stress–response (32). Additionally, there is the potential for colonic injury and, rarely, bowel perforation. Appropriate probe diameter and insert distances are important to reduce risk of damage and of inducing an inflammatory response. Our lab uses a standard of 2-cm insert distance using a Rectal Thermocouple Probe for mice (model MLT1404—Harvard Apparatus) or 3-cm insert distance using a Rectal Thermocouple Probe for Rat (model MLT1403—Harvard Apparatus).
Technique
Ensure calibration of probe by direct comparison with a digital or mercury thermometer in a water bath.
Apply a commercially available water-based lubricant to the rectal probe prior to insertion.
Remove animal from cage.
Insert the probe 2 cm (mouse) or 3 cm (rat) into the rectum.
Record for a minimum of 20 s or until a stable value is reached.
3.1.2 Brain Temperature
There are several methods for directly monitoring brain temperature. The first involves a less invasive “pericranial” approach. Needle thermocouple probes (e.g., MLT1406, Harvard Apparatus) offer a quick, easy approach to obtaining brain temperatures. In this technique, the needle probe is inserted into the temporalis muscle, adjacent to the skull. Measurements, however, are highly sensitive to animal or probe movement and do not give an accurate recording of intracerebral temperature (33).
For direct measurement of temperature at the level of the brain, thermistor probes can be directly inserted into the brain parenchyma. Most new systems utilize telemetry approaches for long-term monitoring; especially, if brain temperatures are to be monitored in the awake and freely moving animal. For brain temperature monitoring in animals that will remain anesthetized, a similar approach can be used with a needle thermocouple device. Both of these approaches require microsurgery skills and detailed knowledge of brain and skull anatomy. Furthermore, the surgical procedures are invasive and complex by their nature. They also introduce the risk of infection and unintentional brain injury. In some large territory stroke models, introducing a temperature probe does increase the risk of mortality.
Technique
For a pericranial approach to brain temperature monitoring:
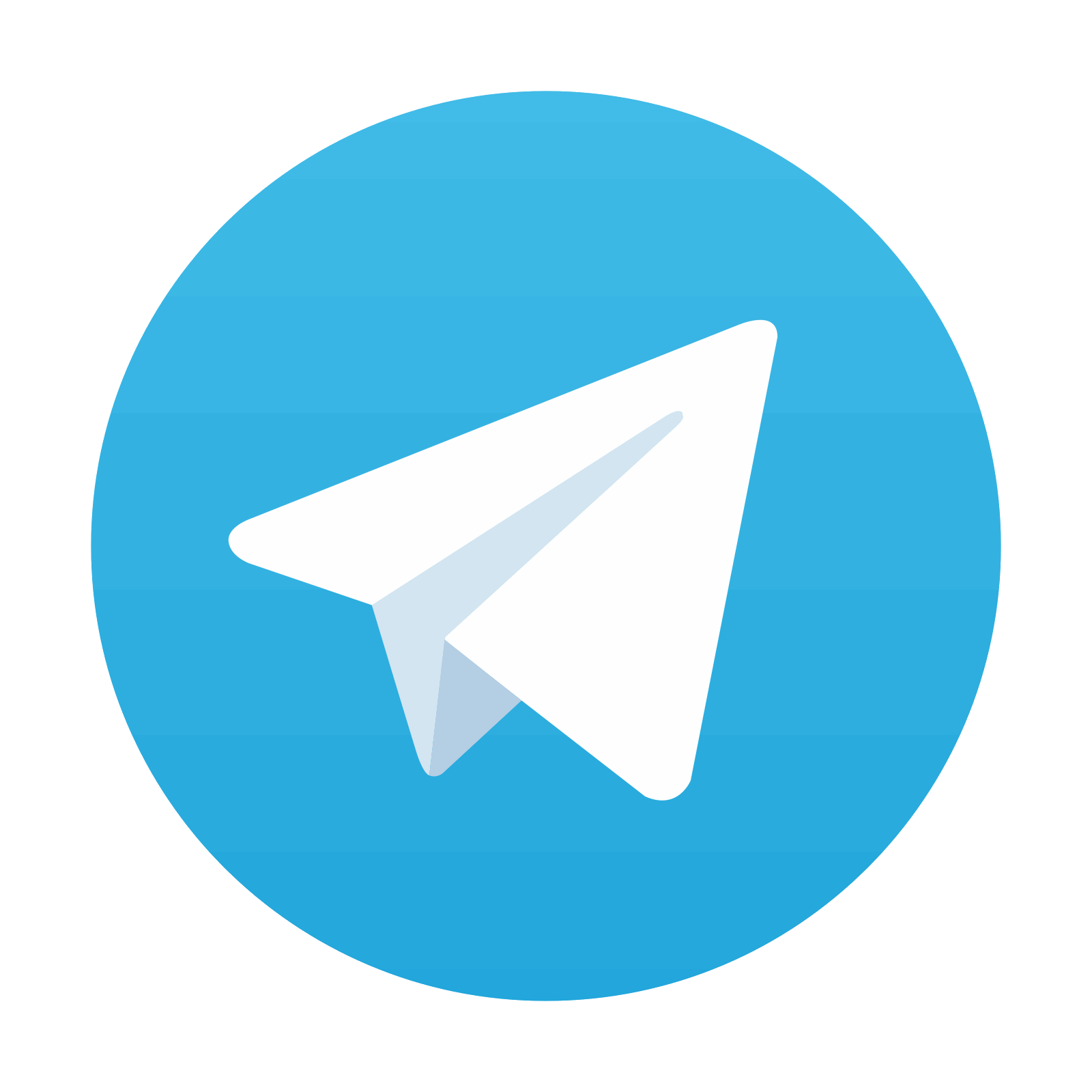
Calibrate the needle thermocouple probe (e.g., MLT1406, Harvard Apparatus) at two temperatures at the upper and lower limits of expected range (e.g., 33°C and 38°C) in a water bath. Calibrate at the beginning of the day before experiments start and any time after power has been turned off or batteries have been changed.< div class='tao-gold-member'>Only gold members can continue reading. Log In or Register a > to continue
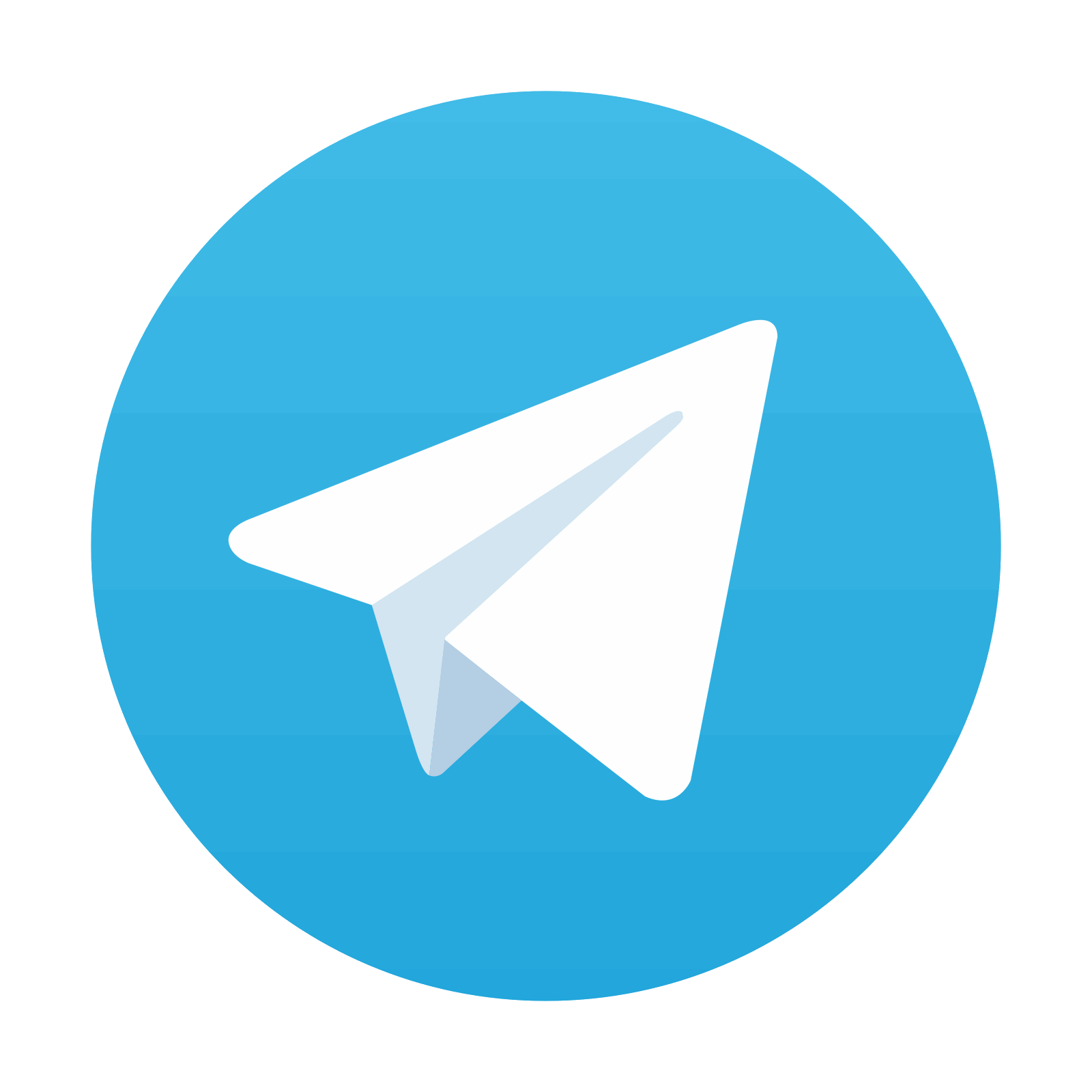
Stay updated, free articles. Join our Telegram channel

Full access? Get Clinical Tree
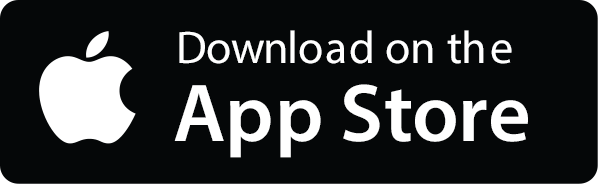
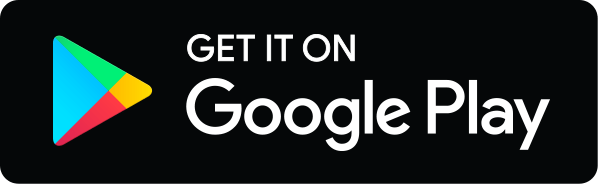
