Chapter 8 Pharmacologic intervention in behavioral therapy
Introduction
The timely and appropriate use of drugs may allow the pet owner an opportunity to resolve the pet’s behavior problem successfully, or modify its behavior sufficiently to allow the pet to remain in the home. Behavior problems can be very disruptive for the family and the people living nearby, often to the point of severely affecting the human–animal bond. Thus, the quick and effective control of clinical signs is usually one of the main goals of behavioral treatment protocols. Drugs are one of the main adjunctive treatments to achieve that objective. Failure to identify and suggest potentially helpful pharmacological agents may mean the difference between a safe and healthy pet–owner relationship and the pet’s demise. Drug selection requires an accurate diagnosis of the behavior problem and a comprehensive knowledge of which drug(s) would be the safest and most effective for resolving the problem at hand (Box 8.1).
Box 8.1
Considerations prior to prescribing or dispensing medications
1. Complete medical workup/premedication baseline
2. Accurate behavioral diagnosis
4. Potential side-effects and adverse effects
6. Expected time to efficacy/improvement
7. Monitoring requirements – when, what tests, how often?
8. Need for concurrent behavioral and environmental management
9. Severity of the problem, owner’s ability to tolerate the problem, immediate danger to pet and others, need for quick control to avoid rehoming or euthanasia
Drug prescribing must proceed in agreement with local regulations and licensing requirements. Since most drugs used in canine and feline behavior therapy are not licensed for use in pets, they should be used cautiously. Whenever possible, medications licensed for use in the species and for the intended purpose should be used. For off-label use (use not specifically indicated on the product label) or compounded medications, the hospital should ensure full disclosure and the owner should sign a release where appropriate (see Appendix C, form C.9, client form #5, printable version available online), indicating informed consent for the use of a product not licensed for this purpose. However, a release does not absolve the practitioner from liability, particularly if the rationale for selection and use of the drug cannot be medically justified. Caution should be taken to assess carefully whether any concurrent medication, supplements, or diet is being utilized that in any way interact with the medication that has been selected. Of course, owner compliance, including ability to administer the medication, may also impact the choice of medication and whether compounding might be necessary (see below). Owners should be advised as to what behaviors are likely to be improved (i.e., intensity, frequency, severity) and over what time frame, and what side-effects or adverse events might be expected. Although the family should be advised to report any unexpected change in health or behavior immediately, the veterinary clinic should be proactive in regularly contacting the client to assess progress or potential problems. Veterinary literature should be regularly reviewed for reports of adverse effects or changes in dosage recommendations. Although human studies cannot necessarily be extrapolated to animals, it is also advisable to consult the human literature and manufacturers’ data to determine areas of potential concern. Ideally, blood and urine tests should be performed before any behavioral drug is dispensed to rule out underlying medical problems and establish a baseline against which future tests can be measured. Testing should be repeated at regular intervals (minimally once a year) based on the pet’s age and health and the potential side-effects of the medication being used. When there is more than one potentially effective treatment regimen, the safest course of action should be followed (Box 8.1).
Evidence-based medicine and veterinary behavioral pharmacology
Most available information on drug therapy for behavior problems in companion animals comes from clinical experience of veterinary behaviorists as well as from inferred comparisons between psychiatric conditions and behavior problems of pets. According to the Oxford Centre for Evidence-Based Medicine scoring system (www.cebm.net), the weakest levels of evidence for measuring therapeutics (levels 4 and 5) are attributed to case-based studies, in vitro research, or expert opinion without crucial appraisal. A systematic review of case-control studies with homogeneity or individual case-controlled studies would receive a higher rating in terms of evidence (level 3), while a systematic review of cohort studies, individual cohort studies, and lower-quality randomized control trials (RCTs) would receive level 2 ratings. The gold standard (level 1a) would be a systematic review with homogeneity of RCTs.1 However, with few published trials for most veterinary behavioral therapeutics, veterinarians have little access to such rigorous research. Whenever possible, it is clear that RCTs should be the standard way to assess efficacy of drug therapy in behavioral medicine, particularly regarding the placebo effect, which may be responsible for 50% or more of the effects in some behavioral studies. For instance, in a study in which fluoxetine was compared to placebo in the treatment of separation anxiety, the effect of treatment on global improvement ranged from 58.6% to 65.1% while the placebo group had improvements ranging from 43.4% to 51.3%.2 In a recent study in which a homeopathic remedy for firework phobias was compared to placebo for the treatment of noise sensitivities, both treatment groups reported a significant level of change over the course of treatment but there was no evidence that the homeopathic remedy had any effect above the control group.3,4 In addition, knowledge of participating in a placebo-controlled trial appeared to have no effect on the owner’s perception of treatment effect.4
In prior periods, the amount of published independent clinical trials on psychopharmacology of behavior problems in companion animals was sparse. One of the main limitations for conducting proper controlled clinical trials is the ethical concern of setting up a pure placebo group on a population of owned dogs presented for behavior problems. Fortunately, over the past few years, more and more clinical trials have been conducted on drug treatment of behavior problems of dogs and cats. For example, the vast majority of available studies on the use of fluoxetine to treat canine behavior problems have been published since the year 2000. Recently, a systematic review of RCTs examining the effects of therapeutic agents on urine marking in cats has been published, representing the highest level of evidence for the use of those drugs to treat that particular condition.5
Recently laboratory models have been developed for a variety of behavior problems, allowing for the assessment of drugs, natural supplements, and behavior products in a controlled environment, including minimal subject variability, validated measures of behavior, and removal of owner bias. An example of this approach is the work done by CanCog Technologies (cancog.com) to develop a variety of validated models for: (1) learning and memory tasks in dogs and cats with specific applications for cognitive dysfunction and brain aging (see Chapter 13); (2) fear of noises6; and (3) fear of humans.7 These models have proven invaluable in validating the efficacy of a number of diets, natural supplements, and drugs that are now licensed for use in dogs and cats. Nevertheless, while laboratory models are an effective means of supporting the efficacy of a product over a placebo or control group, clinical trials in affected pets are necessary to support the efficacy of the product for specific behavioral applications in a real domestic environment.
Compounding might be considered when dose, compliance, or availability is an issue. However, solubility, stability, absorption, and potency are potential concerns. Specifically, drugs that are packaged in blister packs or moisture-proof barriers may not be amenable to compounding into liquid formulations, while drugs originally packaged in light-proof containers may be inactivated if exposed to light. In addition, transdermal medications can be an easy and convenient way to administer medications, particularly in cats. However, in one study the bioavailability of fluoxetine was approximately 10% of oral dosing, and in another study systemic absorption of amitriptyline and buspirone was found to be negligible (compared to oral dosing).8,9 Thus, transdermal medications are not currently considered an effective method to administer psychoactive drugs in dogs and cats.
Target conditions for drug therapy
Most psychotropic drugs used in behavioral medicine exert their effects on the so-called diffuse modulatory neurotransmitters, including serotonin, dopamine, norepinephrine, and acetylcholine (Table 8.1).
Table 8.1 Drugs that affect neurotransmitters
Neurotransmitter | Drugs that increase effects | Drugs that decrease effects |
---|---|---|
Acetylcholine | Carbachol, bethanechol, cholinesterase inhibitors (donepezil, galantamine, neostigmine, physostigmine) | Atropine, scopolamine curare (blocks N-m nicotinic synapses) |
Dopamine | l-DOPA, amphetamine, methylphenidate, apomorphine, bromocriptine, selegiline (MAO-B inhibitor), MAO inhibitors | Neuroleptics (e.g., acepromazine, risperidone) |
GABA | Benzodiazepines | Flumazenil |
Norepinephrine | Alpha-adrenergics (ephedrine, phenylpropanolamine), amphetamine, MAO inhibitors | Beta-blockers (pindolol, propranolol), alpha-agonist (clonidine), risperidone |
Serotonin | Tryptophan, selective serotonin reuptake inhibitors, sumatriptan, MAO inhibitors, buspirone | Cyproheptadine, risperidone |
GABA, γ-aminobutyric acid; MAO, monoamine oxidase.
Overall framework of drug therapy
Adjunct to behavior therapy
Psychotropic drugs are most commonly used as an adjunct to behavior therapy. Factors to consider include whether there may be a drug that could help to resolve the problem more quickly, potential adverse effects of the medication, the owner’s commitment to the behavioral program, and the humane considerations for the pet (i.e., whether the pet’s interests might be better served with medication). The treatment of separation anxiety, fears, and phobias, and aggression are examples of conditions in which a drug might help facilitate the initiation and implementation of behavior therapy. For example, studies on the use of clomipramine for separation anxiety showed that there was greater and faster improvement in the group with drugs and behavior modification compared with the group with behavior modification alone (i.e., placebo group).10 Choosing an appropriate drug may provide an opportunity to resolve the problem in a quicker or safer manner. However, without concurrent behavioral modification, the problem may not improve significantly and is likely to recur when the drug is removed.
Drug desensitization
Drug desensitization is a technique that can be applied when the stimulus cannot be effectively controlled or reduced, or when there are multiple stimuli that lead to fear or aggression. The drug should be given at sufficient dosage so that the pet is relaxed and calm when exposed to the stimulus. By pairing exposure to the stimulus with favored treats, the association can be made even more positive (counterconditioning). Even if the drug alone does not completely calm the pet, it may still be possible to reduce its arousal enough to get the pet to respond to a training command such as focus, sit/stay, or settle, and this response can then be reinforced (response substitution). Drug-aided desensitization should be combined with other behavioral modification techniques such as systematic desensitization, controlled flooding, counterconditioning, and differential reinforcement (see Chapter 7) so that behavioral techniques, rather than the drug itself, are the principal methods of altering behavior. As soon as the successful response (and mood) can be consistently achieved in the presence of the stimulus, the drug can be gradually reduced at subsequent training sessions.
Underlying pathology present
A final indication for drug use is when underlying pathology, whether medical or behavioral, is present. Medical problems that may be involved in the expression of behavior problems include endocrinopathies (hyperthyroidism, hypothyroidism, and hyperadrenocorticism), epilepsy, hepatic encephalopathy, interstitial cystitis, cognitive dysfunction syndrome, chronic painful conditions, or neuropathic pain (see Chapter 6). Similarly, behavioral pathology (i.e., where behavioral changes may be due to altered neurotransmitter function) may only improve with appropriate drug therapy. Compulsive disorders, attention deficit hyperactivity disorders (ADHDs), generalized anxiety disorders, and some of the Pageat (French) classifications of behavior disorders (such as hypersensitivity-hyperactivity and dissociative disorders) may require medication to treat the problems effectively (i.e. mental health disorders).
Classification and selection of psychotropic drugs
Labeling a drug for its therapeutic value might seem clear and self-explanatory but it could create some confusion for both the clinician and the patient. Many conditions in human beings, like anxiety or panic, have a clear counterpart in veterinary medicine. However, others, like depression or schizophrenia, don’t. Since most psychotropic drugs used in behavioral medicine are only licensed for humans, clinicians must emphasize to their clients that information contained on the package insert (or in books, brochures, or websites) refers to use in humans and does not necessarily apply to animals. For example, paroxetine is often classified as an antidepressant, but its use in veterinary behavioral medicine cannot be understood in terms of that diagnostic category. Furthermore, it is becoming more and more clear in veterinary behavioral medicine and human psychiatry that very few, if any, psychotropic drugs can be considered diagnosis-specific. Thus, the current trend in human psychiatry is to abandon the terminology based on indications in favor of one referring to the primary effects on neurotransmission or to the chemical structure.11
Neurotransmitters
After detaching from receptor sites, the neurotransmitter molecules are either enzymatically degraded or diffuse to reuptake receptor sites on the presynaptic neuron, where they attach and are transferred into the cell. The reuptake pump decreases the neurotransmitter’s interneural concentration by physically removing molecules from the interneural space, but also indirectly by increasing the intracellular storage pool. This occurs because an intracellular feedback system inhibits neurotransmitter synthesis as the concentration of neurotransmitter increases within the neuron. Thus, the reuptake receptor site provides an excellent target for drug action by effectively increasing the amount of neurotransmitter available to interact with the postsynaptic cell. Many psychotropic drugs exert their action by either blocking the degrading enzyme or blocking the reuptake pump (Figure 8.1).
It is important to realize that the mechanisms of action of most psychotropic drugs have not been fully elucidated. This could be an additional factor to explain the different clinical responses to drugs apparently belonging to the same category.10
Monoamines
Serotonin
Chemistry and pharmacology
Serotonin levels are controlled by cellular uptake of tryptophan and the action of tryptophan hydroxylase, which is involved in the rate-limiting step in serotonin synthesis. Tryptophan is discussed in detail in Chapter 9. Inactivation is by reuptake or by breakdown by MAO. An increase in serotonin may be associated with an activation of the pituitary–adrenal axis. A decrease in serotonin may lead to depression, increased anxiety, aggression, and decreased food intake. In humans, altered serotonergic system function is associated with hyperaggressive states, schizophrenia, affective illness, major depressive illness, and suicidal behavior. Increasing or normalizing serotonin levels may be useful in the treatment of depression in people, compulsive and stereotypic disorders, and some forms of aggression and anxiety. Selective serotonin reuptake inhibitors (SSRIs) and tricyclic antidepressants (TCAs) increase serotonin availability by decreasing reuptake, and MAO inhibitors (MAOIs) increase serotonin by decreasing serotonin breakdown. Serotonin agonists have been shown in rat studies to reduce offensive aggression, without blocking defensive aggression. Impulsivity (disinhibition, unpredictability, prolonged arousal) may be correlated with the presence of low serotonin metabolites in the cerebrospinal fluid (5HIAA).12 This is also true in humans regarding impulsive violent offenders. Serotonin reuptake inhibitors may decrease these forms of aggression while cyproheptadine (serotonin agonist) may increase aggression. In studies of nonhuman primates increased serotonin was correlated with higher social rank but inversely correlated with aggression.13 In primate studies, fluoxetine inhibited impulsivity in a resident model.14 Mice lacking the 5HT1B receptor were more aggressive, while mice lacking 5HT1 receptors were more anxious.15,16 Serotonin may act to inhibit aggression, at least in part, by antagonizing the aggression-promoting effects of vasopressin. It has also been suggested that there is a relationship between dopamine and serotonin levels in that higher levels of 5HT may inhibit dopamine release. In fact, one factor in schizophrenia in humans may be decreased inhibition of the release of dopamine by 5HT in the mesencephalon and frontal cortex, and that treatment should help to normalize the relationship between 5HT and dopamine. Recent canine studies have demonstrated differences within breed in 5HT levels between aggressive and nonaggressive dogs.17–19
Amino acids
Amino acids are the most prevalent of the CNS neurotransmitters. In contrast with the diffuse modulatory systems, they are involved in rapid point-to-point communication. Glycine, glutamate, and aspartate are three of the most important of the 20 amino acids that function as neurotransmitters. Glycine is an inhibitory neurotransmitter of the hindbrain and spinal cord. Glutamate is an excitatory amino acid, which may be produced in abnormal levels in aggression and impulse control disorders. N-Methyl-d-aspartic acid (NMDA) antagonists such as memantine suppress glutamate, as do barbiturates and progestins. Tryptophan, a precursor to serotonin, has been used as a food supplement as a means of enhancing serotonin transmission. Details can be found in Chapters 9 and 11.
Neuropeptides
Endorphins and neurokinins (substance P and NK1)
This group is composed of molecules that are short-chained amino acids. They mainly function as modulators of other neurotransmitters, evoking facilitation or inhibition of neurotransmitter activity at the postneuron receptor site. The opioid system plays an important role in mediating both physical pain and social affective disorders.20 Endogenous brain opioids may be involved in the development and maintenance of social behavior and social attachments. Opioids have been shown to reduce crying and motor agitation in puppies during social isolation. Opioid administration in socially deprived kennel dogs has also been shown to ameliorate chronic emotional distress while opioid blockade (i.e., administration of naloxone) appears to intensify emotionality. On the other hand, since morphine reduces social need, it appears to decrease social solicitation, while naloxone increases solicitive behavior, including tail wagging and face licking.21 Therefore opioids may play a role in the development and treatment of fear and anxiety. CNS endorphin release has been implicated in some compulsive disorders involving stereotypic behavior, although the role is still not well understood.
Substance P is a neuropeptide that is found in the spinal cord and CNS; it is a modulator of nociception involved in signaling the intensity of noxious stimuli. Along with neurokinin 1 (NK1), substance P is likely involved in the body’s response to stress, anxiety, invasion of territory, and noxious or aversive stimuli. Substance P is present in the limbic system, including the hypothalamus and amygdala, and may play a role in emotional behavior. NK1 is present in the hypothalamus, pituitary, and amygdala, which play a role in affective behavior and response to stress. In cats, NK1 substance P receptors in the midbrain periaqueductal gray potentiate defensive rage and suppress predatory aggression.22 Substance P is one of the primary neurotransmitters released during tissue and mast cell damage.23 Blocking substance P might reduce inflammation, pain, nausea, and neuropathic pain.24 Therefore, as a substance P inhibitor (NK1 antagonist), maropitant citrate (Cerenia: Pfizer Animal Health) may reduce neurogenic inflammation, and substance P inhibitors have potential applications for inflammatory conditions in humans.25 Although there have been no published studies to date on its efficacy as an anti-inflammatory agent in pets, it has been reported to be used anecdotally on an extra-label basis for its anti-inflammatory effects at 1–2 mg/kg twice weekly, every other day or 5 days on and 2 days off (Mandelker, L., personal communication, 2010). It should not be used for long-term daily use as depletion of substance P could lead to depletion in dopamine and resultant parkinsonian-like tremors, although in a study of 15 days of daily injections of maropitant citrate in cats at up to 5 mg/kg for 15 days, it was well tolerated with no adverse effects, except for one cat that developed mild tremors during sleep (but no signs when awake).26
For nausea or vomiting, a dose of 2 mg/kg can be used daily PO for up to 5 days; the oral product is not licensed for cats, but has been used at 0.5–1 mg/kg daily PO for 5 days (off label).26 The product is also available in injectable form, and the dosage for acute vomiting is 1 mg/kg SQ once daily, for up to 5 days in dogs 8 weeks of age and older, and in cats 16 weeks of age and older (label indications may vary by country).26 For motion sickness the canine dose is 8 mg/kg daily for 2 days.
Other neurotransmitters
Hormones
Endogenous compounds such as hormones or other substances that are naturally produced by the body have generated renewed interest for the treatment of human psychiatric disorders. The idea is that if we administer substances that the body already has, we can rebalance underlying deficits. Recent studies have shown that estrogen supplementation, growth hormone, and even secretin, which has been trialed in the treatment of autism, may have beneficial effects in depressed patients. Although some may consider that using substances already found in the body is somehow safer, hormone excess can also lead to serious medical problems. Melatonin is covered in detail in Chapter 9.
Main classes of psychotropic drugs
Neuroleptics/antipsychotics
On the other hand, acepromazine might be particularly beneficial in the sedation of dogs that are too fearful or aggressive to handle or restrain safely and effectively. Oral combinations with benzodiazepines might be effective prior to veterinary visits, grooming, or other potentially fear-evoking events.27 However, intramuscular combinations of either acepromazine or dexmedetomidine plus a narcotic and midazolam are generally highly effective for most procedures (see Chapter 23).
Risperidone has been used in some European countries to treat recently developed sociopathies toward people. Neuroleptics have been utilized in stage 3 deprivation syndrome (deprivation depression associated with sleep disturbances and self-injurious behaviors), in separation anxiety when there are motor disturbances (pipamperone in combination with clomipramine), in some cases of primary dissocialization, in social phobias (thioridazine or fluphenazine), and in stage 2 sociopathies toward humans (in combination with cyproterone and carbamazepine) (see Chapter 22).28
Benzodiazepines
Glutamate and GABA are, in fact, a system in balance where GABA controls glutamate, and vice versa. In general benzodiazepines cause decreased anxiety, hyperphagia, muscle relaxation, decreased locomotor activity, and varying degrees of sedation. Benzodiazepines act as mild sedatives at low doses, as antianxiety agents at moderate doses, and as hypnotics at high doses. They may also act as anticonvulsants. Studies of animal models of anxiety have shown that the inhibition related to fear and anxiety can lead to a decrease in eating, drinking, and exploratory behaviors, and an increase in avoidance or aggression, while treatment with benzodiazepines leads to disinhibition, resulting in increased exploration, resumption of appetite, and a decrease in avoidance and aggression.29 However, in some instances, such as where the fear response is one of avoidance, disinhibition could lead to an increase in aggression. In humans and laboratory animals the aggression-heightening effect of benzodiazepines seems to be dose-dependent, with lower doses enhancing aggression. Some evidence from studies on laboratory rodents indicate that certain benzodiazepines such as oxazepam and clorazepate might be safer in terms of their potential aggression-heightening effect.30
In one study examining the effects of diazepam in dogs with behavior problems, it was very effective or somewhat effective in 67% of anxiety-related behavior cases.31 Upon discontinuation of diazepam, owners reported either lack of efficacy or adverse effects, ranging from sedation to increased appetite, ataxia, agitation, activity, and aggression. Owners reported greater success when using diazepam for fear of thunderstorms than for separation anxiety. When doses of 0.8 mg/kg were used, there were greater reports of increased activity.31 Therefore when side-effects are seen, dose adjustments should be considered or alternative benzodiazepines selected.
Benzodiazepines might be used on an as-needed basis for the treatment of situational anxiety such as with thunderstorms, fireworks, car rides, visits to veterinary clinics, or the anxiety associated with departures in dogs with separation anxiety. They reach peak effect shortly after each dose and can be used alone or in combination with other drugs on an as-needed basis.32,33
Benzodiazepines are absorbed unchanged from the gastrointestinal tract, with the exception of clorazepate, which is converted to its intermediate metabolite nordiazepam (desmethyldiazepam) in the gastrointestinal tract prior to its absorption. Most benzodiazepines, such as diazepam, are metabolized by the liver and some have active intermediate metabolites that may be more active than the parent compounds. The metabolites are then conjugated by the liver and excreted in the urine. For instance, nordiazepam, which in turn is converted to the active metabolite oxazepam, is an active metabolite of chordiazepoxide, diazepam, and clorazepate. Alprazolam and triazolam have short-lived metabolites with minimal activity. Diazepam and particularly its active metabolites have been reported to cause rare cases of hepatotoxicity in cats and for this reason is no longer considered the benzodiazepine of choice in that species.34 Anorexia can be a sign that the cat is having a hepatic reaction and should be cause for immediate cessation of the drug. Oxazepam and lorazepam have no intermediate metabolites and therefore may be safer for the obese, elderly, or those with liver disease and have less chance of residual or cumulative effects.
In cats, diazepam has been used successfully for spraying, anxiety-motivated inappropriate elimination, anxieties, and fears (including fear aggression). It has also been used successfully to stimulate appetite, to control seizures, and to treat feline hyperesthesia. Diazepam may also decrease predation through its inhibitory effect on acetylcholine. Because of the relatively short half-life in dogs (3.2 hours compared with 5.5 hours in cats), as well as the short half-life of its active metabolite nordiazepam (3.6 hours in dogs compared with 21 hours in cats), its primary use in dogs is alone or in combination with SSRIs or TCAs as adjunct to desensitization programs for fears and fear aggression, and prior to fear- and anxiety-evoking events such as owner departures, thunderstorms, or fireworks.35 However, with continued oral dosing of 1–2 mg/kg TID, steady-state plasma concentrations might be achieved.36
Lorazepam provides more sustained release in people but has a slower onset of action. In dogs it has been reported to maintain therapeutic blood levels for 60–90 minutes at a dose of 0.2 mg/kg.35 It has been used for the control of acute agitation and aggression in people and may be effective for an overly fearful victim cat in cases of intercat aggression. Intranasal administration also maintained plasma levels consistent with anticonvulsant activity in 3/6 dogs for at least 60 minutes.37
Long-term use of benzodiazepines may lead to dependence. In humans, benzodiazepines also pose a risk of dependency and abuse; therefore, while drug use by pets can be responsibly controlled by the pet owner, veterinarians should exercise some caution in dispensing and monitoring of benzodiazepine use if they suspect that there might be a potential for human abuse. All benzodiazepines, particularly those of high potency, should be withdrawn slowly (e.g., 10–25% per week). This is especially true in patients administered benzodiazepines for the control of seizures, as status epilepticus may be precipitated if the drug is not tapered slowly. Behavior problems may recur when the drug is withdrawn. In one study, 91% of cases of inappropriate urination in cats recurred when the diazepam was discontinued.38
Combination therapy of benzodiazepines plus TCAs, SSRIs, propranolol, or phenothiazines has also been used occasionally in veterinary medicine (e.g., separation anxiety, thunderstorm phobias). In an open trial, alprazolam in conjunction with clomipramine and behavior modification seems to be effective in controlling noise phobia in dogs.32 For immediate control of panic or phobic states, intranasal lorazepam or intranasal or intrarectal administration of diazepam may be an option for some owners where oral dosing is impractical and immediate effects are needed.37,39
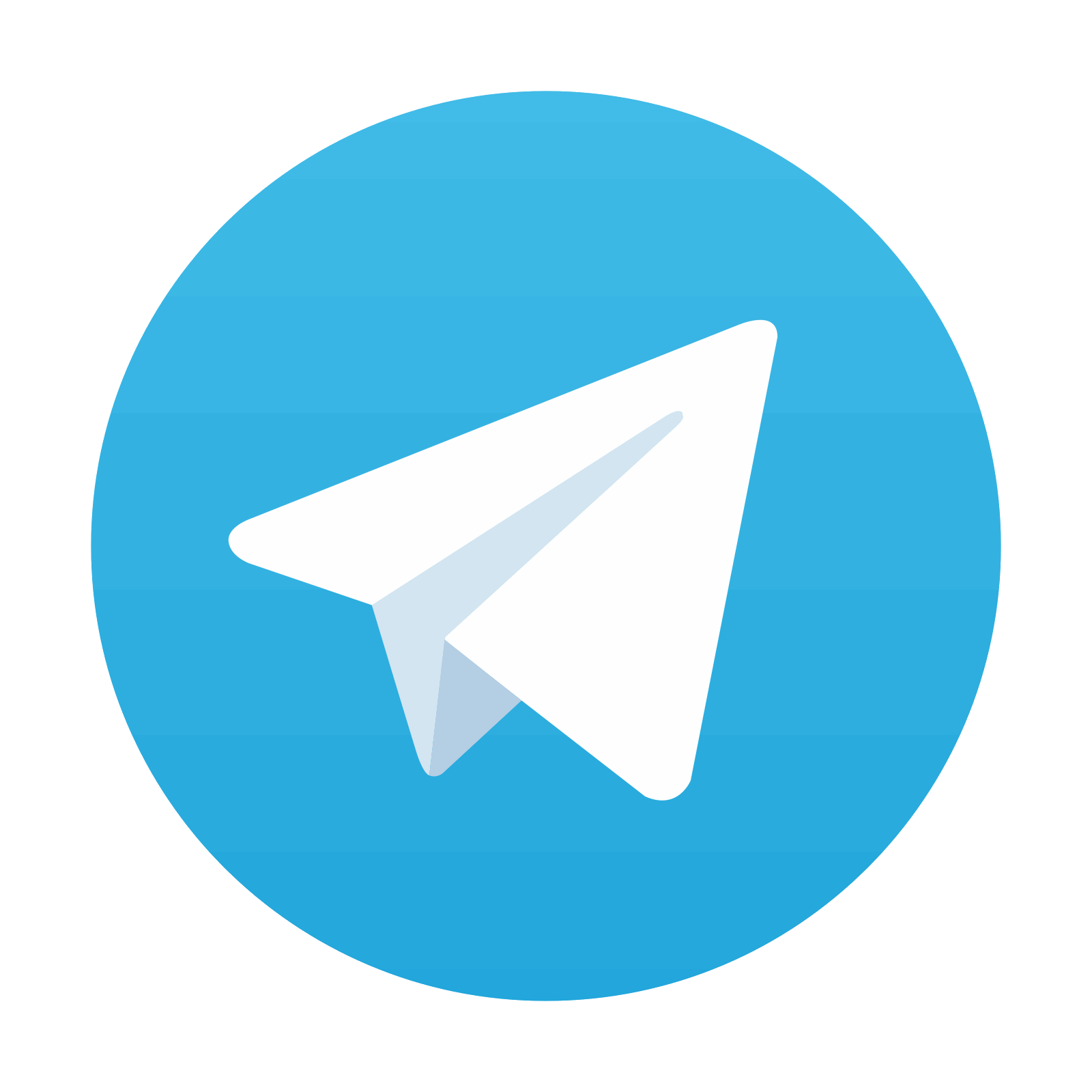
Stay updated, free articles. Join our Telegram channel

Full access? Get Clinical Tree
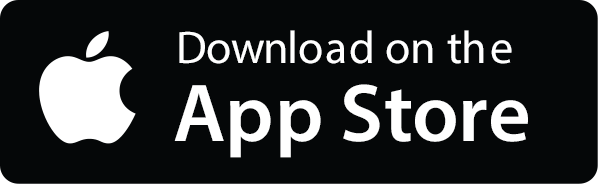
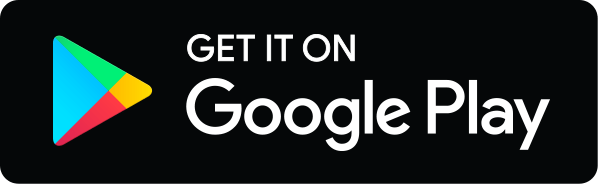