Chapter 16 BIOCHEMISTRY OF EXERCISE AND FATIGUE HEMATOLOGY OF HORSES IN TRAINING Exercise tests and the hemogram Limitations of treadmill tests Measurement of maximal oxygen consumption Poor skeletal conditioning as a cause of “wastage” Methods for assessing bone quality Shin soreness—an exercise-induced injury Training to maximize bone quality and minimize lameness SORE BACK IN THE PERFORMANCE HORSE There are three principal aims of the application of science to training horses. 1. To develop techniques for testing fitness of horses for competition. Exercise testing may contribute to the financial success of training establishments in several ways. These include: (a) Early identification of horses with inferior stamina. This information can hasten their removal from the stables, or identify limitations to performance in young horses even before they have commenced racing. (b) Repeated tests evaluate improvement or deterioration in fitness during training. Information gained in one preparation can be used to assess the fitness attained in subsequent preparations. 2. To design training programs that maximize fitness, reduce the incidence of injury and improve results in competition. Heart rate measurements during exercise and blood lactate concentrations after exercise can provide a guide to ideal training speed for development of stamina. This enables adjustments to the training routine in order to increase fitness without overtraining the horse. Many of the techniques referred to in this chapter have been validated both in the laboratory and on the racetrack in Europe, USA and Australia. Moreover, similar techniques have been used by human athletes and sports science laboratories for decades and are now part of the daily routine of human athletic training. It has taken time to adapt and develop suitable protocols for horses. It is hoped that this chapter increases veterinarians’ understanding of the normal physiology and biochemistry of exercise in equine athletes and illustrates the limitations of some of the traditional methods of assessment of equine performance. This chapter also points the way ahead to the application of new techniques for monitoring fitness in horses. When the demands for energy are low, aerobic metabolism is capable of meeting the requirements for ATP resynthesis. Aerobic metabolism is the primary pathway by which ATP is regenerated during endurance-type exercise. Aerobic metabolism also contributes greatly to the energy supply during high intensity exercise such as galloping. Anaerobic metabolism makes up the deficit in total energy resynthesis during high intensity exercise. At the onset of exercise, the delivery of oxygen to the muscles does not instantaneously reach the level required to support aerobic metabolism. Approximately 30s of exercise is required before maximal aerobic activity is achieved. During this time, there is an increase in heart rate and ventilation, an increase in the oxygen carrying capacity of the blood as the splenic erythrocyte reserve is mobilized, and redirection of blood flow to the skeletal muscles. Increases in body temperature may enhance enzyme activity. During this period, the deficit in energy production is met by anaerobic metabolism. During intense exercise, the maximal accumulated oxygen deficit can be measured, and this measure reflects the anaerobic capacity. During endurance exercise, the biochemical cause of fatigue is depletion of glycogen reserves in the muscle. Muscle biopsy studies have shown that muscle fibers are recruited progressively as glycogen stores in other fibers are exhausted and their power output decreases. When all fibers have depleted their glycogen reserves, exercise must stop. The oxidation of fatty acids alone is not sufficient to maintain the required power output, although the energy held in fat stores would support many days of continuous slow exercise. However, increased use of fatty acids as a substrate for aerobic metabolism has the effect of sparing glycogen reserves and increasing the potential duration of exercise. Aerobic training enhances fat utilization by increasing the concentrations of enzymes involved in fat metabolism and increasing mitochondrial volume. The development of fatigue during high intensity exercise is related to oxygen delivery to the muscles. Oxygen modulates intracellular metabolism to influence the rate of change of metabolites—mainly inorganic phosphate (Pi) —and may affect fatigue by influencing the rate of Pi accumulation. In muscle cells, an increase in Pi may reduce crossbridge force production and myofibrillar Ca2 sensitivity, and inhibit Ca2 release and/or reuptake. When oxygen delivery is limited, there is greater reliance on substrate-level phosphorylation for ATP resynthesis, resulting in disruption of metabolic homeostasis and a decline in developed tension. Studies in humans have found that hypoxic conditions that decreased aerobic capacity had no effect on performance for sprints of up to 60s, and had very little effect on sprints of up to 120s, indicating that maximal human running speeds for short- and intermediate-length sprints are relatively unaffected by large reductions in aerobic power. The ability to maintain power output at a time when aerobic capacity was decreased suggests that additional metabolic energy must have been derived from anaerobic sources during exercise in hypoxic conditions, that maximal metabolic power outputs during sprinting under normoxic conditions are not limited by rates of anaerobic metabolism, and that human running speed is largely independent of aerobic power during all-out sprints lasting less than one minute. 1. The cardiovascular/aerobic model of fatigue is based on the idea that increasing the ability of the muscles to utilize oxygen, thus delaying the onset of lactate accumulation in the blood, will delay the onset of fatigue. 2. The energy supply/energy depletion model is based on the idea that fatigue during high intensity exercise may be due to the inability to supply ATP at a sufficient rate to support exercise. 3. The muscle recruitment (central fatigue)/muscle power model holds that brain concentrations of serotonin (and perhaps dopamine, acetylcholine and other neurotransmitters), alter the neural drive to exercising muscles, or that reflexes generated in the exercising muscles reduce recruitment of alpha motor neurons at the level of the spinal cord. 4. The biomechanical model states that biomechanical characteristics of the muscle (such as the capacity to act as a spring), affect the demands for force production, and therefore limit metabolite accumulation and heat production. 5. According to the psychological model, the ability to sustain exercise results from a conscious effort. Other factors that limit performance, in particular skills and psychology, are beyond the scope of this chapter. Skills training may result in improvements in gait that result in increased efficiency of movement and a decrease in energy demands. In addition, training of this type may improve the psychological condition of a horse, making it more willing to work. The benefits of physical training can easily be undone by inadequate provision of rest periods before competition, so that the horse becomes tired and disinterested in competing. Aerobic training results in increases in circulating blood volume and stroke volume, which produce an increase in the amount of oxygen transported to the muscle. Within the muscle, there is an increase in the capillary distribution to individual muscle fibers, and fiber cross-sectional area. Mitochondrial volume, enzyme concentrations and myoglobin concentration increase within the cell. Training also appears to increase intracellular glycogen stores. An appropriate training load to stress the anaerobic system is one that results in post-exercise blood lactate concentrations in the range 4–10 mmol/L. Heart rates in the range of 85–95% of maximal are also appropriate. At this exercise intensity, anaerobic metabolism is making a significant contribution to energy generation. The resulting metabolic changes cause an increase in sprint capacity and an increase in intramuscular buffering capacity, which enables the horse to sustain intense exercise for longer periods. During the sprints, the horse should not reach the point of fatigue. A fatigued horse is unable to work at maximum speed, and therefore does not receive the appropriate training stimulus. In addition, the risk of injury is increased in a fatigued horse because gait becomes abnormal. The amount of sprint training required to achieve the maximum performance benefit in the horse is unknown. The distance over which the horse is sprinted should equal, or slightly exceed, the distance over which it will sprint during competition. Post-competition blood lactate concentrations in showjumpers approach 10 mmol/L, indicating that anaerobic metabolism makes a significant contribution to energy generation. Training sessions are generally limited to 20–30 min of jumping or other work. There are five factors to be considered in designing an interval training program. These are: 1. The intensity and duration of each interval 3. The rest interval between bouts 4. The type of activity during the rest period Overtraining can be defined as an imbalance between training and rec-overy. Overtrained animals appear fatigued and stale. Their performance deteriorates and they may lose weight. Short-term overtraining can be corrected by rest for a period of days to weeks. Where the severity of the overtraining is greater, a recovery period of several months is required. The horse holds a large store of erythrocytes in its spleen. Under stimulation of the sympathetic nervous system, the spleen contracts, injecting the stored cells into the circulation and resulting in an increase in the hematocrit. Splenic contraction occurs in anticipation of or during exercise. However, changes in the hematocrit (q.v.) are minimal following endurance exercise unless dehydration has occurred. Hematocrit values >0.55L/L, and plasma protein concentrations >90g/L in endurance horses are signs of potentially dangerous fluid loss. It has long been argued that polycythemia (q.v.) is the mechanism or hallmark of overtraining syndrome in Swedish Standardbred trotters. However, a longitudinal study of overtraining that included a control group failed to confirm that overtraining causes red cell hypervolemia.
Performance-related problems and exercise physiology
INTRODUCTION
BIOCHEMISTRY OF EXERCISE AND FATIGUE
AEROBIC METABOLISM
ANAEROBIC METABOLISM
FATIGUE
PRINCIPLES OF TRAINING
INTRODUCTION
AEROBIC TRAINING
ANAEROBIC TRAINING
TRAINING FOR SPECIFIC PURPOSES
Showjumpers
INTERVAL TRAINING
OVERTRAINING
HEMATOLOGY OF HORSES IN TRAINING
RED BLOOD CELLS
Stay updated, free articles. Join our Telegram channel

Full access? Get Clinical Tree
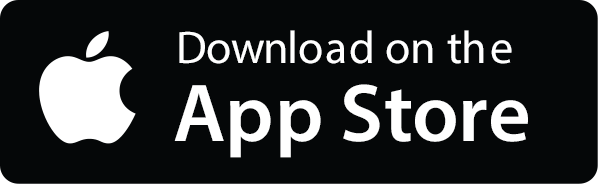
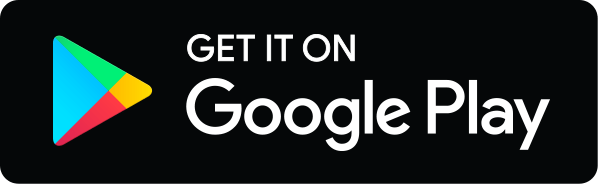