Sandra Z. Perkowski1 and Mark A. Oyama2 1 BluePearl Specialty and Emergency Pet Hospital, Malvern, Pennsylvania, USA 2 Department of Clinical Sciences and Advanced Medicine, School of Veterinary Medicine, University of Pennsylvania, Philadelphia, Pennsylvania, USA In animals with suspected cardiac disease, care must be taken both prior to and during anesthesia to assess the heart’s ability to (1) provide adequate cardiac output and tissue perfusion, (2) maintain low venous pressures and prevent congestion, and (3) avoid arrhythmias. The preanesthetic database should include the medical history and physical examination, with particular attention to cardiac and pulmonary auscultation, inspection of the jugular veins, and palpation of peripheral arterial pulses. Commonly considered diagnostics include electrocardiography (ECG), thoracic radiography, echocardiography, and noninvasive blood pressure measurement. The use of cardiac‐specific blood‐based markers such as N‐terminal pro‐B‐type natriuretic peptide (NTproBNP) and cardiac troponin I (cTnI) as part of the preanesthetic work‐up in animals with suspected cardiac disease may be included. The decision regarding if and how to anesthetize patients with cardiac disease relies on the appropriate selection and interpretation of these and other diagnostic tests. ECG is the gold standard for the evaluation of cardiac arrhythmias. However, in the absence of arrhythmias, the sensitivity of ECG to detect underlying cardiac dysfunction and disease is relatively poor. The ECG should be evaluated for heart rate, mean electrical axis (when applicable), rhythm, and criteria for cardiac chamber enlargement. The reader is referred to several excellent reviews of ECG interpretation for further information [1–3]. ECG findings that most commonly impact anesthesia include abnormalities of rate (either bradycardia or tachycardia) or criteria for chamber enlargement. In cats, abnormalities of mean electrical axis are occasionally detected with underlying structural disease, such as cardiomyopathy. Clinically important bradycardias include second‐ or third‐degree atrioventricular nodal (AV) block, sinus arrest or block, sinus brady‐cardia, and, less commonly, atrial standstill. The presence of AV block, sinus arrest/block, and sinus bradycardia is often challenged by administration of vagolytic agents such as atropine or glycopyrrolate. Complete resolution of the bradycardia following challenge usually indicates that the bradycardia is due to physiologic high resting vagal tone, and bradycardia occurring while under anesthesia can often be effectively treated with repeated administration of atropine or glycopyrrolate. Incomplete resolution or absence of response to vagolytic challenge often indicates injury or disease of the cardiac conduction system and additional diagnostics, such as thoracic radiography, echocardiography, serial ECG, or 24‐h ambulatory ECG (Holter) monitoring should be pursued prior to anesthesia. Depending on the response to vagolytic challenge, some dogs with partial responses can be safely anesthetized with proper selection of anesthetic agents, monitoring, and/or availability of temporary artificial cardiac pacing. Most instances of sinus block/arrest or sinus bradycardia that are part of a pronounced respiratory sinus arrhythmia are vagally mediated and present little risk for anesthesia. In contrast, most cases of high‐grade second‐degree AV block, third‐degree AV block, and atrial standstill indicate the presence of either primary cardiac disease or severe electrolyte or acid‐base disturbances and are associated with a significant increase in anesthetic risk. Various forms of bradycardia can also be present in patients with sick sinus syndrome (SSS) wherein intermittent periods of bradycardia and tachycardia both occur. Clinically important tachycardias include supraventricular tachycardia (SVT), ventricular tachycardia (VT), and atrial fibrillation (AF) or flutter. Sinus tachycardia due to pain, hyperthyroidism, congestive heart failure, and drugs/toxins (e.g., theophylline, terbutaline, and theobromine) can also be present. The impact of tachyarrhythmias on anesthesia depends on the rate, frequency, and duration of such arrhythmias. Very rapid, frequent, and sustained tachyarrhythmias decrease diastolic filling time and cardiac output (CO) while increasing myocardial oxygen demand. Tachyarrhythmias such as sustained SVT, VT, and AF are often associated with clinically significant primary cardiac disease, such as degenerative mitral valve disease in the dog or various forms of cardiomyopathy in both the dog and cat. Ventricular arrhythmias are also common in dogs with extra‐cardiac disease, especially diseases of the abdomen such as splenic or hepatic neoplasia or gastric dilation‐volvulus. Thus, further diagnostics such as thoracic radiography, echocardiography, Holter monitoring, abdominal ultrasound, and serum chemistry are usually pursued in animals with tachyarrhythmias. In cases wherein arrhythmias appear to be due solely to extra‐cardiac causes such that echocardiography reveals normal cardiac structure and function, anesthesia often can be achieved with minimal additional risk. Thoracic radiography is an extremely useful tool in assessing patients with cardiac disease. Cardiac disease results in activation of neurohormonal responses that serve to increase cardiac preload via renal retention of fluid and sodium. The resultant volume overload produces eccentric cardiac hypertrophy, the severity of which reflects the degree of injury and neurohormonal activity. Thus, radiographic heart size is an excellent surrogate marker of disease severity and risk for congestive heart failure (CHF). Evaluation of radiographic heart size is confounded by variables such as radiographic technique, patient body weight, chest conformation, and breed. Standardized measurement techniques such as the vertebral heart size (VHS) are useful to minimize variability and increase diagnostic value. The VHS system has been extensively reviewed elsewhere [4–6]. Briefly, the long and short axes of the heart are measured from the right or left lateral projection and these measurements are indexed to the number of vertebral bodies starting from the cranial aspect of the fourth thoracic vertebra (Fig. 36.1). The sum of the vertebral length and width of the heart represents the VHS. The range of VHS in healthy dogs and cats is 8.5–10.5 and 6.9–8.1 vertebra, respectively. Although these ranges are generally regarded as applicable across a wide range of breeds and ages, some reports indicate slightly different ranges, although only two of the studies had at least 100 subjects (Table 36.1). As a preanesthetic test, VHS is useful in determining the severity of canine degenerative mitral valve disease in geriatric patients with the typical left‐sided systolic murmur. In the authors’ experience, regardless of the intensity of the murmur, dogs with VHS < 11.0–11.5 are at low risk for spontaneous development of CHF, whereas those with VHS > 11.5–12.0 are at higher risk. Coupled with inspection of the pulmonary veins and pulmonary parenchymal pattern, VHS helps assess the risk of induced CHF secondary to IV fluid administration (at 5–10 mL/kg/h) while under anesthesia. In species and diseases other than canine degenerative mitral valve disease, the authors regard VHS as a useful means to assess the risk of induced or spontaneous CHF in dogs with dilated cardiomyopathy and cats with hypertrophic or restrictive cardiomyopathy. In instances of suspected canine or feline cardiomyopathy, detailed echocardiographic evaluation of systolic and diastolic function can supplement radiographic findings. Figure 36.1 Right lateral thoracic radiograph from a 13‐year‐old female spayed Toy Poodle demonstrating the vertebral heart size (VHS) measurement technique. The length of the cardiac silhouette is measured from a point ventral to the carina of the trachea to the apex of the heart and the width of the silhouette is measured along the widest portion of the heart perpendicular to the long axis. The ventral border of the caudal vena cava is often used as the starting point for the width measurement. The length and width measurements are compared with the number of vertebral bodies starting from the cranial aspect of the fourth vertebral body. In this instance, the length of the silhouette is 6.2 vertebra and the width is 5.0 vertebra, yielding an overall VHS of 11.2. Table 36.1 Reported mean (SD) radiographic vertebral heart size from various studies in dogs and cats. Echocardiography represents the gold standard for the clinical evaluation of cardiac structure and function. It is less useful than thoracic radiography in trying to determine the presence or likelihood of CHF manifested as pulmonary edema. Echo provides data regarding ventricular and atrial chamber dimensions, thickness of the ventricular walls and interventricular septum, pattern of blood flow through the heart and proximal portions of the great vessels, myocardial contractility, and morphology of valve structure (Table 36.2). Echo should be performed by individuals not only proficient in image acquisition but also with an adequate familiarity with the pathophysiology of the most common cardiac diseases since interpretation of the echocardiographic‐derived data is necessary to assess the impact of changes on anesthetic risk. Measurements from individual animals are interpreted using reference ranges from healthy animals indexed to body weight. The most accurate reference ranges utilize indices indexed to the body weight raised to the power of approximately 1/3, providing a single range of values applicable across all body weights (Table 36.3) [14]. Diseases that result in volume overload (e.g., eccentric hypertrophy) such as canine mitral valve disease, dilated cardiomyopathy (DCM), and patent ductus arteriosus (PDA), result in increased diastolic chamber dimensions, whereas diseases that result in concentric hypertrophy such as feline hypertrophic cardiomyopathy (HCM), systemic hypertension, and congenital subaortic stenosis (SAS), result in increased diastolic and systolic ventricular wall thickness. Diseases that produce decreased contractility, such as DCM, result in increased systolic chamber dimensions. Interpretation of the echocardiogram relies on both subjective and objective evaluation. Because of many breed peculiarities with respect to chest conformation and imaging planes, objective data should always be compared against the subjective evaluation of the sonographer. In fact, subjective estimation of cardiac function by experienced echocardiographers possesses a better correlation with angiographic evaluation than many of the routine objective calculations [15]. Thus, objective measurements should align closely with the trained echocardiographer’s subjective assessment of cardiac morphology and function, and discrepancies between the two often indicate measurement errors due to suboptimal imaging planes. Table 36.2 Common echocardiographic measurements and calculations. Table 36.3 Upper and lower bounds of the 95th percentile confidence interval (CI) for indexed echocardiographic formula constants in healthy dogs. The constants multiplied by the body weight (BW in kg) raised to an exponential power provide a single reference range across all body weights for the indicated measurements. Source: Adapted from Cornell et al. [14]. Echocardiographic studies are typically obtained by imaging through the thorax, but images can also be obtained with the use of an intraesophageal probe or transesophageal echocardiography (TEE). TEE, by virtue of its closer proximity to the heart, renders highly detailed views of the heart and great vessels and is often used in patients whose chest conformation or body weight precludes high‐quality transthoracic imaging. The high resolution and visual acuity of TEE images facilitate cardiac catheterization procedures, such as device occlusion of PDA or balloon valvuloplasty of valvular pulmonic stenosis (PS). The TEE probe consists of a flexible and steerable shaft (similar to an endoscope) with a phased array ultrasound transducer at its tip. Biplane TEE utilizes two transducers positioned at right‐angles from each other whereas multiplane TEE utilizes a single transducer that can be rotated within the scope’s housing to permit imaging along any plane from 0º to 180º. In veterinary patients, TEE examination is performed under general anesthesia or heavy sedation, and the TEE probe is inserted through a mouth gag into the patient’s oropharynx and esophagus. The probe is positioned over the heart base and multiple imaging planes of the heart can be obtained by switching between the two transducers (biplane) or by rotating the transducer within the probe housing (multiplane) (Fig. 36.2). Excellent reviews of biplane TEE imaging technique and planes in the dog and cat are available [16,17]. The heart is an active endocrine organ. In the case of the sympathomimetic and renin–angiotensin–aldosterone systems, the heart is an end‐organ target of neurohormonal activity, whereas in the case of atrial and B‐type natriuretic peptide (ANP, BNP), the heart is a source of neurohormonal production. Measurement of ANP, BNP, and NTproBNP (a precursor of BNP) can help differentiate cardiac versus respiratory etiology of respiratory signs, detect occult cardiomyopathy, and provide prognostic information [18–21]. In the setting of anesthesia, the natriuretic peptides, in addition to markers of cardiac tissue injury, such as cTnI, are probably most useful in helping to ascertain the risk of occult cardiomyopathy in cats during the preanesthetic work‐up. In selected populations of cats that are at high risk for cardiomyopathy, such as adult cats with a heart murmur, gallop, or arrhythmia, NTproBNP assay detects clinically significant occult cardiomyopathy with moderate sensitivity and specificity [19,20]. Elevated values warrant additional diagnostics such as echocardiography to achieve a definitive diagnosis and help formulate anesthetic recommendations. Natriuretic or troponin assay as part of the preanesthetic work‐up prior to routine elective surgery (e.g., spay or neuter) is not currently recommended as the low prevalence of cardiomyopathy in these populations will result in many false positive results. Animals with pre‐existing cardiac disease often undergo evaluation by a cardiology specialist prior to anesthesia. Depending on findings, cardiologists might make recommendations regarding chronic medications and recheck exams following anesthesia and resolution of the animal’s primary problem. In the authors’ opinion, cardiologists should refrain from routinely including specific anesthesia recommendations as part of their consult barring specific requests from the anesthesiologist. The goal of the preanesthetic cardiology consult is to provide the anesthesiologist with information regarding diagnosis and severity of cardiac disease so that the anesthesiologist can make appropriate plans. Good communication and division of duties between the cardiologist and anesthesiologist are essential for proper patient care. Figure 36.2 Transesophageal echocardiography (TEE) in dogs. A. Dog with congenital pulmonic stenosis showing the incomplete opening and abnormal thickness of the pulmonic valve leaflets from the cranial transverse probe position (RVOT, right ventricular outflow tract; Ao, aorta; PA, pulmonary artery). B. Dog with congenital mitral stenosis showing incomplete opening of the mitral valve leaflets and from the middle transverse position (LA, left atrium; LV, left ventricle; RV, right ventricle; RA, right atrium). When anesthetizing any animal with cardiovascular disease, maintaining CO and tissue perfusion is a primary goal. How that goal is best achieved will differ depending on the underlying cardiac condition. CO is the product of stroke volume and heart rate and decreases in either of these can lead to a low output state. Conversely, fast heart rates, either atrial (e.g., AF) or ventricular in origin, can also lead to a low output state, since diastolic filling times are decreased and/or the atrial contribution (atrial “kick”) to ventricular filling is decreased. To determine accurately the reasons for a decrease in stroke volume, it is important to ascertain whether it is due to a change in preload, afterload (increased systemic vascular resistance [SVR]), and/or contractility of the left ventricle. All of these variables are interdependent. For example, chronic excess preload can cause ventricular over‐distension and systolic dysfunction. Myocardial contractility and stroke volume also may be affected by indirect causes such as pericardial effusion, inflammatory cytokines, and anesthetic agents. Valvular heart lesions and/or septal defects lead to changes in the loading conditions of the heart and the approach to the patient changes accordingly. For example, the left ventricle is volume overloaded in mitral regurgitation and pressure overloaded in subaortic stenosis. Compensatory mechanisms differ between conditions and can include chamber enlargement, myocardial hypertrophy, changes in vascular tone, and alterations in sympathetic activity. Changes in myocardial compliance and chronic myocardial dysfunction may follow. Anesthetic management requires an understanding of the alterations in loading conditions so that compensatory mechanisms may be preserved, and problems may be anticipated and avoided before they occur. Mitral valve regurgitation (MR) can be caused by primary incompetence of the valve leaflets (e.g., myxomatous mitral valve degeneration [MMVD], bacterial endocarditis, congenital malformation) or secondarily to dilation of the annular ring in diseases that cause eccentric hypertrophy secondary to systolic dysfunction (e.g., DCM). MMVD is the most common cause of MR, having a prevalence of approximately 30% in small‐breed dogs over the age of 10 years. Regardless of the initial cause, the presence of MR induces further eccentric hypertrophy and annular dilation in a vicious cycle wherein “MR begets further MR.” MR results in volume overload of the left ventricle and left atrium. With incompetence of the mitral valve, the outflow of the left ventricle is divided between the relatively high‐pressure/low‐compliance outflow tract through the aorta and arterial tree, and the low‐pressure/high‐compliance outflow route across the incompetent mitral valve into the left atrium. This mismatch in afterload conditions can result in MR volumes representing a high percentage of total stroke volume. This regurgitant fraction can be as high as 50–70% of total stroke volume, highlighting the often‐severe degree of volume overload within the left ventricle and atrium. Thoracic radiography is particularly useful in dogs with MMVD such that the radiographic heart size is proportional to the severity of MR. In many cases of uncomplicated MMVD, radiographic inspection of the cardiac silhouette, pulmonary vessels, and pulmonary parenchyma provides sufficient information regarding severity of disease and risk for CHF and helps guide the need for chronic medical therapy. Although the volume work of the left ventricle is increased, left ventricular systolic wall tension is minimally increased, as the presence of MR allows a large percentage of the work to be done at low pressure. Thus, in most small‐breed dogs (body weight < 15 kg) with MR, systolic contractility often appears normal or even hypercontractile when assessed by echocardiography. In larger breed dogs, systolic dysfunction secondary to MR is much more common, for reasons that are poorly understood. Thus, preanesthetic echocardiography to detect systolic dysfunction is often of greater value in large‐ than small‐breed dogs. The time course for the development of MR influences the severity of symptoms. If the MR develops slowly over time, the left atrium compensates with gradual dilation, and some dogs with severe amounts of MR can be asymptomatic. However, if the MR occurs acutely, as in the case of bacterial endocarditis or acute ruptured primary chordae tendinae, the sudden volume overload can lead to a rapid increase in left atrial pressures and severe pulmonary congestion. Chronic medical therapy for MR involves reduction in preload (diuretics such as furosemide and spironolactone), reduction in arterial afterload (vasodilators such as angiotensin‐converting enzyme inhibitors [ACEIs], and pimobendan), and maintenance of adequate systolic contractility (positive inotropes such as pimobendan). Initiation of medical therapy prior to elective anesthesia should be considered for newly diagnosed cases of MR. The primary goal of anesthesia in the patient with MR is to maintain forward aortic flow while minimizing regurgitant flow and development of pulmonary edema (Box 36.1). The volume of regurgitant flow across the mitral valve is related to the size of the regurgitant orifice (which is related to ventricular size), the time available for retrograde flow, and the pressure gradient across the dysfunctional valve [22]. Patients with chronic, compensated MR will be sensitive to changes in ventricular loading conditions and preload should generally be maintained during anesthesia [23]. Overzealous fluid administration should be avoided; however, to avoid ventricular distension and resultant changes in regurgitant flow. Maintenance fluid rates of 2–4 mL/kg/h are generally recommended. Preload reduction may be helpful in some cases. Heart rates should be maintained at a normal or slightly above normal rate to help maintain smaller left ventricular volumes and minimize MR secondary to annular dilation. Bradycardia also increases the duration of systolic contraction and the time available for regurgitant flow. Table 36.4 Inotropic and vasopressor drugs, adrenergic receptor binding, and standard dose range for dogs and cats. Most patients with MR benefit greatly from afterload reduction. By reducing afterload, the left ventricular systolic pressures required for ejection from the ventricle to the aorta and arterial vasculature decrease, optimizing forward flow. This, in turn, decreases the pressure gradient from the left ventricle to the left atrium during systole and decreases regurgitant flow. Adequate depth of anesthesia and anesthetic agents that promote arteriolar dilation and maintenance of heart rate are generally recommended. In contrast, administration of drugs that cause increases in systemic blood pressure and afterload (e.g., α2‐adrenergic receptor agonists) can impair forward flow, increase regurgitant flow, and cause rapid deterioration of patients with MR. In early compensated MR, LV contractility may be preserved, but patients with significant MR may benefit from inotropic support with a drug such as dobutamine (5–15 μg/kg/min) (Table 36.4). Premedication may be required in some cases to decrease the anxiety of the animal and stress during patient handling, minimizing catecholamine release, tachycardia, and increased myocardial work. Ideally, patients should receive supplemental oxygen after receiving sedative medication, but the stress of restraint for oxygen administration should be considered. If preoperative sedation is necessary, opioid receptor agonists are generally the drug class of choice since they usually cause minimal cardiovascular depression at clinically useful doses, with left ventricular contractility, CO, and systemic blood pressure being well maintained. In healthy animals, opioids cause behavioral changes ranging from sedation to excitement; however, in depressed or critically ill patients, opioids usually cause sedation. Opioids may cause dose‐dependent bradycardia by stimulation of the vagal nerve [24]. Heart rate should be monitored and an anticholinergic drug such as glycopyrrolate or atropine administered if beneficial. Some animals with MR may also have pulmonary hypertension (PHT) and right heart failure. Increased carbon dioxide secondary to opioid‐induced hypoventilation can increase pulmonary pressures and may be of concern in those animals with PHT. A combination of an opioid receptor agonist (e.g., fentanyl, methadone, or hydromorphone) or agonist/antagonist (e.g., butorphanol and buprenorphine) and a benzodiazepine tranquilizer (diazepam or midazolam) may be used to sedate patients for chest radiographs or echocardiography. This combination provides sedation with minimal cardiovascular depression [25–27]. The use of fentanyl may be more likely to cause clinically significant bradycardia. Reversal may be accomplished by using an opioid receptor antagonist such as naloxone and/or a benzodiazepine antagonist such as flumazenil. Phenothiazine tranquilizers such as acepromazine in low doses may be beneficial in some patients with MR because they calm the patient, decrease afterload, and decrease the incidence of arrhythmias. Acepromazine should only be used after careful consideration; however, since α1‐adrenergic receptor antagonism may cause hypotension, not only by decreasing SVR, but also by causing venodilation and decreased preload. In conscious dogs, intravenous acepromazine (0.01–0.05 mg/kg) decreased mean arterial pressure (MAP) and stroke index, but did not significantly change SVR, heart rate, or cardiac index [28]. In general, a carefully titrated anesthetic induction is preferred in the cardiac patient with continuous monitoring of cardiovascular parameters. Although no one best technique exists, a high‐dose opioid technique including an opioid and a benzodiazepine tranquilizer with or without low‐dose propofol, etomidate, and/or alfaxalone is often advocated for induction to maintain systolic function in patients with severe myocardial dysfunction. Premedicaton with maropitant 30–60 min prior to induction is helpful in preventing emesis if hydromorphone or etomidate are being used [29]. MR patients with minimal systolic dysfunction may be anesthetized with other protocols using propofol or alfaxalone, (remembering to avoid excessive increases in SVR). The primary advantages of opioids are lack of myocardial depression and maintenance of hemodynamic stability. Most opioid receptor agonists, with the exception of meperidine, lack negative inotropic effects with clinically used doses. Hydromorphone, methadone, fentanyl, or synthetic fentanyl derivatives such as remifentanil [30–34] are usually preferred. μ‐Opioid receptor agonists such as fentanyl and its analogs, although relatively free of direct cardiovascular effects, can cause a significant vagally mediated bradycardia that would result in decreased CO. The extent of the bradycardia is dependent on the dose and rate of drug administration, and the use of any opioid in high doses can produce excessive bradycardia. Some anesthesiologists prefer to administer anticholinergics such as glycopyrrolate prior to high‐dose opioids used during induction to avoid extreme swings in heart rate. Vagally mediated bradycardia induced by fentanyl (e.g., at 5 μg/kg IV) can sometimes be useful in ameliorating the sympathomimetic effects of other induction agents such as alfaxalone and help prevent significant changes in heart rate during induction. Other adjunctive drugs are commonly used, including benzodiazepine tranquilizers such as midazolam or diazepam. A low dose of propofol given as a slow infusion is often used as an induction agent in patients with MR or other cardiac disease. Propofol acts as a vasodilator and can decrease afterload while maintaining heart rate. Rapid administration of large doses of propofol should generally be avoided owing to potentially profound cardiovascular effects, including vasodilation, decreased cardiac contractility, and hypotension [35–41]. In patients with poor systolic function, propofol induction requirements can be reduced by concurrent administration of other drugs in a balanced induction protocol, including an opioid, a benzodiazepine, and/or etomidate. Co‐administration of propofol and etomidate decreases the required doses of each individual drug. While one study found that midazolam co‐induction decreased the induction dose of propofol and improved the quality of induction, it did not improve cardiopulmonary variables in critically ill dogs [40]. Etomidate is a useful anesthetic induction agent in any animal with cardiac disease associated with poor systolic function [42–45]. Left ventricular pressures, mean aortic pressure, and coronary blood flow do not change significantly in healthy dogs receiving up to 2.5 mg/kg for induction. Etomidate is not arrhythmogenic and may also be useful in animals with cardiac conduction abnormalities. The addition of midazolam decreases the etomidate induction dose required with no change in cardiopulmonary function [45]. An opioid is also used as part of this induction protocol. Alfaxalone has a rather large therapeutic index and can also be used to induce animals with heart disease, although dose‐dependent adverse effects such as tachycardia can be seen after larger doses are given rapidly [46–51]. The administration of fentanyl prior to alfaxalone can decrease induction requirements and ameliorate changes in heart rate. Maintenance of anesthesia can be achieved with an opioid (e.g., fentanyl or remifentanil), propofol infusion, and/or alfaxalone infusion (or some combination of these) with or without an inhalant agent. Etomidate infusions are not recommended owing to the potential for hemolysis associated with the high osmolality of the commercially available formulations and also concerns related to prolonged adrenocortical suppression [52–54]. A single bolus injection of 2 mg/kg etomidate reduces the adrenocortical response to anesthesia and surgery for 2–6 h, and longer infusions have been associated with prolonged adrenocortical suppression and higher mortality rates in critically ill multiple‐trauma patients [55]. The hemodynamic changes associated with dexmedetomidine administration include bradycardia and increased SVR and are generally to be avoided. When given as a constant rate infusion without a loading bolus, plasma concentrations are lower and may reduce cardiovascular side effects, although increased blood pressure and decreased heart rate still occur [56]. Use of vatinoxan (MK‐467), a peripheral α2‐adrenergic receptor antagonist, in combination with medetomidine may reduce the increase in SVR and may expand the potential utility of α2‐adrenergic receptor agonists in patients with myocardial disease. However, further research to establish the safety of this combination in patients with heart disease is needed. Most of the commonly used inhalant agents (e.g., isoflurane, sevoflurane, and desflurane) tend to preserve myocardial contractility at light anesthetic planes. The advantages of inhalant anesthetics for maintenance of anesthesia include their dose‐dependent cardiovascular effects, the ability to rapidly adjust the depth of anesthesia, and their suppression of sympathetic responses associated with surgical stress. Disadvantages include myocardial depression, systemic hypotension (due to decreased contractility or vasodilation), and lack of postoperative analgesia. Mild decreases in SVR coupled with adequate systolic function may reduce regurgitant fraction and are goals of anesthetizing patients with MR. Decreased preload and decreased systolic function associated with deep planes of inhalant anesthesia are not recommended; therefore, multimodal “balanced” anesthetic techniques are often advocated. Patients on medication for heart disease are generally continued on their medications (especially diuretics, calcium channel blockers, antiarrhythmics, and inotropic drugs) until the time of anesthesia. Pimobendan acts as both a positive inotrope (by increasing calcium binding to the myofibrils) and as a vasodilator (by acting as a phosphodiesterase 3 inhibitor) and is usually given on the day of anesthesia. However, hypotension may be more pronounced under anesthesia in patients receiving ACEIs such as enalapril and some anesthesiologists prefer to delay giving enalapril until after recovery. It is always prudent to have inotropic and venoconstrictor agents immediately available for the management of intraoperative hypotension since fluid administration is often contraindicated. Dilated cardiomyopathy (DCM) is defined as idiopathic systolic dysfunction and is accompanied by eccentric dilation and volume overload as the heart’s generation of normal forward stroke volume decreases. The resulting left ventricular dilation involves the mitral valve annulus creating secondary MR, which further contributes to volume overload. DCM is common in large‐breed dogs, and in particular the Doberman Pinscher, Irish Wolfhound, and Great Dane. DCM is relatively uncommon in cats following the recognition of taurine deficiency and subsequent reformulation of commercial diets. Taurine deficiency is occasionally seen in dogs, in particular, the Cocker Spaniel. Some dogs receiving grain‐free diets are also noted to have moderate‐to‐severely decreased contractility. DCM predisposes to CHF in addition to ventricular arrhythmias. In most cases, heart failure is left‐sided (pulmonary edema) but can occasionally be right‐sided (ascites, pleural effusion). Frequent ventricular arrhythmias can cause activity intolerance, syncope, and sudden cardiac death. A combination of echocardiography, thoracic radiography, and ECG is typically used to diagnose and stage the severity of DCM. Holter monitoring is commonly performed to screen at‐risk dogs for ventricular premature beats, which is an early sign of disease. Chronic therapy is targeted toward reduction of preload (diuretics such as furosemide and spironolactone), reduction of afterload (ACEIs and pimobendan), and increased contractility (positive inotropes such as pimobendan and digoxin). Suppression of ventricular arrhythmias is performed acutely using lidocaine or procainamide continuous rate infusion (CRI), and chronically using sotalol or amiodarone. β‐Adrenergic receptor antagonists (β‐blockers) are occasionally used as an adjunctive ventricular antiarrhythmic but, owing to their negative inotropic effects, caution should be exercised in animals with severe systolic dysfunction or active CHF. Many cases of DCM are further complicated by the development of AF. AF is particularly common in Great Danes, Mastiffs, Irish Wolfhounds, and other giant breed dogs with DCM. The rapid ventricular rate associated with AF increases myocardial oxygen demand and reduces CO as diastolic filling time decreases. Hence, therapy of AF involves reduction of AV conduction and slowing of ventricular heart rate. The most common agents used include digoxin, calcium channel blockers (e.g., diltiazem), and β‐blockers. Many dogs with early DCM are asymptomatic. Older, large‐breed dogs that have occasional ventricular early depolarizations (VPCs) before or during anesthesia, which do not resolve following anesthesia, may benefit from examination by a cardiologist. Some cases of occult DCM are only diagnosed after unexplained VPCs are noticed during and after anesthesia, prompting consultation with a cardiologist. Anesthetic considerations for DCM patients are similar to those of animals with MR, with the added concerns of decreased systolic function (which may be severe), CHF, and increased incidence of cardiac arrhythmias. Additionally, dogs with cardiomyopathy often have a significant downregulation of β‐adrenergic receptors in the heart, although receptor affinity does not change [57], making these animals more resistant to treatment with a positive inotrope. Dogs with significant systolic dysfunction probably benefit from inotropic drug support (e.g., dobutamine or dopamine) during anesthesia. Ventricular arrhythmias can be seen during anesthesia in animals with DCM. Antiarrhythmic drugs such as lidocaine and procainamide should be available but are often reserved for cases where the frequency of abnormal beats creates significant blood pressure or CO decreases. Although often unnecessary, esmolol, a short‐acting β‐blocker, may be given as an infusion as indicated (0.5 mg/kg loading bolus followed by an infusion of 0.01–0.2 mg/kg/min to reduce the frequency of ventricular premature contractions associated with sympathetic stimulation). The dose rate may be adjusted as needed to minimize any negative inotropic and bradycardic effects associated with β‐receptor blockade. In addition to blood pressure monitoring (direct or indirect), monitoring of end‐tidal CO2 can be helpful under anesthesia, as a secondary means of monitoring CO. In dogs with concurrent DCM and AF, electrocardioversion may be considered (if the patient is a candidate) at the beginning of anesthesia. The increased ventricular filling following resynchronization of the atria and ventricles can improve CO. Alternatively, drugs that slow the sinus rate and conduction through the AV node, such as fentanyl, can be used during anesthesia. Anticholinergic agents are to be avoided in dogs with AF but may be useful for dogs with DCM without AF that are bradycardic under anesthesia. Fentanyl at a dose of 5–40 μg/kg/h can be an effective anesthetic in sick dogs when used as a primary agent in combination with oxygen and ventilation in those animals that do not tolerate inhalant anesthetics. Remifentanil has an elimination half‐life shorter than that of fentanyl, with rapid recovery times regardless of the duration of infusion, although either opioid is suitable for an infusion technique. Infusion of remifentanil at 0.25–0.5 μg/kg/min with propofol at 0.2 mg/kg/min produces little change in mean arterial blood pressures in healthy dogs, although the heart rate decreases with increasing doses of remifentanil [34]. Both ventricular septal defect (VSD) and patent ductus arteriosus (PDA) permit left‐to‐right shunting, which produces volume overload of the pulmonary circulation and left heart. As the physiologic response to the increased pulmonary circulation progresses, pulmonary and right ventricular pressures may increase, resulting in minimal or reverse flow (right‐to‐left shunt). Anesthesia without consideration of drug selection and patient management on SVR (and blood pressure) and pulmonary and right ventricular pressure may result in reverse shunt flow and rapid deterioration of the patient’s condition. Careful echocardiographic evaluation prior to anesthesia and careful perianesthetic monitoring of patients can facilitate anesthetic management of patients with cardiovascular shunts. Many VSDs in dogs and cats are relatively small and the volume of the shunt is not sufficient to cause CHF. In contrast, the majority of animals with PDA will suffer from left‐sided heart failure within the first year of life if the defect is not corrected. PDA is common in dogs and less so in cats, whereas VSD is more common in cats than in dogs. Both PDA and VSD generate characteristic murmurs that often permit a presumptive diagnosis based on the physical examination findings alone. In both diseases, a combination of echocardiography and thoracic radiography facilitates a definitive diagnosis and permits assessment of shunt volume and risk for heart failure. In general, the extent of left ventricular eccentric hypertrophy and degree of left atrial dilation reflect the magnitude of the shunt. The extent of shunting depends not only on the cross‐sectional area of the lesion, but also on the relative pressures and compliance of the right and left portions of the circulation. Low pressure and compliance of the pulmonary circulation and right ventricle relative to the systemic circulation and left ventricle promote left‐to‐right shunting. Interventions that either increase right‐sided pressure or decrease left‐sided pressure decrease the shunt severity, at least to the point where flow reverses through the shunt when hypoxemia and polycythemia can develop. Hence systemic arterial vasodilators can be used to reduce the shunt volume, as can surgical interventions such as pulmonary artery banding, which increase pressure within the right ventricle. In animals with VSD or PDA, the degree and magnitude of shunt flow are dependent upon relative outflow resistances, which are determined by the size of the defect or ductus and the difference between systemic and pulmonary resistance. With simple left‐to‐right shunting, pulmonary blood flow is increased, and the right ventricle and pulmonary vasculature can be pressure or volume overloaded; some degree of pulmonary hypertension (PHT) may be present. Further increases in pulmonary blood flow are avoided by preventing increases in SVR. In addition, manipulations that decrease pulmonary vascular resistance (PVR) (hypocapnia, alkalemia, spontaneous ventilation, and deep anesthesia) can also increase the magnitude of left‐to‐right shunt flow. In many cases, heart failure is not yet present, and the animal is relatively young and active and may become excited with restraint. Almost any anesthetic agent that does not significantly alter SVR may be used safely for premedication, induction, or maintenance. Drugs that significantly increase SVR, including the α2‐adrenergic receptor agonists (e.g., dexmedetomidine) are generally avoided. Repair of PDA may be accomplished by surgical ligation or transvascular occlusion, with the rate of survival being similar between the two techniques [58], although arrhythmias and hypotension are commonly reported [59]. If not corrected within the first year, a significant percentage of dogs with PDA will develop CHF. For those cases in which some degree of CHF is present, considerations are similar to those for patients with volume overload (see earlier). An opioid‐based induction technique with benzodiazepine tranquilizer and etomidate, alfaxalone, or propofol followed by inhalant anesthesia or propofol infusion, or a combination of fentanyl/remifentanil and propofol infusion can all be used [33,58,59]. Propofol can result in clinically significant changes in cardiac shunt direction and arterial desaturation; however, due to a decrease in SVR [60]. This decrease is usually dependent on the dose and rapidity with which the propofol is given. Diastolic pressures are often low in these patients owing to the shunting of blood from the systemic circulation to the lower resistance pulmonary circulation. A characteristic change in the arterial waveform may be seen with ligation of the PDA, including a decrease in the pulse pressure, increase in mean and diastolic pressures, and reappearance of a dicrotic (reflected) wave (Fig. 36.3). Hypotension may be pronounced in some patients due to the low diastolic pressures and is generally treated with the use of an inotrope such as dobutamine (see Table 36.4), which helps maintain myocardial function and heart rate while avoiding increases in SVR. However, significant decreases in SVR must also be avoided, especially in those patients with significant PHT in which shunt flows may reverse in the face of inhalant anesthetic‐associated arteriolar dilation. These patients will benefit from the administration of a vasoconstrictor (e.g., dopamine or phenylephrine; see Table 36.4) to maintain baseline SVR. A sudden decrease in arterial oxygen saturation due to venous admixture as monitored with a pulse oximeter or arterial blood gas may be an early indicator of shunt reversal. If surgery is to be performed on a relatively young animal for repair of a congenital heart defect, the progressive maturation of the autonomic nervous system and cardiovascular responses must be taken into account. In puppies, the adrenergic vasoconstrictor response is not fully developed until after 8 weeks of age [61]. Similarly, although chronotropic responsiveness is apparent at 1 week, the inotropic response to sympathetic stimulation does not fully develop until after 8 weeks of age [62]. Neonatal patients typically have a higher heart rate and lower arterial blood pressure and SVR than adults. In addition, the cardiovascular response to sympathomimetic agents may be less pronounced in the neonatal or pediatric patient than the adult animals of similar species [63–65]. Figure 36.3 Output from a patient monitor during occlusion of a patent ductus arteriosus (PDA) showing the electrocardiogram and arterial pressure waveform. Notice the rapid increase in diastolic arterial blood pressure and the appearance of a dicrotic (reflected) wave during diastole immediately following occlusion (blue arrow). These changes are characteristic of decreased flow through the PDA and can be used to help identify the vessel during surgery. Tetralogy of Fallot (TF) and reverse patent ductus arteriosus (rPDA) are uncommon congenital heart defects in dogs and are rare in cats. The pathophysiology of both TF and rPDA involves right‐to‐left shunting and systemic hypoxemia. In the case of rPDA, concurrent pulmonary hypertension (PHT) is present. TF is caused by defects in the fetal conotruncal formation resulting in a dextro‐ or rightward‐positioned aortic root, VSD, PS/pulmonic hypoplasia, and secondary right ventricular concentric hypertrophy. The presence of the pulmonic lesion creates pressure overload of the right ventricle sufficient to cause right ventricular to left ventricular shunting through the VSD. The dextropositioned aorta further contributes to admixture of unoxygenated blood into the systemic circulation. rPDA is characterized by a large cylindrical ductus arteriosus and the development of progressive PHT over the animal’s first 6–8 months of life. The PHT increases pulmonary artery pressures sufficiently to “reverse” the flow through the ductus arteriosus and shunt blood from the pulmonary circulation to the systemic circulation. Animals with TF or rPDA often display poor growth, activity intolerance, weakness, fainting, and cyanosis. Echocardiography and radiography help provide a definitive diagnosis and assessment of disease severity. ECG abnormalities commonly include right axis shift, interventricular conduction abnormalities, ST segment changes, and occasionally ventricular arrhythmias. The degree of hypoxemia can be assessed through pulse oximetry or arterial blood gas analysis. Chronic hypoxemia stimulates red blood cell production and polycythemia is an important component of the pathophysiology of right‐to‐left defects. Clinical signs in animals with TF or rPDA are usually due to hypoxemia and polycythemia, and CHF is very rare in both conditions. A packed cell volume (PCV) as high as 70–75% is not uncommon, and activity intolerance, organ dysfunction, and clotting abnormalities commonly occur at these extreme values. Periodic phlebotomy is used to maintain PCV at or below 65%. The balance between the right‐ and left‐sided pressures affects the extent of shunting, and interventions that lower SVR such as arterial vasodilators can potentiate signs. Signs of weakness or cyanosis are common during attempted exercise as systemic arterial vasodilation to the animal’s skeletal muscle occurs. Affected animals are sometimes prescribed non‐selective β‐blockers such as propranolol to try and limit the amount of β2‐adrenergic receptor‐mediated vasodilation during activity. Goals of anesthesia for the patient with TF or other reverse shunt disorders are to maintain SVR and arterial pressures while minimizing changes in PVR and pulmonary pressures. As right‐to‐left shunting increases so does arterial oxygen desaturation. Patients with significant PHT can be sensitive to changes in preload. Hypotension secondary to hypovolemia or vasodilation should be avoided and treated immediately if it occurs. Premedication with an intramuscular opioid may be useful as struggling and sympathetic stimulation with restraint can increase right‐to‐left flow. These drugs should, however, be used judiciously as hypoventilation can lead to hypercarbia and respiratory acidosis, which can increase PVR and reverse shunting. Hypoxemia and hypotension can occur quickly in these patients. Preoxygenation prior to induction of anesthesia is extremely important, not only to address the underlying chronic hypoxemia that occurs in these animals, but also as a means of decreasing PVR. Other manipulations that can help decrease PVR include lowering the hematocrit to < 60% prior to anesthesia and hyperventilation with the maintenance of relative hypocarbia and respiratory alkalosis during anesthesia. Care must be taken with controlled ventilation, as increases in intrathoracic pressure with mechanical ventilation or the use of positive end‐expiratory pressure can increase PVR and the magnitude of right‐to‐left shunt flow. Patients with TF often have a dynamic component to the stenotic lesion and increases in heart rate may exacerbate the pulmonary outflow obstruction and reverse shunting [23]. An opioid‐based induction technique is often used with or without etomidate, although significant decreases in heart rates should be avoided, especially if fentanyl or remifentanil is used. All catheters and injection ports should be cleared of bubbles to avoid systemic air embolization. SVR must be maintained in these patients, as excessive hypotension will increase the right‐to‐left flow. Phenylephrine, an α‐adrenergic receptor agonist that causes arterial and venous vasoconstriction, can be useful in reversing right‐to‐left shunting during anesthesia, although other pressor agents such as norepinephrine or dopamine may also be used. Subaortic stenosis (SAS) and pulmonic stenosis (PS) are common congenital valvular defects in the dog. SAS and PS lead to a chronic systolic pressure increase in the left or right ventricle, respectively, with a resultant increase in wall tension and a compensatory increase in ventricular wall thickness or concentric hypertrophy. The increase in ventricular muscle mass and the increase in myocardial work required to generate increased systolic pressures result in an increased demand for coronary blood flow and myocardial oxygen delivery. In theory, the resulting risk for ischemia is greatest during periods of tachycardia and leads to myocardial necrosis, replacement fibrosis, and development of ventricular arrhythmias. In young dogs with severe SAS, the risk for syncope or sudden arrhythmic death is high, whereas the risk of left‐sided CHF is typically recognized in much older dogs. Right ventricular concentric hypertrophy, even in cases of severe PS, appears to be better tolerated than cases of left ventricular hypertrophy. Sudden death in dogs with severe PS is relatively uncommon, although clinical signs such as activity intolerance or syncope occur. In the authors’ experience, CHF, in the form of ascites, is more common in dogs with PS. Chronic medical management of severe SAS or PS often involves β‐blockers to reduce heart rate and myocardial oxygen demand. Echocardiography is an extremely useful modality to assess the severity of the SAS or PS lesion and the extent of concentric hypertrophy. ECG, 24‐h ambulatory ECG (Holter) monitoring, and cardiac troponin assay are useful to evaluate cardiac rhythm and to detect ST segment elevation or depression, which is suggestive of myocardial ischemia. In both SAS and PS, arterial vasodilators should be used with caution as a decrease in arterial blood pressure increases the pressure gradient across the stenotic valve and can lead to increased myocardial work, severe hypotension, and decreased coronary perfusion pressure. Patients with SAS or PS often have poor ventricular compliance; thus, it is critically important to maintain adequate preload in these animals. Maintaining an adequate venous return by insuring a full intravascular volume to fill the non‐compliant ventricular chamber will help optimize diastolic filling and CO, although volume overload should be avoided. In addition, these patients are dependent on maintenance of a normal sinus rhythm and atrial “kick” to optimize ventricular filling. Tachycardia must be avoided to limit myocardial oxygen deficit (Box 36.2). The stenosis may also have a dynamic component, becoming more pronounced at rapid heart rates.
36
Pathophysiology and Anesthetic Management of Patients with Cardiovascular Disease
Introduction
Preanesthetic evaluation
Electrocardiography
Thoracic radiography
Species/Breed
n
Mean (SD)
Comments
Canine/Beagle [7]
19
10.3 (0.4)
Canine/Greyhound [8]
42
10.5 (0.1)
Canine/Whippet [9]
44
11.0 (0.5)
Right lateral recumbency
11.3 (0.5)
Left lateral recumbency
Canine/Rottweiler [9]
38
9.8 (0.1)
Canine/Cavalier King Charles Spaniel [6]
10
10.8 (0.49)
Canine/mixed [10]
63
9.8 (0.6)
Right lateral recumbency
9.5 (0.8)
Left lateral recumbency
Canine/puppies [11]
11
10.0 (0.5)
3 months
9.8 (0.4)
6 months
9.9 (0.6)
12 months
6
10.3 (0.6)
36 months
Canine/Boxer [12]
20
11.6 (0.8)
Canine/Labrador Retriever [12]
25
10.8 (0.6)
Canine/German Shepherd [12]
20
9.7 (0.7)
Canine/Doberman [12]
20
10.0 (0.6)
Canine/Cavalier King Charles Spaniel [12]
20
10.6 (0.5)
Canine/Yorkshire Terrier [12]
22
9.7 (0.5)
Canine/mixed [4]
100
9.7 (0.5)
Feline/mixed [13]
50
7.3 (0.49)
Right lateral recumbency
7.3 (0.55)
Left lateral recumbency
Feline/mixed [5]
100
7.5 (0.3)
Echocardiography
Measurement
Commonly used abbreviation(s)
Left ventricle at end‐diastole
Left ventricular end‐diastolic dimension; values that are indexed or normalized to the animal’s body weight commonly utilize an “i” or “n” (i.e., iLVEDD or LVIDdN)
LVEDD, LVIDd, LVd
Thickness of the left ventricular posterior wall
LVPWd
Thickness of the interventricular septum
IVSd
Left ventricle at end‐systole
Left ventricular end‐systolic dimension; values that are indexed or normalized to the animal’s body weight commonly utilize an “i” or “n” (i.e., iLVESD or LVIDsN)
LVESD, LVIDs, LVs
Thickness of the left ventricular posterior wall
LVPWs
Thickness of the interventricular septum
IVSs
Diameter of the aortic root
AoD
Diameter of the left atrium
LAD
Ratio of the aortic to left atrium diameter
LA:Ao, LAD:AoD
Fractional shortening [(LVEDD – LVEDSD)/LVEDD] × 100%
FS%
Measurement
95% CI boundary
Formula (BW in kg)
iLVIDd
1.35–1.73
iLVIDd = LVIDd/BW0.294
iLVIDs
0.79–1.14
iLVIDs = LVIDs/BW0.315
iIVSd
0.33–0.52
iIVSd = IVSd/BW0.241
iIVSs
0.48–0.71
iIVSs = IVSs/BW0.240
iLVPWd
0.33–0.53
iLVPWd = LVPWd/BW0.232
iLVPWs
0.53–0.78
iLVPWs = LVPWs/BW0.222
iLAD
0.64–0.90
iLAD = LAD/BW0.345
iAoD
0.68–0.89
iAoD = AoD/BW0.341
Blood‐based cardiac markers
Cooperation between the cardiologist and anesthesiologist
Anesthesia for specific conditions
Conditions associated with volume overload
Mitral valve insufficiency/regurgitation
Anesthetic considerations
Drug
α1
α2
β1
β2
Dose
Comments
Epinephrine
5+
3+
4+
2+
0.01–1 μg/kg/min
Primarily β effects at lower doses; increasing α effects at higher doses
0.02–0.2 mg/kg bolus
For cardiac arrest
Norepinephrine
5+
5+
3+
0/1+
0.01–0.1 μg/kg/min
β2 effect not evident clinically
Dobutamine
0/1+
0
4+
2+
5–15 μg/kg/min
Positive inotropic effects, β2 effect decreases afterload
Phenylephrine
5+
2+
0
0
0.2–2 μg/kg/min
Dopamine
1–5+
2+
3+
2+
Actions are dependent on dose:
1–3 μg/kg/min
Acts primarily on dopamine‐1 receptors in renal and splanchnic vasculature to produce vasodilation
5–10 μg/kg/min
Primarily β effects
> 10 μg/kg/min
Primarily α effects
Ephedrine
2+
1+
3+
1+
0.05–0.5 mg/kg
Primary action by an indirect effect; direct and indirect effects (norepinephrine release)
Dilated cardiomyopathy
Anesthetic considerations
Left‐to‐right shunting congenital defects – ventricular septal defect and patent ductus arteriosus
Anesthetic considerations
Right‐to‐left shunting congenital defects
Tetralogy of Fallot and reverse patent ductus arteriosus
Anesthetic considerations
Conditions associated with pressure overload
Subaortic stenosis and pulmonic stenosis
Anesthetic considerations
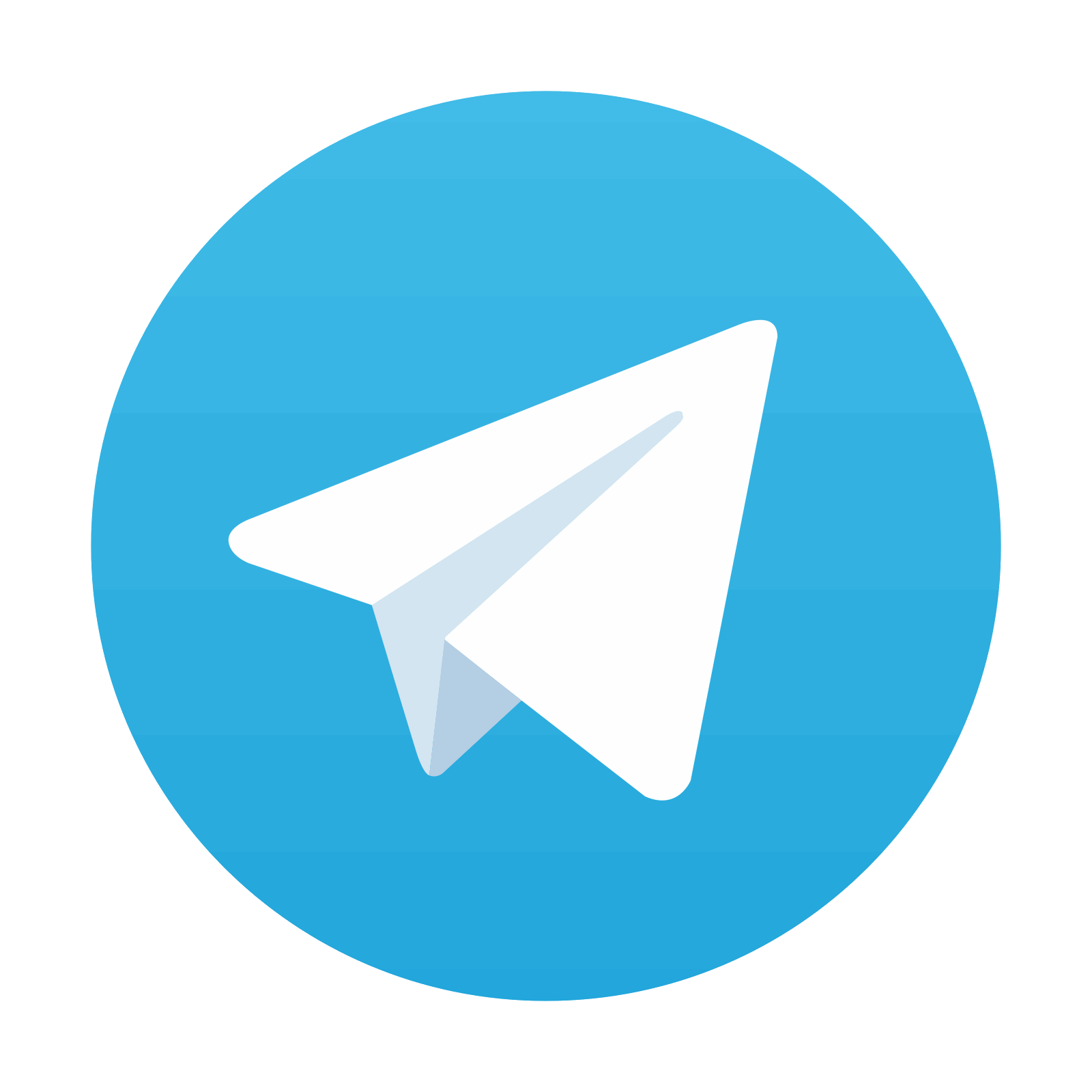
Stay updated, free articles. Join our Telegram channel

Full access? Get Clinical Tree
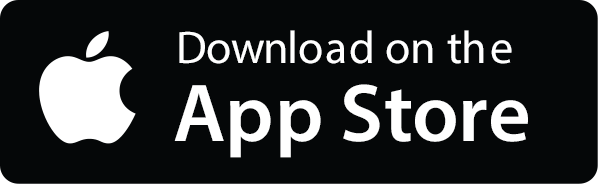
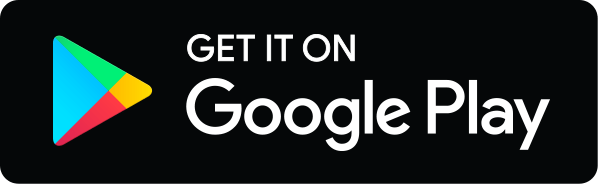