Chapter 24 Gregg P. Adams and Jaswant Singh Veterinary Biomedical Sciences, Western College of Veterinary Medicine, University of Saskatchewan, Saskatoon, Saskatchewan, Canada The intent of this chapter is to provide an overview of our present understanding of ovarian function in cattle, with emphasis on the dynamics and control of follicular and luteal gland development. The following sections are arranged, more or less, in chronological order beginning with fetal ovarian development and follicle assembly, early folliculogenesis and oogenesis, antral follicle dynamics during the estrous cycle, ovulation, and development of the corpus luteum. A following section includes a description of ovarian dynamics during different reproductive states (i.e., prepubertal and peripubertal periods, pregnancy and the postpartum period, and during old age). Lastly, reference is made to implications of our new-found knowledge of ovarian function on clinical and breeding management practices in cattle, particularly on the design of modern protocols for ovarian synchronization and superstimulation. The gross, ultrasonographic, and histologic morphology of the mature bovine ovary is illustrated in Figures 24.1 and 24.2. Similar to most mammalian species (except the horse), the bovine ovary is arranged with an outer cortex containing the transient corpus luteum and thousands of follicles at differing stages of development, and an inner medulla composed primarily of connective tissue, vessels, nerves, and the rete ovarii. Figure 24.1 Left and right ovaries from a cow in proestrus (top to bottom: surface view, cut surface, ultrasound image, and histological section stained with H&E). In the left ovary, two large antral follicles are apparent side by side, a regressing former dominant follicle (on the left), and the preovulatory dominant follicle (on the right). In the right ovary, a regressing corpus luteum is the predominant structure. The scale bar is 5 mm for all images. Figure 24.2 Histological section (stained with H&E) and a diagram of the ovary of a cow at day 18 of the estrous cycle (day 0 = ovulation) showing structures of the outer cortex (regressing corpus luteum, preovulatory and subordinate follicles, corpus albicans) and the inner medulla (rete ovarii, blood vessels, connective tissue). The ovarian primordium develops from an elongated gonadal ridge on the ventral aspect of the embryonic kidney (mesonephros). Recent immunohistochemical studies of bovine ovaries suggest that the ovarian primordium does not initially have a distinct surface epithelium with a basement membrane separating it from the underlying stroma, but instead is composed of a cluster of “gonadal ridge epithelial-like” (GREL) cells1 (Figure 24.3). The GREL cells likely develop from the surface epithelium of the mesonephros and are precursors of both granulosa cells of future follicles and the surface epithelium of the future ovary. Primordial germ cells (PGC) migrate from the yolk sac/developing hindgut to the dorsal celomic wall of the embryo via the dorsal mesentery to colonize the gonadal ridge. The PGC undergo massive mitotic proliferation and intermingle with the resident GREL cells. Invading fingers of stroma (“cell streams”) from the underlying mesonephros penetrate the gonadal ridge/ovarian primordium and separate the clumps of PGC and associated GREL cells into ovigerous cords that radiate from the surface epithelium toward what will become the ovarian medulla at the base of the ovary.2,3 The developing medulla contains stroma, blood vessels, and mesonephric tubules that will persist in the adult ovary as the rete ovarii. The penetrating stroma has a basal lamina at its leading edge, hence the ovigerous cords become surrounded by a basal lamina. As the ovary develops, the ovigerous cords break down into smaller groups of PGC/GREL cells resulting in the formation of primordial follicles containing an oocyte surrounded by flattened granulosa cells separated from surrounding tissue by a basal lamina. After forming a primordial follicle, mitotic division of the oogonium ceases, it enlarges, and becomes arrested in the early stages of Meiosis I. The first follicles form close to the medulla while the outer cortex still contains shorter ovigerous cords. The gradual progression of follicle formation extending from the medulla to the surface has been observed in fetal ovaries of human, cattle and sheep, and in postnatal rat ovaries (reviewed in Ref. 1). At later stages of ovarian development, when preantral and antral follicles appear, the cells on the ovarian surface become single-layered and the stroma underlying the surface thickens to become the tunica albuginea. Figure 24.3 Model of bovine fetal ovarian development and follicle assembly (from Hummitzsch et al.1) and chronology of early folliculogenesis and oogenesis. (a) Ovary development commences at the mesonephric surface epithelium (yellow) in the location of the future gonadal ridge. (b) Some surface epithelial cells change phenotype into gonadal ridge epithelial-like (GREL) cells (yellow-blue). (c) The GREL cells proliferate and the basal lamina underlying the surface epithelium breaks down allowing stromal cells (green) to penetrate into the gonadal ridge. (d) GREL cells continue to proliferate and primordial germ cells (gray) migrate into the gonadal ridge between the GREL cells. The mesonephric stroma, including vasculature (red), continues to penetrate and expand in the ovary. (e) Oogonia proliferate and the stroma penetrates further toward the ovarian surface, thus enclosing oogonia and GREL cells into ovigerous cords. The cords are surrounded by a basal lamina at their interface with the stroma, but are open to the ovarian surface. (f) Compartmentalization into cortex and medulla becomes obvious. The cortex is characterized by alternating areas of ovigerous cords and stroma, whereas the medulla is composed of stromal cells, vasculature, and tubules originating from the mesonephros (rete ovarii). As the stroma penetrates toward the periphery, the GREL cells at the surface of the gonadal ridge are aligned by a basal lamina at their interface with the stroma and begin to differentiate into typical ovarian surface epithelium (yellow cells). (g) Ovigerous cords are partitioned into smaller cords and eventually into individual primordial follicles as the GREL cells mature to granulosa cells (blue) and surround an oogonium. (h) Finally, the surface epithelium becomes mostly single-layered and a tunica albuginea, densely packed connective tissue, develops from the stroma below the basal lamina of the surface epithelium. Some primordial follicles become activated and commence development into primary and preantral follicles. Folliculogenesis is the developmental process in which an activated primordial follicle develops to a preovulatory size through the growth and differentiation of the oocyte and its surrounding granulosa cells.4–6 Ovarian follicles have been classified in various ways based on morphologic characteristics, such as the number of granulosa cell layers surrounding the oocyte, the morphologic features of the granulosa cells, the diameter of the oocyte and the follicle, and the absence or presence of a fluid-filled antrum, but in general may be categorized as primordial, primary, secondary, or tertiary (antral or vesicular) (Table 24.1).7–9 Table 24.1 Characteristics of bovine ovarian follicles during development. a Largest cross-section of the follicle defined by the nucleolus of the oocyte. b Oocyte commenced growth when there were at least 40 granulosa cells in the largest cross-section (fourth generation of follicle cells). c Beginning of antrum formation was observed in follicles with at least 250 granulosa cells in the largest cross-section. Initiation of follicular growth, referred to as activation, begins with the transformation of the flattened pre-granulosa cells of the primordial follicle into a single layer of cuboidal granulosa (follicular) cells, at which point the follicle is referred to as a primary follicle.8,10 Proliferation of granulosa cells results in an increase in the number of layers around the oocyte. A follicle with two to six layers of granulosa cells is referred to as a secondary follicle, and a follicle with more than six layers of granulosa cells and a fluid-filled antrum is referred to as a tertiary (or antral) follicle.7,8 The diameter of a primordial follicle is about 0.04 mm and the diameter of the smallest antral follicle is 0.25 mm.7 The largest antral or Graafian follicle becomes the ovulatory follicle following the preovulatory gonadotropin surge.8 In cattle, primordial, primary, secondary and tertiary follicles first appear at days 90, 140, 210, and 250 of gestation, respectively.11 The time required for a follicle to grow from the large preantral stage (secondary follicle) to a mature ovulatory size has been estimated to be about 42 days7 (Table 24.2 and Figure 24.4). Table 24.2 Developmental rates of bovine ovarian follicles. Figure 24.4 Histological section (stained with H&E) and transmission electron micrograph of the wall of the dominant follicle during (a, b) the growing phase (3 days after ovulation) and (c) the early static phase (6 days after ovulation, right) in cattle. The granulosa cells of the growing dominant follicle have many cytoplasmic processes projecting into intercellular spaces. In the early static dominant follicle, most of the granulosa cells are polyhedral (others are elongated) and the intercellular spaces are wide. The granulosa cells contain abundant mitochondria, smooth and roughendoplasmic reticulum, and occasional lipid vacuoles (LV). The area enclosed in the box is magnified in the upper right corner to show that gap junctions (arrowheads) between the cells are frequent. An, antrum; BM, basement membrane; *, intercellular spaces; MG, membrana granulosa; GC, granulosa cells; TI, theca interna; Cp, capillary. While early histologic studies provide insight regarding the morphologic changes that occur during folliculogenesis and an overall impression of the time required, they do not provide an understanding of day-to-day dynamics and control of antral follicle development during the estrous cycle. By inference from Rajakoski’s review of the early literature,12 it appears that two schools of thought prevailed at the time (Figure 24.5): (i) follicular growth and regression is a continuous process and is independent of the phase of the reproductive cycle; or (ii) a reservoir of antral follicles remains in a resting stage, and at a particular time in each species one or several of these follicles begin to grow and reach maturity during the ensuing estrus. However, based on gross and histologic evaluation of ovaries obtained from cows slaughtered on known days of the estrous cycle (n = 1–3 cows/day), Rajakoski concluded that for antral follicles (>5 mm) two growth waves occurred during the estrous cycle. The first wave of growth was initiated on days 3 and 4 of the estrous cycle (estrus = day 1) and the second between days 12 and 14. His interpretation was that the follicles in each wave that did not ovulate regressed and became atretic. Figure 24.5 Theories of ovarian follicular dynamics during the estrous cycle in cattle, illustrated from one ovulation to the next (i.e., one interovulatory interval). Estrus is indicated by the solid bar along the x-axis and plasma LH concentrations by the dashed line. (a) The theory of continuous recruitment or “propitious moment” is that antral follicles grow and regress continuously throughout the interovulatory interval and the ovulatory follicle is the one that by happenstance is at the appropriate stage of development to respond to the preovulatory surge of LH. (b) The wave theory of follicle recruitment is that two or more cohorts (i.e., waves) of antral follicles are recruited (i.e., emerge) during the ovarian cycle, regardless of the presence of a corpus luteum (follicular phase or luteal phase). The dominant follicle that develops in the final wave of the interovulatory interval ovulates while preceding waves are anovulatory. Modified from Adams G, Singh J, Baerwald A. Large animal models for the study of ovarian follicular dynamics in women. Theriogenology 2012;78:1733–1748. Contrary to the conclusions of Rajakoski, interpretation of later histological studies supported the notion that follicular growth is continuous and independent of the phases of the cycle.13–15 Controversy regarding the wave theory of follicular dynamics was evident in later reviews in which the two-wave theory was refuted.16–18 Evidence was presented to support the concept that follicles are recruited continuously throughout the cycle and the follicle destined to ovulate was a result of coincidence of its stage of maturity (readiness) and the occurrence of the preovulatory gonadotropin surge, i.e., the “propitious moment” theory (Figure 24.5). However, with the introduction of ultrasonography in the late 1980s, the barrier to our understanding of the dynamic process of follicle development was suddenly removed. The technique of detecting and monitoring ovarian structures by transrectal ultrasonography was described initially in 1984.19 By comparing the results of ultrasonography with the results of slicing excised ovaries, the ultrasound technique was validated as a tool for detecting follicles greater than 2 mm in diameter and for monitoring the corpus luteum.20,21 Subsequent studies using ultrasonic imaging to monitor follicle populations in different size categories22 or to monitor individually identified follicles23–26 convincingly documented that follicular growth in cattle occurs in a wave-like fashion and that the majority of estrous cycles in cattle comprise two or three such waves. The method by which individual follicles may be monitored throughout one interovulatory interval by ultrasonography is illustrated in Figure 24.6. During daily examinations, a sketch of each ovary is made to record the number, diameter, and relative positions of follicles and the corpora lutea. Even though the ovaries are quite moveable at the end of the mesovarium, their position remains remarkably consistent from one examination to the next, allowing accurate “mapping” of ovarian structures over time. The results of ultrasound studies are consistent with those in which three waves of follicular growth were hypothesized27 based on the presence of one “estrogen-active” follicle during three different periods of the estrous cycle28,29 and increases in estradiol in blood from the utero-ovarian veins during approximately the same time periods.30 Figure 24.6 Mapping ovarian follicular and luteal dynamics in cattle by serial ultrasonography. During daily examinations (day 0 = ovulation), a sketch of each ovary is made to record the number, diameter, and relative positions of follicles and the corpus luteum. The data are used to identify the number of follicular waves during the interovulatory interval (two waves in this example), follicular wave emergence (days 0 and 10), the dominant follicle of each wave (growing, dark blue; regressing, pale blue), the preselection and subordinate follicles of each wave (black), the occurrence of ovulation (days 0 and 20), and the growth and regression of the corpus luteum (orange). Note that both ovaries contribute to a given follicular wave, and successive dominant follicles may be in either ovary (random). The size and number of ovarian structures in each sketch are tabulated under the respective follicle and corpus luteum columns. The high progesterone (luteal) phase is outlined in the yellow box. Follicular wave emergence in cattle is characterized by the sudden (within 2–3 days) growth of 8–41 small follicles that are initially detected by ultrasonography at a diameter of 3–4 mm (Figures 24.6 and 24.7).20,25,26,31,32 The growth rate is similar among follicles of the wave for about 2 days, after which one follicle is then selected to continue growth (dominant follicle) while the remainder become atretic and regress (subordinate follicles). In both two- and three-wave estrous cycles, emergence of the first follicular wave occurs consistently on the day of ovulation (day 0). Emergence of the second wave occurs on day 9 or 10 for two-wave cycles, and on day 8 or 9 for three-wave cycles. In three-wave cycles, a third wave emerges on day 15 or 16. Under the influence of progesterone (e.g., diestrus, pregnancy), dominant follicles of successive waves are anovulatory and undergo atresia.33 The dominant follicle present at the onset of luteolysis becomes the ovulatory follicle, and emergence of the next wave is delayed until the day of the ensuing ovulation. The corpus luteum begins to regress earlier in two-wave cycles (day 16) than in three-wave cycles (day 19), resulting in a correspondingly shorter estrous cycle (20 days vs. 23 days, respectively). Hence, the proverbial 21-day estrous cycle of cattle exists only as an average between two- and three-wave cycles. Figure 24.7 Follicular dynamics during two-wave (upper) and three-wave (lower) estrous cycles in cattle. Data from both ovaries are used to identify the dominant follicle of each wave (growing, dark blue; regressing, pale blue), the subordinate follicles of each wave (growing, black; regressing, gray), the occurrence of ovulation, and the growth and regression of the corpus luteum (dark yellow). A follicular wave in cattle is characterized by the sudden emergence (within 2–3 days) of a group of small follicles (n = 8–41) that grow at a similar rate for about 2–3 days, at which time one follicle is selected to continue growth (dominant follicle) while the rest become atretic and regress (subordinate follicles). The often referred to “21-day” estrous cycle of cattle exists only as an average between two-wave cycles (20 days) and three-wave cycles (22 days). The majority of bovine estrous cycles (>95%) are composed of either two or three follicular waves (Figure 24.7).34,35 Some have reported a preponderance (>80%) of either the two-wave or the three-wave pattern during an interovulatory interval, while others have reported a more even distribution. There appears to be no breed- or age-specific predilection for a given wave pattern in Bos taurus. However, an increase in the proportion of three-wave patterns has been associated with a low plane of nutrition36,37 and heat stress.38,39 In Bos indicus, no seasonal effect on wave pattern was detected,40 but the pattern was influenced by parity. The majority of Nelore heifers (65%) exhibited a three-wave pattern, whereas the majority of cows (83%) exhibited a two-wave pattern.41 Others have reported that up to 27% of estrous cycles in Bos indicus cows consist of four waves of follicular development, compared with only 7% in Bos indicus heifers (reviewed in Ref. 42). Predictive factors associated with a two- versus three-wave pattern may provide insight into mechanisms controlling the pattern, and have important implications for breeding management and the development of effective protocols for ovarian synchronization, superstimulation, and fixed-time artificial insemination. Pregnancy rates in cattle with two- versus three-wave patterns were compared43–45 based on the notion that the preovulatory follicle in the two-wave pattern grows for a longer period31 and may therefore contain a relatively aged oocyte. However, results have been contradictory; pregnancy rates did not differ between two- versus three-wave cycles in some studies,43,45 whereas a lower pregnancy rate following ovulation at the end of the two-wave pattern was reported in another study.44 In a more recent study involving ultrasonographic data from 91 interovulatory intervals,35 two- and three-wave patterns of follicular development were compared to determine the repeatability and predictive characteristics of a given wave pattern. Two-wave cycles were 3 days shorter than three-wave cycles (19.8 ± 0.2 vs. 22.5 ± 0.3; P < 0.01). The majority of cycles of 21 days or less were of the two-wave pattern (88%; P < 0.05), while the majority of cycles 22 days or longer were of the three-wave pattern (78%; P < 0.05). The proportion of successive cycles in which the pattern remained the same (i.e., repeatability) was more than twofold greater than the proportion of cycles that changed patterns (70% vs. 30%; P < 0.01). The repeatability of wave pattern, and the proportion of two- versus three-wave patterns within the herd were not affected by the season of the year; in other words not affected by pasture-based (spring and summer) versus non-pasture-based (fall and winter) seasons. Interestingly, the strongest correlate to the number of waves in the interovulatory interval was the duration of follicular dominance of Wave 1. The duration of dominance of the Wave 1 dominant follicle was 3 days longer, and the onset of regression was later in two-wave patterns than in three-wave patterns (P < 0.01). Dominance of Wave 1 was associated with a subsequent delay in the attainment of maximum diameter by the dominant follicle of Wave 2, as well as early onset of luteolysis (Figure 24.7). Results supported the hypothesis that factors which influence the development of the dominant follicle of Wave 1 are responsible for regulating the wave pattern. The two ovaries act as a single unit – one ovary does not have follicular waves independent of the other. The ovaries respond in unison to factors regulating follicular wave dynamics, hence a follicular wave is the result of multiple follicle growth in both ovaries (Figures 24.6 and 24.7). In a critical study of the intraovarian relationships among follicles and the corpus luteum, Ginther et al.46 concluded that the dominant follicle suppresses subordinates and new wave emergence via systemic rather than local channels. The presence of a dominant follicle in one ovary had no effect that could not be seen equally in the other ovary. Hence, no intraovarian relationships were found in the location of successive dominant follicles during the estrous cycle; in other words, the side of anovulatory and ovulatory dominant follicles was random. These observations are consistent with the findings of early studies on the effects of unilateral ovariectomy. Removal of the ovary with the largest follicle resulted in an increase in follicle development in the remaining ovary, but removal of the ovary with’out the largest follicle resulted in no such compensatory effect (cited in Ref. 46). While intrafollicular (autocrine and paracrine) factors are important for growth, health, and demise of an individual follicle, there is no convincing in vivo documentation of one follicle affecting the health/regression status of its neighbors directly. Asymmetry in follicle dynamics in the left and right ovaries has been used to elucidate local versus systemic mechanisms of control of ovarian function, and some have reported greater follicular activity and proportion of ovulations in the right ovary in cattle (~60%),12,22 whereas others report no such differences.26,31 Ovarian follicular asymmetry has also been attributed to the presence of a corpus luteum. A positive intraovarian effect of the corpus luteum on the development of small antral follicles (≤3 mm) has been documented in sheep47 and cattle,21 but the positive effect did not extend to large dominant follicles.21,46 Conversely, the corpus luteum of pregnancy has been associated with a negative intraovarian effect on the dominant follicle.46,48 Dominant follicles of successive waves were more frequently (75–80%) found in the ovary contralateral to the corpus luteum during pregnancy. The cause of the negative local association between the corpus luteum and the follicles is unknown but it may be directly related to the conceptus rather than to the corpus luteum. Support for this notion is found in the observation that there were no ovarian asymmetries during the first two follicular waves in pregnant cattle or in 10 successive follicular waves in unmated progesterone-treated heifers.33 The latter observation, however, is confounded by the fact that exogenous progesterone did not forestall luteolysis in treated heifers; luteal regression occurred at the expected time for a nonpregnant animal regardless of progesterone treatment. However, an experimental design involving hysterectomy during mid-diestrus permitted direct comparison of the effects of a persistent corpus luteum due to hysterectomy or pregnancy.49 Results demonstrated that unilateral attenuation of follicle development was due to the conceptus or the ipsilateral gravid uterine horn, and not the corpus luteum. Results of early studies of follicle dynamics gave rise to the hypothesis that the dominant follicle suppresses the growth of the subordinates in the existing wave, and suppresses the emergence of the next follicular wave.31,32 Support for this hypothesis was provided in a series of studies involving systemic treatment with the proteinaceous fraction of follicular fluid and by electrocautery of the dominant follicle.50–52 The applied implications of these findings were immediate and far-reaching, and marked a new era for ovarian synchronization and superstimulation in cattle.53–55 Emergence of a follicular wave and selection of the dominant follicle are temporally and causally associated with a rise and fall in circulating concentrations of follicle-stimulating hormone (FSH)51 (Figure 24.8). Emergence of a follicular wave is preceded by a surge in plasma FSH concentrations in both spontaneous waves and induced waves.51,56 Surges in FSH preceding each wave are of similar magnitude: mid-cycle surges are similar in amplitude and breadth as those of the preovulatory gonadotrophin surge.51,56 Follicular products, especially those from the dominant follicle, are responsible for suppressing FSH release and therefore the emergence of the next follicular wave57 (Figure 24.9). A direct relationship between the number of follicles emerging in response to the FSH surge and the subsequent degree of FSH suppression58–60 documents that all follicles of the new wave contribute to FSH suppression. Figure 24.8 Temporal and causal relationship between surges in circulating concentrations of FSH and follicular wave emergence in cattle. Peak concentrations of FSH occur on the day before follicular wave emergence (at 4 mm) and decline to nadir by 3 days after wave emergence at the time the dominant follicle can be distinguished from the subordinates. Development of a dominant or subordinate follicle may be divided into a growing phase (best characterized by a quadratic equation), a static phase, and a regressing phase (best characterized by simple linear equations). Data from Adams et al.51, 53 Figure 24.9 Hormonal interplay controlling follicular wave dynamics during a two-wave interovulatory interval in cattle. Dominant and subordinate follicles of each wave are indicated as gray-shaded circles, and the corpus luteum is the gray-shaded irregular shape drawn above the follicles. A surge in circulating concentration of FSH precedes emergence of each wave. A surge in circulating concentration of LH precedes ovulation. The LH surge is preceded and succeeded by a period of high LH pulse frequency as a result of low circulating concentrations of progesterone (i.e., period of luteolysis and luteogenesis, respectively). The number of follicles ≥4 mm fluctuates in a wave-like fashion in response to surges of FSH. Products of the follicles of each wave (primarily inhibin and estradiol) feed back on the hypothalamus and pituitary to suppress FSH. Concentrations of FSH decline at the time that selection of divergence in growth rate of the dominant versus subordinate follicles, and follicle numbers decline. The dominant follicle of each wave is the primary source of estradiol, and production is twice as high in the ovulatory versus anovulatory dominant follicle. Upon the demise or ovulation of the dominant follicle, the follicular products responsible for suppressing FSH drop, and circulating concentrations of FSH are again allowed to surge resulting in new wave emergence. Adapted from Adams G, Jaiswal R, Singh J, Malhi P. Progress in understanding ovarian follicular dynamics in cattle. Theriogenology 2008;69:72–80. Estradiol and inhibin-A and -B are the principal follicular products responsible for suppressing FSH.57,61–65 Inhibin-A is produced by all the small growing follicles of the wave and appears to be the most important suppressor of FSH during the first 2 days of wave emergence; estradiol secreted from the dominant follicle is the most important FSH suppressor thereafter.66–69 The nadir in FSH is reached 4 days after wave emergence and levels remain low for next 2–3 days. At the end of the period of dominance (i.e., at ovulation, or the mid-static phase of an anovulatory dominant follicle), circulating concentrations of FSH begin to rise over the next 2 days and peak about 12–24 hours before emergence of the next follicular wave, when the future dominant follicle is 4–5 mm in diameter. If an existing dominant follicle is removed (i.e., follicular ablation), a surge in FSH begins within the next 12 hours and results in emergence of a new follicular wave within the next 24 hours.52,70 Interestingly, FSH only rises again when inhibin-A concentrations reach nadir, despite the fact that estradiol declines 2 days earlier.63 An inverse relationship between circulating progesterone concentrations and dominant follicle growth was suggested by the observations that (i) in three-wave estrous cycles, the maximum diameter of the dominant anovulatory follicle was greater for Wave 1 (under minimal luteal dominance during its growing phase) than for Wave 2 (under maximum luteal dominance); (ii) the maximum diameter attained by the dominant anovulatory follicle was greater for Wave 1 than for all subsequent waves in pregnant and nonmated progesterone-treated heifers; and (iii) high concentrations of progesterone (endogenous plus exogenous) resulted in a smaller maximum diameter of the dominant follicle of Wave 2 compared with subsequent waves (exogenous progesterone only) in nonmated progesterone-treated heifers (reviewed in Ref. 71). A cause-and-effect relationship was established in studies in which progesterone was shown to suppress the dominant follicle during its growing phase in a dose-dependent manner71 through the negative feedback effect of progesterone on luteinizing hormone (LH) pulsatility.72–74 The suppressive effects on the dominant follicle were not mediated by decreased circulating FSH since progesterone treatment did not suppress FSH.71 High plasma progesterone during follicular growth resulted in a smaller shorter-lived dominant follicle, an earlier surge in FSH preceding the next follicular wave, and hastened emergence of the next follicular wave (Figure 24.10). Conversely, low concentrations of progesterone resulted in an oversized persistent dominant follicle, a delayed surge in FSH, and delayed emergence of the next wave.71 The oversized dominant follicle in the low-dose situation would have been considered a pathological follicular cyst because of its size and persistence for more than 10 days in the absence of a corpus luteum.75,76 Low-level progesterone exposure (and therefore less inhibition of LH), at the time the dominant follicle normally stops growing, appears to be a fundamental component in the etiology of ovarian follicular cysts.71 Figure 24.10 Suppressive effect of progesterone on the growth of the dominant follicle in cows. Exogenous progesterone administered in low (30 mg/day), medium (150 mg/day), and high (300 mg/day) doses for 14 days resulted in progressively greater suppression of the growth of the dominant follicle and progressively shorter interwave intervals (arrowheads on x-axis). Maximum plasma progesterone concentrations were 2, 9, and 17 ng/mL in the low-, medium- and high-dose groups, respectively. The suppressive effect on the growing phase of the dominant follicle is attributed to progressively more suppression on LH pulse frequency. Adapted from Adams G, Matteri R, Ginther O. The effect of progesterone on growth of ovarian follicles, emergence of follicular waves and circulating FSH in heifers. J Reprod Fertil 1992;96:627–640. In monovular species, the term “selection” refers to a process by which a single follicle becomes functionally and morphologically dominant over other follicles of a wave. In cattle, the mechanism of selection of the dominant follicle is based on relative reliance of follicles within a wave on FSH, and their relative responsiveness to LH. The transient rise in FSH permits sufficient follicular growth so that some (not all) follicles acquire LH responsiveness. The ability to respond to LH imbues the follicle with the ability to survive without FSH. It remains unclear precisely when the process begins, but selection of the dominant follicle is associated with declining levels of FSH in circulation during the first 3 days of the wave.51,77 Small follicles of the emerging wave (<6 mm) are dependent on elevated circulating concentrations of FSH for continued development. In the face of the post-surge decline in FSH, the growth of most of the follicles of a wave stops and they begin to regress within 2–5 days of emergence. Conversely, the follicle destined to become dominant can maintain cell proliferation and estradiol production despite declining FSH concentrations, an ability that appears to be brought about by maintaining high FSH receptor mRNA expression and FSH-binding affinity.28,29,78,79 Divergence in the growth profiles (defined as a significant difference in diameter) of the largest and second largest follicle is a manifestation of the selection process and is apparent by 2.5 days after wave emergence (Figures 24.8 and 24.9).77 Similarly, follicle “deviation” (defined as a difference in growth rate between the dominant and subordinate follicles) is manifest at 2.5 days after wave emergence, when the future dominant follicle reaches a mean diameter of 8.5 mm in Holstein heifers (reviewed in Ref. 80). Continued development of the dominant follicle, beyond the 8-mm threshold (point at which divergence in the growth rate of the largest two follicles becomes evident), is associated with a transition from FSH to LH dependence.66,68,81 Granulosa cells from the dominant follicle acquire an enhanced ability to bind LH compared with that of the subordinates, and have greater LH receptor mRNA expression.29,78,79,82,83 Although a slight increase in LH receptor mRNA expression was detected in granulosa cells from the largest follicle a few hours before the onset of deviation,84 it is not yet clear whether the observed increase in LH receptor expression in the dominant follicle is the cause of dominance or a consequence of the selection process. Perhaps the increased LH receptor expression is merely a reflection of a follicle with a developmental advantage before such an advantage becomes ultrasonographically apparent. In this regard, results of a critical study of the development of small follicles support the hypothesis that the follicle destined to become dominant has a size advantage over the others in the wave from its earliest detection at 1 mm (Figure 24.11) (see section Small antral follicles). Figure 24.11 Growth (mean ± SEM) of dominant and subordinate follicles in cattle (n = 9) relative to (a) wave emergence (defined as the day on which the dominant follicle was detected at 1 or 4 mm in diameter; double x-axis), and (b) the peak in plasma FSH concentrations (mean ± SEM). (a) The future dominant follicle had a size advantage over all other follicles of the wave from its earliest detection at 1 mm (much earlier than previously documented). Successive slowing of the growth rate of the third, second, and first largest follicles of a wave during the decline in the FSH surge suggests that the selection mechanism involves sequential suppression of progressively larger follicles over a period of 72 hours (i.e., selection hierarchy). “Deviation” is defined as the first day on which the growth rate of the largest and second largest follicles differed (P ≤ 0.05). (b) Plasma FSH concentrations were positively correlated (P = 0.01) with follicle diameter from the time of follicle detection at 1 mm to the time at which FSH concentrations peaked. Transient elevations in plasma FSH concentration were followed within 6 hours by an increase in the growth rate of 1–3 mm follicles. For FSH, values with no common superscript (a, b) are different (P ≤ 0.05). Adapted from Jaiswal R, Singh J, Adams G. Developmental pattern of small antral follicles in the bovine ovary. Biol Reprod 2004;71:1244–1251.
Ovarian Follicular and Luteal Dynamics in Cattle
Introduction
Ovarian development and follicle assembly
Folliculogenesis and oogenesis
Follicle stage
Follicle type
FSH/LH receptors
Granulosa cell layersa
Granulosa cells per sectiona
Follicle diameter (mm)
Oocyte diameter (µm)
Zona pellucida
Theca interna
Primordial
1
1
<10 flattened
<0.04
30
Absent
Absent
Transitory
1a
Entered growing pool and surrounded by a mixture of flattened and cuboidal cells
Primary
2
FSHr form (granulosa)
1–1.5
10–40b cuboidal
0.04–0.08
31
Absent
Absent
Secondary
3 (small preantral)
2–3
41–100
0.08–0.13
50
Begins to form
Begins to form
4 (large preantral)
LHr form (theca)
4–6
101–250c
0.13–0.25
69
Partially formed
Partially formed
5 (small antral)
>6
>250
0.25–0.5
93
Tertiary (antral)
Large antral Graafian
LHr in granulosa of dominant follicle
40 × 106
10–15
132
Fully formed
Follicle size range (mm)
Granulosa cell generation
Time in each size class
Percent atretic
Mitotic activity
Hours
Days
0.13–0.28
6
365
15.1
1.6
0.29–0.67
8
285
11.9
6.6
0.68–1.52
10
100
4.2
40.5
Maximum
1.53–3.67
11
83
3.5
30
3.68–8.57
12
186
7.8
67.4
>8.57
13
60
Total
1019
42.5
Antral follicle dynamics
Follicular waves during the estrous cycle
Two-wave versus three-wave patterns
Two ovaries, one wave
Hormonal interplay controlling follicular wave dynamics
Selection of the dominant follicle
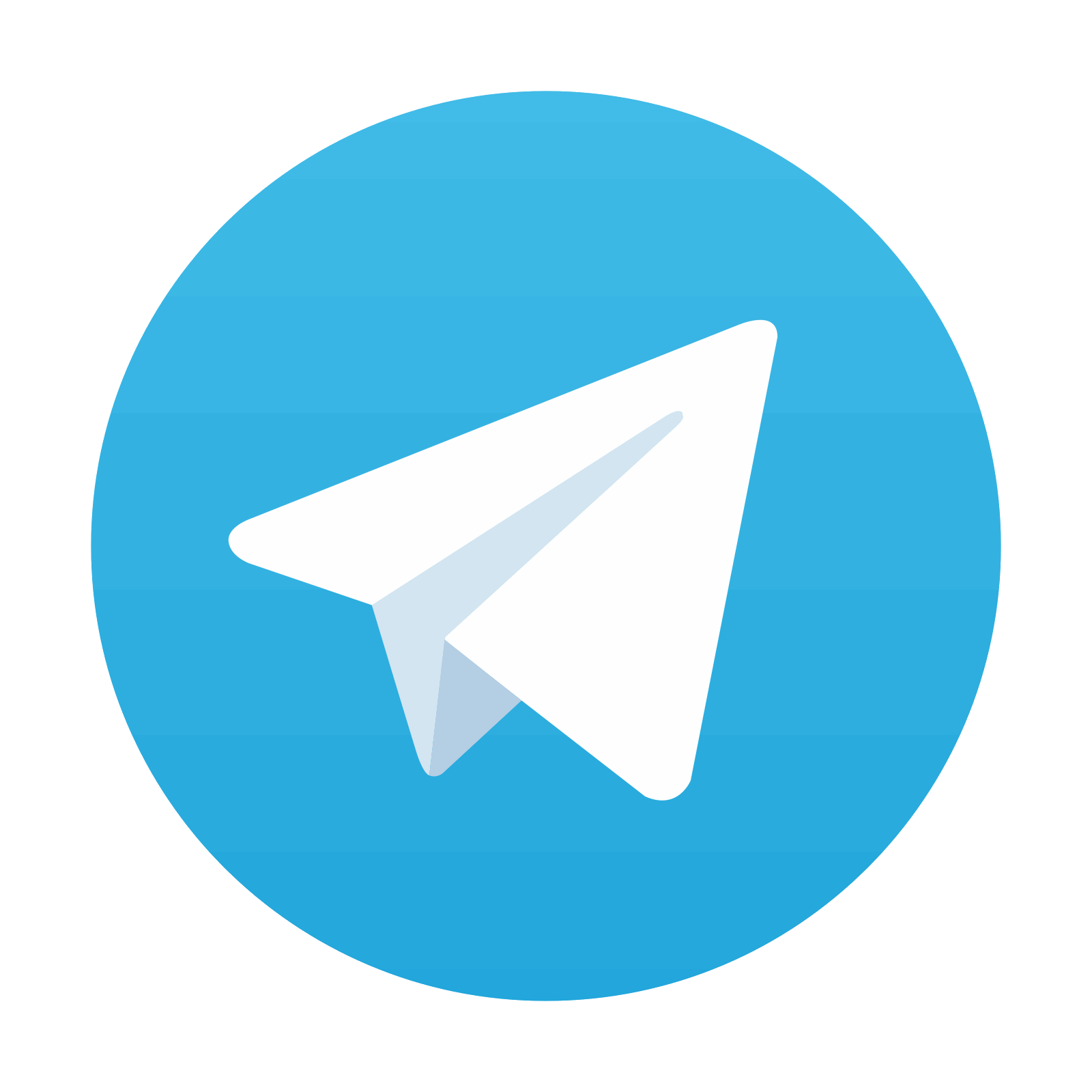
Stay updated, free articles. Join our Telegram channel

Full access? Get Clinical Tree
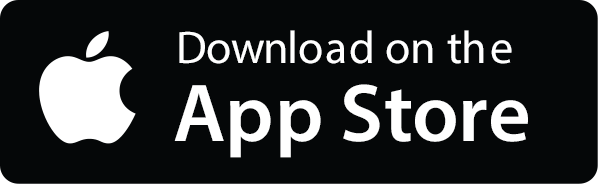
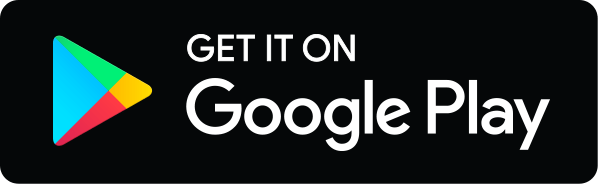