Vaidehi Paranjape and Stephen Cital Opioids form an integral part of an anesthesia regime to provide perioperative analgesia, sedation, and anesthetic‐sparing effects in small animals. However, their significant side‐effects and restricted availability due to the ongoing abuse crisis in humans warrant their limited use. Hence, opioid alternative techniques are increasingly being used to enhance the anesthetic and postanesthetic experience in animals. These include nonopioid analgesic infusions, oral gabapentanoids, psychotropic drugs, cannabinoids, antiinflammatories, nonpharmacologic pain modalities and robust use of local‐regional anesthetic techniques, which are briefly reviewed in this chapter. Ketamine (N‐methyl‐D‐aspartate receptor antagonist), dexmedetomidine (alpha‐2 adrenoreceptor agonist), and lidocaine (sodium channel blocker) are routinely used as constant‐rate infusions (CRIs) to provide analgesia in the perioperative period. With basic understanding of the major pain pathways, these drugs are used as either primary analgesics or adjunctive analgesics to provide multimodal analgesia. The NMDA receptor is a ligand‐gated, voltage‐dependent ion channel, widely distributed in the CNS and primary sensory neurons. It serves as a binding site for glutamate, which is released with noxious peripheral stimuli. It serves as a noncompetitive antagonist at the NMDA receptor and is used at subanesthetic doses of 0.12–1.2 mg/kg/h as an adjunctive to other analgesics. These doses are less likely to produce dissociative and cardiostimulatory effects. Activation of NMDA receptor leads to the development of cancer, chronic, neuropathic and wind‐up pain, central sensitization and hyperalgesia [1]. It may be more effective for somatic pain than visceral pain [2]. Additionally, it exhibits opioid‐sparing effects and counteracts opioid‐induced hyperalgesia. Authors recommend its cautious use in cats with progressive renal disease and hypertrophic cardiomyopathy. Alpha‐2 adrenoceptors are a class of G protein‐coupled receptors that serve as a primary site for norepinephrine and epinephrine. They are found peripherally and at spinal and supraspinal sites within the CNS. They induce antinociception by: Dexmedetomidine is the most selective drug for alpha‐2 adrenoreceptors, with a binding affinity for alpha‐2:alpha‐1 receptors reported as 1620:1. It is a pure S‐enantiomer of the racemic medetomidine and is twice as potent as medetomidine. Micro‐bolus doses of 0.0005–0.002 mg/kg can be given intraoperatively as needed, or 0.0005–0.002 mg/kg/h administered as a CRI for sedation, anxiolytic effect, anesthetic adjunct, inhalant‐sparing effect, supplemental somatic and visceral analgesia, and muscle relaxation [4]. Cardiorespiratory monitoring is essential when it is used postoperatively. If excessive sedation or significant cardiovascular effects are extended into the recovery period, reversal with atipamezole IM is considered. Side‐effects requiring possible intervention include bradycardia, atrioventricular blocks, respiratory depression, nausea, vomiting, and ileus. This drug should be avoided in patients with moderate‐severe cardiac disease, cardiac arrhythmias, preexisting hypertension, and hypovolemia. The systemic analgesic action is thought to be due to lidocaine’s interaction with Na+, Ca2+, and K+ channels and the NMDA receptor [5]. It is the only local anesthetic that is administered intravenously to provide analgesia and inhalant‐sparing effects. It possesses antiinflammatory effects and free radical scavenging properties that contribute to management of inflammatory pain. In dogs, IV loading dose of 1–2 mg/kg followed by infusion rates of 3–6 mg/kg/h are commonly used for intraoperative analgesia. Lower infusion rates of 0.6–1.5 mg/kg/h are used postoperatively. In cats, studies show that the doses required to achieve inhalant‐sparing effects can cause greater cardiovascular depression compared to an equipotent dose of isoflurane alone [6]. Gabapentin was traditionally used as an anticonvulsant, but its benefits include anxiolysis, analgesia, antiallodynia, and antihyperalgesia. Pregabalin is structurally similar to gabapentin but has higher oral bioavailability and a longer half‐life. However, at the time of writing, it is expensive. This makes gabapentin a more popular gabapentanoid for use during chronic pain; the authors encourage the reader to evaluate the prices from your distributors. Gabapentin is a structural analogue of gamma‐aminobutyric acid (GABA) but does not interact with GABA receptors to produce analgesia. It binds to the α2δ subunit on the voltage‐dependent calcium channels located in neurons of the peripheral and CNS. This reduces calcium influx into neurons and inhibits release of excitatory neurotransmitters like glutamate, substance P and norepinephrine that are actively involved in pain pathways. Neuropathic pain is a result of a lesion or disease affecting the somatosensory system and is generally chronic and challenging to treat. With prolonged use, psychotropic drugs possibly relieve neuropathic pain by (1) recruitment of noradrenergic descending pathways, (2) peripheral recruitment of noradrenaline from sympathetic fibers sprouting into dorsal root ganglia, and (3) action via adrenergic receptors. Tricyclic antidepressants (TCAs, e.g., amitriptyline), selective serotonin reuptake inhibitors (SSRIs, e.g., fluoxetine), NMDA antagonists (e.g. amantadine), and atypical antidepressants (e.g., trazodone) are often used as adjunctive therapy for managing chronic pain states [16–18]. Psychotropic drugs are useful in clinical scenarios manifesting neuropathic pain such as Chiari‐like malformation/syringomyelia, radiculopathy caused by chronic cervical or lumbosacral disc disease, diabetic or other polyneuropathies, spinal cord injury caused by intervertebral disc extrusion, chronic osteoarthritis, and musculoskeletal pain. Drug interactions can occur when different serotonin‐enhancing agents are administered together (e.g., fluoxetine with tramadol or fluoxetine with selegiline) leading to “serotonin syndrome” (see Chapter 5, p. 154) [20]. It is vital that these interactions are well known to the prescribing clinician and owners. Other common adverse effects of psychotropic drugs include lethargy, sedation, vomiting, diarrhea, panting, hyperactivity, ataxia, increased anxiety, increased appetite, shaking, restlessness, and/or agitation. Arrhythmias, tachycardia, and orthostatic hypotension can be seen with high doses of TCAs. The ECS is the largest G‐protein‐coupled receptor system with neuromodulatory functions. It is now known as one of the key endogenous systems regulating pain sensation, with modulatory actions at all stages of pain‐processing pathways. Its effects are mediated by the interaction of cannabinoid receptors (CB1, CB2), endogenous ligands, and synthesizing and hydrolyzing enzymes [21,22]. CB1 receptors are mainly present in the brain and spinal cord and are responsible for centrally mediated analgesia as well as adverse central effects. They inhibit acetylcholine, L‐glutamate, GABA, norepinephrine, dopamine, and serotonin. CB2 receptors are found in the hematopoietic and immune cells, including microglial cells. These are possibly involved in immune regulation and antiinflammatory effects [23]. Phytocannabinoids are molecules produced by the Cannabis sativa plant which can act on the ECS receptors. Hemp and marijuana are both varieties of the Cannabis genus. Hemp is a variety of cannabis that produces large amounts of cannabidiolic acid compared to the amount of tetrahydrocannabinolic acid produced. The inverse is true for marijuana. After harvesting and drying of the plant material, particularly the flowering tops of female plants, the acidic forms of these two prominent phytocannabinoids become decarboxylated into their mainstream personalities – cannabidiol (CBD) and tetrahydrocannabinol (THC). Tetrahydrocannabinol is a major intoxicating compound, associated with unpleasant clinical presentation in animals that consume this molecule. Animals may experience increased anxiety with mild‐moderate doses of THC. Tolerance can be built up to THC as it is the only phytocannabinoid to sit in the orthosteric binding site on the CB1 receptor. The stronger affinity of THC compared to endogenous ligands when dosed chronically can downregulate endocannabinoid production which may have adverse physiologic consequences. Also, because of the risk and particular sensitivity of THC in companion animals, it may be best to avoid THC‐dominant products and utilize products derived from hemp. In addition, newer research has shown that THC alone is not a good analgesic for chronic [24] pain states. On the other hand, CBD may possess analgesic effects, best described in chronic or neuropathic pain states. Both of these phytochemical compounds, among the hundreds of other phytocannabinoids, have predominantly allosteric binding to ECS receptors and affinity/dimerizing effects on several receptors systems related to pain signaling which include the transient receptor potential vanilloid (TRPV), NMDA, glycine, G‐protein‐coupled (GPR55), GABA, and serotonin (5‐HT) receptors [25]. Well‐controlled studies in companion animals on the efficacy of these compounds are increasing. Three pharmacokinetic studies in dogs found that doses of 2 mg/kg create adequate serum concentrations, related to therapeutic benefits extrapolated from other lab animals and human subjects. Three long‐term studies (12, 39, and 56 weeks) have been performed in dogs with doses from 2 mg/kg up to 100 mg/kg. Mild‐moderate elevations of liver enzymes were noted in the higher dose long‐term studies [26,27]. In cats, only two studies have been published, elucidating that a 2 mg/kg dose may need to be given more than twice a day to maintain suspected therapeutic serum concentrations [28,29]. Efficacy of hemp CBD products has also been evaluated for management of osteoarthritic pain in dogs [30–32]. These studies had successful outcomes, particularly for pets on traditional pharmaceuticals such as NSAIDs and gabapentin. Owners were also able to eliminate the traditional pharmaceuticals out of the analgesia regime. Acute pain studies are still under way in companion animals; currently, only anecdote abounds [33]. Dosing of CBD‐dominant products will depend on the other phytocannabinoids and terpenes present. Terpenes are aromatic molecules also produced by the Cannabis plant that have their own set of therapeutic potentials. Doses from the two canine studies found oral doses of a full/complete spectrum cannabinoid product of 2 mg/kg twice a day to be safe and effective. The authors would suggest a dosing range of 0.5–2 mg/kg, or higher, depending on clinical response. Clinicians prescribing cannabinoid products must be fully aware of the primary source (hemp vs marijuana), the concentration and the phytocannabinoid/terpene profile of the product verified by a certificate of analysis (COA). There are a few different types of phytocannabinoid products on the market being sold as animal supplements [34]. Clinicians may notice increased lethargy in patients that are on concurrent oral narcotics or gabapentanoids. Doses of the cannabinoid product or pharmaceutical are adjusted to minimize this effect. Other notable drug interactions occur with benzodiazepines, where patients may seem uncoordinated or ataxic. Long‐term pharmacological management of pain in small animals includes use of nonsteroidal antiinflammatory drugs (NSAIDs) or corticosteroids. Nonsteroidal drugs possess antipyretic, antiinflammatory, and analgesic effects. Significant analgesic and antiinflammatory clinical effects are due to the inhibition of the COX enzyme isoforms and central pain transmission. They are widely used to control mild‐moderate pain during the perioperative period as well as for chronic pain states. On the other hand, corticosteroids inhibit the production of arachidonic acid, which can stop the inflammation and stop the production of prostaglandins, similar to NSAIDs. They do not have a direct effect on nociception and are not primary analgesics. However, they are beneficial in controlling pain mediated via inflammatory processes as seen in musculoskeletal and joint disorders. More information on this class of drugs is available in the drug formulary (Section II). Acupuncture and physical rehabilitation are fundamental in the multimodal management of pain, especially of a chronic nature. These aid in balancing analgesic regimes, strengthening human–pet relationships, and increasing the quality of patient care and comfort. Acupuncture involves placement of percutaneous needles in specific anatomic locations on the body, like nerve bifurcations or neurovascular structures in tissues, in order to stimulate an endogenous response facilitating analgesia, healing, and immunomodulation. It also improves blood flow, inhibits inflammation, reduces muscle tension, resets proprioceptive mechanisms and posture, and affects autonomic nervous system. Stimulation of A‐β, A‐δ and C nociceptive fibers prevents pain transmission via local spinal inhibition of nociception, spinal release of endogenous opioids, and activation of inhibitory interneurons and descending inhibitory pain pathways. The bioactive acupuncture points lie along “meridians” that serve as an energetic distribution network following specific peripheral nerve pathways targeted for preventing nociception. Stimulation of acupuncture points in animals is manifested by a response to needle placement followed by sudden relaxation. Acupuncture is a complex intervention requiring additional training. Clinical scenarios for its use include spinal cord injury/disease [35], wounds, chronic pain, osteoarthritis [36], visceral pain, and myofascial trigger point pain [37]. Physical rehabilitation involves objective assessment, diagnosis, and treatment of musculoskeletal and neurologic impairments during acute, subacute, and chronic pain states. The assessment utilizes careful evaluation of posture, gait, function, strength, muscle extensibility, passive range of motion, and joints to create a problem list and develop an assessment from which targeted treatment modalities are developed. These modalities provide pain relief, promote soft tissue healing, improve muscle extensibility, and facilitate muscle strengthening. Some of the commonly used physical therapy modalities are described below. Application of heat to tissues increases nerve conduction velocity and blood flow, facilitating healing, muscle relaxation, and resolution of local ischemia. Heat activates cutaneous thermal receptors that inhibit pain transmission. Heat can be applied with hot packs or warm baths for 15–20 min at a time. It is avoided in acute stages of tissue injury. Cooling results in vasoconstriction and a reduction in tissue metabolism and oxygen requirement, sensory and motor nerve conduction velocity, edema, and muscle spasm [37]. It is used in the acute inflammatory state. Ice packs should not be placed directly over the skin and their application should not exceed 10 min at a time. Portable machines, based on active compression and cold exchange loop technology, facilitate icing of limbs with tailored protocols, and may be quite useful to practices which extensively treat orthopedic pain [39,40]. Low‐level laser therapy is light energy used to stimulate healing, provide pain relief, and facilitate the reorganization of injured tissues. The range of therapeutic window for this therapy is 600–1000 nm. It aims at imparting analgesia within the target tissue, and causes cartilage stimulation, fibroblast production, enhancement of immune cells to combat pathogens, acceleration of collagen synthesis, and increasing vascularity of the healing tissue [41]. It is used to reduce inflammation and treat musculoskeletal, neuropathic, and osteoarthritic pain [42–45]. Studies are conflicting regarding the benefits of this therapy. Electrotherapy targets sensory and motor nerve fibers, causing nerve cell excitation and changes in cell membrane permeability. This enables skeletal muscle and smooth muscle contraction, enhancing muscle‐pumping action and improving joint mobility and circulatory and lymphatic drainage [46]. TENS decreases pain and inflammation by providing a low‐level electrical current which disrupts the normal pain perception pathways. The pulse rate and width are adjusted to deliver the desired effect [47]. This is production of high‐pressure sound waves emitted at high velocity by a generator that converts electrical energy into mechanical energy. The acoustic waves are delivered either in a focused manner, with greater penetration into the deeper tissues, or in a radial manner, affecting a wider area. Its benefits include angiogenesis, increase in collagen synthesis, increase in bone remodeling, and decrease in inflammatory mediators. It is incorporated in analgesic protocols for various musculoskeletal and joint disorders [48–50]. These therapeutic novelties increase connective tissue extensibility, vascular supply, and lymphatic drainage, and improve joint range of motion. Active and passive exercises are recommended for treatment of chronic pain associated with osteoarthritis. Reduced activity and deconditioning cause decreased muscle mass and strength, loss of endurance, increased joint stiffness, and loss of cardiovascular fitness. Reduction of pain and disability is achieved through improvement of muscle strength, stability of joints, and range of motion (ROM). Passive ROM exercises are used to enhance analgesia in inflamed joints and assist tissue stretching. Active ROM exercises are required to prevent muscle atrophy, increase strength and endurance, and enhance circulation [51]. Glucosamine hydrochloride and chondroitin sulfate are commonly recommended natural health products for treating osteoarthritis in small animals. Glucosamine regulates the synthesis of collagen in cartilage and possesses mild antiinflammatory effects. Chondroitin sulfate inhibits destructive enzymes in joint fluid and cartilage [52]. Other novel therapies include PRP and HA, which are gaining momentum for their beneficial effects in canine osteoarthritis, as evidenced both clinically and histologically [53,54]. Both therapies are accessible and cost‐effective for veterinary practitioners using commercially available products and kits [55]. Other nonpharmacologic techniques used in adjunctive management of acute and chronic pain include pulsating electromagnetic fields (PEMF), therapeutic ultrasound, neuromuscular electrical stimulation (NMES), myofascial release and trigger point therapy, nutraceuticals, and palliative radiotherapy. Local anesthetics consist of a lipophilic benzene ring (confers liposolubility) and a hydrophilic amine group (confers hydrosolubility). These two structures are linked together with an ester or amide linkage. Drugs with ester linkage include cocaine, procaine, tetracaine, and benzocaine that undergo hydrolysis by pseudocholinesterase in the blood and require minimal liver metabolism. Drugs with amide linkage include lidocaine, mepivacaine, bupivacaine, and ropivacaine which undergo extensive oxidative metabolism in the liver via cytochrome P450. Hepatic blood flow and hepatic function determine the clearance of these drugs. Hence, they are used cautiously in patients with liver disease. More information regarding the most commonly used LAs is provided in Table 7.1. Table 7.1 Commonly used local anesthetics and their toxic doses. Source: Adapted from [56]. Note: It is important to bear in mind the sliding scale of toxicity of these agents. In humans, the first known side‐effect of accumulating local anesthetic is tinnitus, or ringing in the ears. As this would be difficult to identify in companion animal species, veterinarians often see nausea and vomiting, which can progress to seizures (CNS toxicity) and then cardiovascular collapse. Suggested doses are given with the intent to reduce all complications, although this may vary by patient. CNS, central nervous system; IV, intravenous. Local anesthetics bind to sodium channels present in the peripheral neurons during their inactive state. This prevents subsequent channel activation and inhibition of sodium permeability that occurs with membrane depolarization. As the threshold level for the action potential is not attained, suppression of nerve signal transmission occurs between neurons. Local anesthetics with larger molecular weights, greater lipid solubility, and higher protein binding (e.g., bupivacaine) have a longer duration of action. The onset of action is related to the lipid solubility of the drug. The rate at which a LA diffuses into a nerve is determined by its concentration. The higher the concentration, the more rapid the onset of the block. Because of the lack of evidence showing a consistent advantage of mixing LAs, the authors prefer to simply select a single agent based on its desired and predictable characteristics [57]. Epinephrine may be used with LAs to cause vasoconstriction and therefore prolong the duration of action of LAs and intensity of the blockade. It also reduces the systemic absorption of LAs and decreases the probability of systemic toxicity. However, caution is advised if LA with epinephrine is used on extremities, as ischemia of the tissue may result. Preservative‐free buprenorphine (0.004 mg/kg) with bupivacaine injected into the epidural space provided up to 24 h analgesia in dogs undergoing stifle arthroplasty [56]. It is also used for perineural injections in order to extend the analgesic duration of LAs. Alpha‐2 agonists like dexmedetomidine when added to LAs enhance analgesia via hyperpolarization of C fibers during neuraxial anesthesia and peripheral nerve blocks. These drugs can produce analgesia through supraspinal and spinal mechanisms (via adrenergic receptors) and have inhibitory effects on conduction of nerve impulses. A dose of 0.1 μg/kg added to LA in femoral nerve blocks effectively prolongs analgesia for 24 h [56]. The same dose is also used for perineural injections. Ketamine produces localized hypoalgesia due to its LA‐like effects, which may be through its ability to inhibit neuronal sodium channels. Apart from causing NMDA blockade‐mediated analgesia in the CNS and spinal cord receptors, ketamine also interacts with opioid and monoaminergic receptors. Thoracic epidural administration of lidocaine with ketamine provides longer duration of analgesia of the thorax and forelimbs bilaterally in dogs [58]. Administration of an incorrect dose and inadvertent IV administration are likely the most common causes of systemic toxicity. Cats are most susceptible to developing methemoglobinemia after topical exposure to products containing benzocaine, which should be avoided in these species [59]. Toxicity of local anesthetics is additive by nature and may be due to multiple routes of exposure (topical and regional), mixtures of local anesthetics, or recurrent dosing. Post IV overdose, initial clinical signs include salivation, vomiting, and hypothermia. There is an immediate progression to pronounced CNS signs like nystagmus, muscle twitching, convulsions, tremors/seizures, and generalized CNS depression. These are then followed by cardiovascular effects like hypotension, bradycardia, and arrhythmias. Muscle tremors and seizures are managed with benzodiazepines (0.2–0.4 mg/kg IV) or propofol (2–4 mg/kg IV). Hypotension is treated with IV crystalloids and a vasopressor (phenylephrine 0.1–3 μg/kg/min). 20% lipid emulsion is recommended at 4 mL/kg initial bolus over 2 min followed by an infusion 0.25 mL/kg/min for 20–30 min. This extracts the LA from the aqueous plasma into a “lipid sink,” making it unavailable to the tissues [60]. Other supportive therapy includes airway management, treating arrhythmias, and cardiopulmonary resuscitation if cardiac arrest ensues. Small‐gauge needles (25–27 G) minimize the risk of nerve injury and are generally used for infiltration anesthesia and superficial blocks (e.g., dental blocks). Large‐gauge needles of a longer length (19–22 G, 1.5–3.5 inch) are used for deeper blocks (e.g., brachial plexus, epidural). Spinal needles with removable stylets are sharp, specially designed for penetrating the dura mater. Tuohy needles are blunt‐ended, curved at the distal tip, and used for epidural injections or placement of indwelling epidural catheters. Insulated nerve block needles, which are also blunt‐ended, are coated with a thin layer of nonconducting material over the entire length of the needle except for a small area at the distal tip. When the needle is connected to a peripheral nerve stimulator, low‐intensity currents (0.2–0.5 mA) can stimulate motor fibers, allowing successful identification of the target nerves. This device generates a square‐wave electrical current to locate target nerves, when used with an insulated needle (Figure 7.1). At sufficiently low currents (0.4–1 mA), if the stimulating needle elicits contractions of the muscle group of the target nerve, it indicates that the needle‐to‐nerve proximity is ideal for an injection of LA to cause a sensory block. If current is still conducted at 0.4 mA or less, it is likely that the needle is intraneuronal; it is advised to back the needle out prior to injection to avoid perineural injections, which may damage the nerve. Ultrasound allows for real‐time visualization of the stimulating needle, as well as identification of peripheral nerves and other anatomic structures such as vessels, muscles, and fasciae. Ultrasound guidance and electrolocation are often used together while performing nerve blocks, to increase the accuracy of nerve location and efficacy of the blockade. High‐frequency linear transducers (10–15 MHz) are suitable for performing most peripheral nerve blocks, and imaging superficial nerves that are less than 5 cm in depth. It is crucial that the anesthetist has: (1) a thorough understanding of the operative functions and basic principles of the ultrasound machine; (2) knowledge of anatomy and landmarks surrounding the target nerves; and (3) training to identify the echogenicity of the peripheral nerves, muscles, bones, fasciae, and blood vessels. Figure 7.1 A peripheral nerve stimulator in use for the placement of a sacrococcygeal epidural injection. Injection of LA around a particular nerve or nerve roots produces loss of sensation (sensory block) with or without paralysis (motor block) in that region, depending on the drug and its concentration. Different techniques for delivering LAs include topical anesthesia, infiltration anesthesia, neuraxial anesthesia, and peripheral nerve blocks. Some of the most common techniques are briefly reviewed below but fully described in the digital addendum. Additional resources for regional anesthesia strategies in small animals are listed at the end of this chapter. Topical lidocaine spray (2%, 4% or 10% solution) is available for laryngeal desensitization before tracheal intubation. A eutectic mixture of local anesthetics (EMLA) cream or patches (2.5% lidocaine and 2.5% prilocaine) prevent pain associated with peripheral catheter placement [61,62], superficial skin closure and blood sampling [63]. The cream is applied to the skin and covered with a dressing to enable absorption of the LAs. Time to achieve local effect is 45–60 min and it lasts up to 2 h after the dressing is removed. Transdermal self‐adhesive lidocaine patches (Lidoderm®, 5%, Teikoku Pharma) are used for surgical, incisional or trauma wounds. The manufacturer recommends placing the patch about 1 cm from the incision edge, covering the entire length of the incision to produce prolonged dynamic pain control and reduce requirement of systemic opioids postoperatively. However, some investigators found no adverse effects when the patch was placed directly over the incision [64]. This patch is cut to the desired length and shape. Plasma peak lidocaine concentrations are reached in 12–60 h in dogs and cats, with duration of analgesic effect lasting for 3–5 days [65,66]. Lidoderm and EMLA cream provide dermal anesthesia and minimal systemic absorption of the LA occurs via these methods. Incisional, intraperitoneal, and tissue infiltration of LAs is used for a variety of surgeries, wound care, multimodal analgesia, and in sterilization programs carried out in countries with limited drug availability [67]. Splash blocks are performed prior to closure of an incisional site to provide local analgesia [68] (Table 7.2). Wound infiltration using a multifenestrated catheter inserted into the surgical wound at the end of the procedure is a novel method of permitting repeated or continuous infusion of LAs for a prolonged period of time for postoperative analgesia [69] (Figure 7.2). The nerve endings in the tissues or in the area of infiltration are desensitized, thus preventing nociception. Infiltration technique should be avoided in infected tissues/wounds, as pH changes render LAs ineffective, and for masses where malignant cells may be spread beyond margins by the needle used. Care must be taken not to exceed the toxic dose for LAs (see Table 7.1). Table 7.2 Infiltrative or splash blocks. Figure 7.2 A soaker catheter placed by the surgical team for wound infiltration. A liposome‐encapsulated injectable suspension of bupivacaine (Nocita®, Elanco) is the newest FDA‐approved formulation for providing postoperative analgesia using a single injection technique at the time of incision closure for stifle surgery in dogs [70,71], and for regional analgesia following onychectomy in cats. It is also used for tissue infiltration during wound/incision closure (off‐label) and nerve blocks not at incisional sites (off‐label except for blockade of the radius/ulnar/musculocutaneous nerves). It consists of a unique liposomal delivery technology that encapsulates the bupivacaine with liposomal chambers. Post administration, these chambers break down, gradually releasing the bupivacaine over an extended period (72 h). Injection at closure prevents liposome disruption during surgical tissue manipulation. Slow bupivacaine release results in lower systemic exposure and decreased incidence of adverse effects. It is essential to use a 22 G or larger bore needle for the injection as smaller‐bore needles can disrupt the liposomes [72]. This liposomal bupivacaine may not be as effective when used for a splash block. Dosage for liposome‐encapsulated injectable suspension of bupivacaine is as follow. Epidural anesthesia is the administration of LA (or other drugs, e.g., opioids) into the epidural space (outside the dura) (Figure 7.3). If the drug is injected in the subarachnoid space, it is called spinal/intrathecal anesthesia. Epidural blocks are typically performed in anesthetized or heavily sedated patients, to relieve acute and chronic pain associated with a variety of surgeries and medical conditions. Analgesia occurs due to the bathing of the spinal nerve roots with the LAs. The extent of spinal nerve blockade following an epidural injection depends on the site of injection and the volume injected. Greater volumes cause cranial spread of the injected solution, increasing the area of desensitization. Also, higher concentrations of LAs are associated with more profound and prolonged blockade. Figure 7.3 Diagram demonstrating landmarks for epidural placement. Source:Courtesy of Teton NewMedia. Table 7.3 Lumbosacral (LS) epidural placement (Figures 7.4–7.7). a Total volume calculation options: 1 mL/10 kg for perianal, 1 mL/7 kg for hindlimb procedures, 1 mL/5 kg for thoracic/abdominal procedure. Avoid total volumes over 6 mL. Sterile 0.9% normal saline is used to add volume if desired. Figure 7.4 L7–S1 epidural placement in a canine patient in lateral recumbency. Figure 7.5 Canine patient in sternal positioning during an epidural technique. Figure 7.6 Threading of an epidural catheter in the L7–S1 space of a canine patient. Figure 7.7 L7–S1. Left: Epidural catheter placed and secured in a canine patient. Right: Final fixation of the epidural catheter with an adhesive plaster. Table 7.4 Sacrococcygeal (SC) or intercoccygeal (IC) epidural anesthesia placement. a Total volume calculation options: 1. To block pelvic limb motor function, use total volume of 0.2 mL/kg; 2. for tail and perineal surgeries use total volume of 0.1 mL/kg. Avoid total volumes over 6 mL. Sterile 0.9% NaCl is used to add volume if desired. Figure 7.8 Infraorbital nerve block in canine skeleton. Source: Courtesy of Anderson da Cunha. Figure 7.9 Infraorbital nerve block in the feline skeleton. Source:Courtesy of Anderson da Cunha. Table 7.5 Infraorbital nerve block. Figure 7.10 Maxillary nerve block via internal approach in the skeleton of a dog. Source: Courtesy of Anderson da Cunha. Figure 7.11 Maxillary nerve block via internal approach in the skeleton of a cat. Source: Courtesy of Anderson da Cunha Table 7.6 Maxillary nerve block. Figure 7.12 Approaches for a caudal inferior alveolar (mandibular) nerve block in a canine skull. Source: Courtesy of Anderson da Cunha. Figure 7.13 Caudal inferior alveolar (mandibular) nerve block in a feline skull. Source: Courtesy of Anderson da Cunha. Figure 7.14 Caudal inferior alveolar (mandibular) nerve block in canine patient. Source:Courtesy of Anderson da Cunha. Table 7.7 Caudal inferior alveolar (mandibular) nerve block. Figure 7.15 Mental nerve block in canine skull. Source: Courtesy of Anderson da Cunha. Figure 7.16 Mental nerve block in a feline skull. Source: Courtesy of Anderson da Cunha. Table 7.8 Mental nerve block. Figure 7.17 Retrobulbar nerve block. Source: Courtesy of Filipe Espinheira Gomes. Table 7.9 Retrobulbar nerve block: inferior temporal palpebral technique. Figure 7.18 Auriculotemporal nerve block. Figure 7.19 Greater auricular nerve block. Table 7.10 Auriculotemporal nerve block and greater auricular nerve block. Figure 7.20 Intercostal nerve block in canine patient. Figure 7.21 Intercostal nerve block in canine patient. Table 7.11 Intercostal nerve block. Table 7.12 Transversus abdominis plane block. Figure 7.22 Local anesthetic solution injected in the transverse abdominis plane using an ultrasound system with linear array transducer. D, dorsal; V, ventral; EO, external oblique abdominal muscles; IO, internal oblique abdominal muscle; P, peritoneum; TA, transversus abdominis muscle. Figure 7.23 Spinal needle (attached to the syringe with the local anesthetic solution) insertion cranial to the linear array transducer (13‐6 MHz) for placement of the transverse abdominis plane block. Table 7.13 Brachial plexus block (Figures 7.24–7.27). Figure 7.24 Diagram demonstrating brachial plexus block with a nerve locator and insulated needle. Source:Courtesy of Teton NewMedia. Figure 7.25 Brachial plexus block in canine patient using a peripheral nerve stimulator. Source: Courtesy of Patricia Queiroz‐Williams. Figure 7.26 Brachial plexus block in feline patient using a nerve stimulator. Figure 7.27 Brachial plexus block in canine patient using the blind technique. Figure 7.28 Diagram for radial, ulnar, and medial nerve block. Source:Courtesy of Teton NewMedia. Table 7.14 Metacarpal/metatarsal ring block. Table 7.15 Femoral (FNB) and sciatic nerve block (SNB) (Figures 7.29–7.31). Figure 7.29 Palpation of landmarks for a sciatic nerve block with the assistance of a nerve locator and insulated needle. Figure 7.30 Placement of the stimulating needle using an ultrasound system and a linear array transducer (13‐6 MHz) while performing a sciatic nerve block. Figure 7.31 Placement of the stimulating needle using a peripheral nerve stimulator, an ultrasound system and a linear array transducer (13‐6 MHz) while performing a femoral nerve block. To summarize, in both acute and chronic pain settings, opioids are a mainstay in providing analgesia. However, they are associated with possible detrimental effects, as well as being subject to shortages caused by things like natural disasters or pandemics. Nonopioid analgesia alternatives include an assortment of pharmacologic and nonpharmacologic interventions. Also, development of novel regional anesthesia techniques for small animals has grown tremendously in recent times. Veterinary clinicians and technicians should be aware of these potential options and consider them when developing a multimodal approach to pain management.
Chapter 7
Nonopioid analgesia alternatives and locoregional blocks
I. Nonopioid analgesic constant‐rate infusions
A. Ketamine
1.Clinical uses
B. Dexmedetomidine
1. Pharmacology
2.Clinical uses
C. Lidocaine
1. Pharmacology
2.Clinical uses
II. Oral gabapentinoids
1.Pharmacology
2.Clinical uses
III. Psychotropic drugs
1.Clinical uses
2.Dosage
IV. Cannabinoids
1. Endocannabinoid system (ECS) and pain
2.Clinical uses
V. Antiinflammatories
VI. Nonpharmacological pain therapy
A. Acupuncture
B. Physical rehabilitation
1.Superficial thermotherapy
2.Cryotherapy
3.Laser
4.Transcutaneous electrical nerve stimulation (TENS)
5.Extracorporeal shockwave therapy
6.Massage, therapeutic exercises
7.Glucosamine‐chondroitin, hyaluronic acid (HA) and platelet‐rich plasma (PRP)
8.Other nonpharmacologic techniques
VII. Local‐regional anesthetic techniques
A. Local anesthetics (LAs)
1. Pharmacology
Drug name
Onset of action
Duration of action
Comments
Lidocaine
1–3 min
1–2 h
Dose 4–6 mg/kg (dogs); 2–4 mg/kg (cats)
Toxic IV dose for CNS toxicity: 20–mg/kg (dogs); 12–mg/kg (cats)
Toxic IV dose for cardiovascular collapse: 80–mg/kg (dogs); 47 mg/kg (cats)
Authors suggest do not exceed 6 mg/kg in dogs and 3 mg/kg in cats by any route
Mepivacaine
3–5 min
2–3 h
Dose 4–6 mg/kg (dogs); 2–3 mg/kg (cats)
Toxic IV dose for CNS toxicity: 29–mg/kg (dogs)
Toxic IV dose for cardiovascular collapse: 80–mg/kg (dogs); unknown in cats
Authors suggest do not exceed 6 mg/kg in dogs and 3 mg/kg in cats
Ropivacaine
5–10 min
5–8 h
Dose 1–3 mg/kg (dogs); 1–2 mg/kg (cats)
Toxic IV dose for CNS toxicity: 4.8 mg/kg (dogs); unknown in cats
Toxic IV dose for cardiovascular collapse: 42 mg/kg (dogs); unknown in cats
Authors suggest do not exceed 3 mg/kg in dogs and 2 mg/kg in cats
Bupivacaine
2–20 min
4–8 h
Dose 1–2 mg/kg (dogs); 1 mg/kg (cats)
Toxic IV dose for CNS toxicity: 4–8 mg/kg (dogs); 4 mg/kg (cats)
Toxic IV dose for cardiovascular collapse: 20 mg/kg (dogs); 18 mg/kg (cats)
Authors suggest do not exceed 3 mg/kg in dogs and 2 mg/kg in cats
2.Analgesic action
3.Clinical considerations
4.Adjuncts used with LAs
5.Local anesthetic toxicity
B. Equipment used for local‐regional anesthesia
1. Needles
2.Peripheral nerve stimulator (PNS)
3.Ultrasound machine
C. Local‐regional anesthesia
1.Topical anesthesia
2.Infiltration anesthesia
Materials: 20–22 G needle, appropriately sized labeled syringe with local anesthetic solution, aseptic preparation, disposable or sterile gloves
Drugs: 0.5–0.75% bupivacaine 1–2 mg/kg for dogs and 1 mg/kg for cats; 2% lidocaine 3 mg/kg in dogs and 2 mg/kg in cats. Dilution can be achieved by 0.9% normal saline
Infiltrative technique: An infiltrative block involves distribution of local anesthetic into tissue, usually subcutaneous, around a mass or area of incision. Area is clipped and aseptically prepped prior to block
Splash technique: Splash block involves the “splashing” of local anesthetic over an incision after closure of the muscle layer, but prior to closure of the skin. The solution is given sterilely to the surgeon for administration or splashed sterilely into incision
3. Neuraxial anesthesia
a. Lumbosacral (LS) epidural anesthesia (Table 7.3)
Materials: Aseptic skin preparation supplies, sterile gloves, spinal needle/Tuohy needle of appropriate diameter and length, drugs in appropriately labeled syringes, +/– glass syringe, +/– nerve stimulator and appropriate insulated needle, +/– sterile 0.9% normal saline
Drugs: Preservative‐free (PF) morphine 0.1 mg/kg +/– bupivacaine PF 0.5–1.0 mg/kg or lidocaine PF 0.5 mg/kg not to exceed calculated total volumecssStyle=” cssStyle=”color:#0563C1″ a
Technique:
LOR: Place 1–2 mL of air in a glass syringe or loss of resistance syringe and secure to the spinal needle. Test for loss of resistance by injecting air to see if the plunger of the syringe advances smoothly. If there is no resistance, the needle is in the epidural space.
Nerve stimulation: When using a nerve stimulator, a nerve stimulation test (0.7 mA, 1 Hz, 0.1 ms) will elicit muscle contraction of the pelvic limbs and tail. However, it does not differentiate if the needle tip is in the epidural or subarachnoid space.
b. Sacrococcygeal (SC) or intercoccygeal (IC) epidural anesthesia (Table 7.4)
Materials: Aseptic skin preparation supplies, sterile gloves, spinal needle or hypodermic needle of appropriate diameter and length, drugs in appropriately labeled syringes, +/− nerve stimulator and appropriate insulated needle, +/− sterile 0.9% normal saline
Drugs: Preservative‐free (PF) morphine 0.1 mg/kg +/− 0.5% bupivacaine PF 0.5 mg/kg or 2% lidocaine PF 0.5 mg/kg not to exceed calculated total volumecssStyle=” cssStyle=”color:#0563C1″ a
Technique:
D. Peripheral nerve blocks
1. Head
a. Infraorbital nerve block
Materials: Disposable or sterile gloves, hypodermic needles (23–25 G) or 24 G catheter, drug solution labeled in 1 mL syringe
Drugs: 0.5% bupivacaine or 2% mepivacaine or 2% lidocaine 1–1.5 mg/kg. Generally, the dose per site is dependent on the patient size and weight and is 0.1–1 mL/site (dog) and 0.1–0.3 mL/site (cat)
Technique:
b. Maxillary nerve block
Materials: Disposable or sterile gloves, hypodermic needles (23–25 G), drug solution labeled in 1 mL syringe
Drugs: 0.5% bupivacaine or 2% mepivacaine or 2% lidocaine up to 1–1.5 mg/kg. Generally, the dose per site is dependent on the patient size and weight and is 0.1–1 mL/site (dog) and 0.1–0.3 mL/site (cat).
Technique (inside mouth approach):
Technique (from outside mouth):
c. Caudal inferior alveolar (mandibular) nerve block
Materials: Disposable or sterile gloves, hypodermic needles (23–25 G), drug solution labeled in 1 mLsyringe.
Drugs: 0.5% bupivacaine or 2% mepivacaine or 2% lidocaine up to 1–1.5 mg/kg. Generally, the dose per site is dependent on the patient size and weight and is 0.1–1 mL/site (dog) and 0.1–0.3 mL/site (cat).
Technique:
d. Mental nerve block
Materials: Disposable or sterile gloves, hypodermic needles (23–25 G), drug solution labeled in 1 mL syringe.
Drugs: 0.5% bupivacaine or 2% mepivacaine or 2% lidocaine up to 1–1.5 mg/kg. Generally, the dose per site is dependent on the patient size and weight and is 0.1–1 mL/site (dog) and 0.1–0.3 mL/site (cat).
Technique:
e. Retrobulbar nerve block
Materials: 22 G spinal needle (length is dependent on size of patient but is typically 3–5 cm), drug solution labeled in a 3 mL syringe, aseptic preparation, sterile gloves
Drugs: 2% lidocaine or 0.5% bupivacaine up to 1 mg/kg; maximum volume of injection is 1–3 mL in dogs and 0.3–0.5 mL in cats. Dilution is achieved with sterile 0.9% normal saline
Technique:
f. Auriculotemporal nerve block and greater auricular nerve block
Materials: 22–25 G hypodermic needle, 1 or 3 mL syringe, sterile gloves, aseptic preparation
Drugs: 0.5% bupivacaine or 2% lidocaine up to 1 mg/kg; maximum volume limited to 0.5–3 mL /site (dogs) and 0.2–0.5 mL (cats). Dilution can be achieved by sterile 0.9% normal saline.
Technique:
2. Thorax and cranial abdomen
a. Intercostal nerve block
Materials: 22–25 G hypodermic needle, 3 mL syringe, sterile gloves, aseptic preparation
Drugs: 0.5% bupivacaine or 2% lidocaine up to 2 mg/kg; maximum volume limited to 0.5–2 mL /site (dogs) and 0.5–1 mL (cats). Dilution can be achieved by sterile 0.9% normal saline.
Technique:
b. Transversus abdominis plane block
Materials: Clippers, aseptic skin preparation solutions, disposable or sterile gloves, 20–22 G 2.5 m (cats, dogs <5 kg) and 5–6 cm (dogs) hypodermic or spinal needles, +/– extension set, drug solution in appropriately labeled syringe, ultrasound guidance using high‐frequency linear transducer (7.5–15 MHz)
Drugs: 0.5% bupivacaine (preferred due to longer duration of action) 1 mg/kg or 2% lidocaine up to 2 mg/kg; maximum volume limited to 1 mL/kg/injection site. Dilution can be achieved by sterile 0.9% normal saline
Technique:
3. Thoracic and pelvic limb
a. Brachial plexus block
Materials: 20 22 G 2.5–5 cm spinal needle (patient size dependent), aseptic preparation, sterile gloves, labeled syringe containing drug solution, +/– nerve stimulator with appropriately sized insulated needle
Drugs: 2% lidocaine or 0.5% bupivacaine up to 2 mg/kg; maximum volume limited to 0.2–0.3 mL/kg. Dilution can be achieved by sterile 0.9% normal saline
Technique:
b. Metacarpal/metatarsal ring block
Materials: 22–25 G hypodermic needle, 1–3 mL syringe, local anesthetic solution, aseptic skin preparation, disposable, or sterile gloves
Drugs: 0.5% bupivacaine 1–2 mg/kg for dogs and 1 mg/kg for cats; 2% lidocaine up to 3 mg/kg in dogs and 2 mg/kg in cats. Divide this between limbs if procedure involves multiple limbs. Maximum total volume is limited to 0.1–0.3 mL/kg. This total volume is divided equally per site, depending on the total number of sites of injection. Dilution can be achieved by sterile 0.9% normal saline.
Technique:
c. Femoral (FNB) and sciatic nerve block (SNB)
Materials: 20–22 G insulated needle (length depends on size of patient), peripheral nerve stimulator, labeled syringe with drug solution, aseptic skin preparation, sterile gloves
Drugs: Up to 2 mg/kg of 0.5% bupivacaine (preferred due to longer duration of action) or 2% lidocaine. Total injection volume is 0.1 mL /kg for each FNB and SNB [84,87].
Technique:
VIII. Conclusion
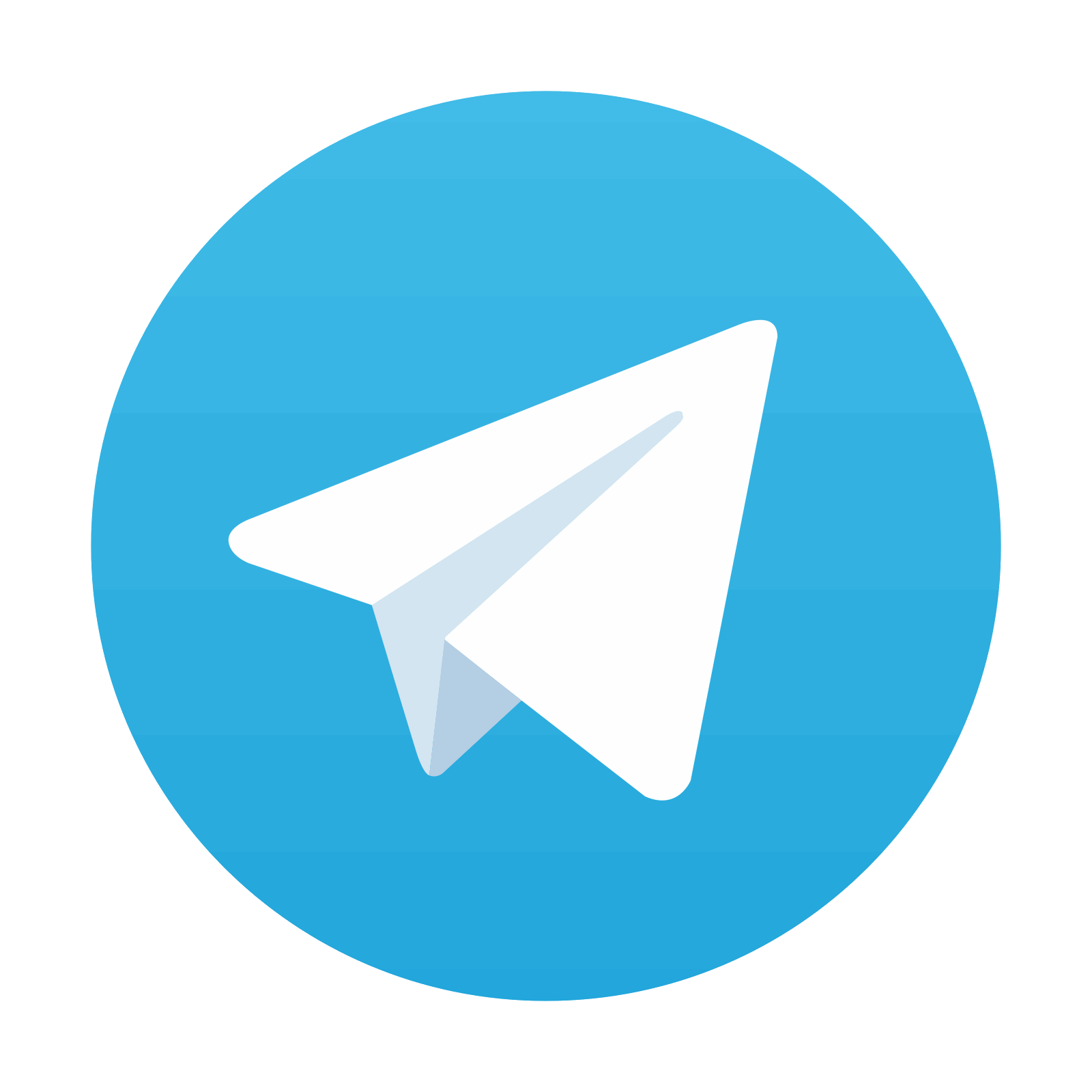
Stay updated, free articles. Join our Telegram channel

Full access? Get Clinical Tree
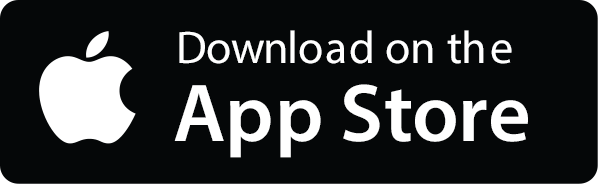
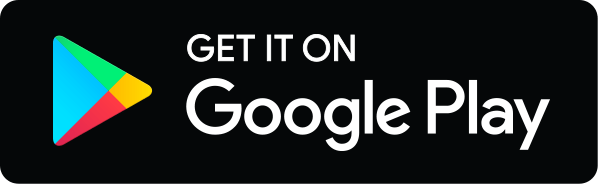