Stuart Clark‐Price Department of Clinical Sciences, College of Veterinary Medicine, Auburn University, Auburn, AL, USA Neuromuscular blocking agents (NMBAs) are a class of drugs used to produce skeletal muscle relaxation and paralysis most commonly to facilitate surgical and diagnostic procedure in anesthetized animals. Skeletal muscle paralysis can be useful for procedures that require precision and do not tolerate patient movement, such as ophthalmic surgery involving an intraorbital approach or repair of the cornea. Profound muscle relaxation achieved with the use of NMBAs can be helpful where muscle contraction impedes the progress of surgical repair, such as realignment of long bone fracture fragments. Animals having abdominal procedures may benefit from neuromuscular blockade through reduction of abdominal muscular tone that allows for easier apposition of the body wall for suturing. It is critical to understand that use of NMBAs results in paralysis of all skeletal muscle, including the diaphragm and intercostal muscles. This necessitates the use of assisted/mechanical ventilation to allow for gas exchange. It is important to remember that NMBAs do not induce unconsciousness nor do they provide any analgesia. Therefore, anesthetic and analgesic agents must be used in conjunction with these drugs. The use of this class of drugs without rendering an animal unconscious first is inhumane and unacceptable under any circumstances. A detailed review of neuromuscular physiology is beyond the scope of this chapter. For further detail, the reader is referred to medical textbooks. The neuromuscular junction is the synapse between a presynaptic motor nerve endplate and the corresponding postsynaptic muscle fiber. The neuromuscular junction is the site of action where a motor nerve transmits a signal to muscle to initiate the process of contraction. Most motor nerves are of the alpha subtype and are heavily myelinated and have high conduction velocities. After making initial contact with a muscle group, motor nerves divide into small terminal nerve fibers and innervate a small number of skeletal muscle fibers. Terminal fibers tend to innervate large numbers of fibers in muscle responsible for gross movement (e.g. postural muscles), whereas terminal fibers tend to innervate few fibers in muscles responsible for fine movement (e.g. extraocular muscles). Acetylcholine (ACh) is the chemical messenger that traverses the neuromuscular junction to relay information from the nerve to the muscle fiber [1]. Most ACh is synthesized in the terminal end of the nerve and packaged in vesicles. When an action potential reaches the terminal end of the motor neuron, calcium enters the cell and helps mobilize ACh vesicles through a voltage‐gated calcium‐dependent exocytosis. This results in multiple quanta (packets) of ACh entering the synaptic cleft. The released ACh diffuses across the synaptic cleft in order to interact with receptors on the postsynaptic muscle. Only about half of the ACh molecules will reach their receptors as acetylcholinesterase (AChE), present in the synapse, rapidly hydrolyzes any ACh molecule it meets to choline and acetate. The primary receptor for ACh on the postsynaptic muscle is the nicotinic cholinergic receptor. These receptors are part of a pentameric protein ion channel. Two ACh molecules must bind with the receptor to allow for activation (opening) of the ion channel (ligand‐gated ion channel). Once the ion channel is open, sodium and other cations flow into the cell, resulting in membrane depolarization and subsequent muscle contraction. The binding of ACh to a receptor is short‐lived and ACh disassociates from the receptor and diffuses back into the synaptic cleft. Abundant AChE within the synaptic cleft quickly deactivates the ACh and the free choline is taken up by the presynaptic nerve for synthesis of new ACh. A clear understanding of the physiologic events occurring at the neuromuscular junction is essential, as it is the site of action for drugs used to prevent muscular contraction. NMBAs can be broadly classified into two categories based on their mechanism of action: depolarizing NMBAs and nondepolarizing NMBAs. Depolarizing NMBA drugs are limited in clinical use to only succinylcholine. When administered, succinylcholine binds to postsynaptic nicotinic receptors in a similar way to ACh. This results in opening of the ion channel and muscle membrane depolarization. However, this depolarization results in disorganized muscle contraction, which then manifests mainly as short‐lived fasciculation. Succinylcholine does not dissociate from the receptor as quickly as ACh, thus prolonging the period of time that the ion channel is open. This prevents repolarization of the muscle and readying of the receptor to accept further ACh to initiate a subsequent muscle contraction. Skeletal muscle remains in a flaccid, depolarized state. Additionally, succinylcholine is not degraded by AChE, thus succinylcholine resides in the synaptic cleft longer, resulting in a prolonged effect. Succinylcholine has an onset of action of 30–60 seconds with a duration of action of 5–15 minutes. Although onset of action is quick and therefore useful for assistance with intubation, the short‐lived duration of action limits the drug’s clinical usefulness. Additionally, administration of succinylcholine can cause liberation of large quantities of potassium from muscle cells, particularly in animals with extensive muscle trauma, and can lead to life‐threatening arrhythmias. Nondepolarizing NMBA drugs are the current standard for patient paralysis in human and veterinary medicine. Drugs in this class work through competitive antagonism at the postsynaptic ACh receptors. These drugs do not cause depolarization of the muscle membranes but simply block the ACh receptors from being available to be bound by ACh. Because two ACh receptors must be bound by ACh to open the ion channel, nondepolarizing NMBAs only need to bind one of the receptors to render the ion channel inoperative. After administration, NMBA concentration quickly becomes higher than that of ACh at the receptors and thus the NMBA outcompetes ACh for receptor binding and exerts its clinical effect. Muscle relaxation occurs because ACh cannot evoke a response. The neuromuscular junction has an intrinsic wide safety margin due to high numbers of ligand‐gated ion channels and thus a large number of ACh receptors. This can be seen clinically, as greater than 70% of the postsynaptic ACh receptors must be blocked by an NMBA before any clinical effect will be seen. This necessitates a higher initial dosage of NMBA to get the desired effect but fractional dosages are all that are necessary to prolong the effect. Continued high dosages of drug will prolong the effect further but will not necessarily deepen the degree of muscle relaxation. There is very little published research into the use of NMBAs in food and fiber species. Much of the published literature relates to the use in sheep and swine for human research purposes. Thus, much of what is executed clinically is based on extrapolation from research in small animals and horses, and clinical observation of veterinary anesthesiologists. Currently, the most commonly used nondepolarizing NMBAs are atracurium, cis‐atracurium, vecuronium, and rocuronium. They can be divided into two categories based on their chemical structure, benzylisoquinolines and aminosteroids. Atracurium is a benzylisoquinolinium compound that has been clinically available since the early 1980s. It is composed of 10 different isomers that are variable in potency [2]. Additionally, atracurium is removed from circulation via nonspecific enzyme metabolism and Hoffman elimination, a nonenzymatic degradation related to pH and patient temperature [3]. In humans, atracurium causes release of histamine on injection and results in a decrease in blood pressure and an increase in heart rate [4]. However, although documented in laboratory species, the effect of atracurium causing release of histamine in food and fiber animals has not been reported. Onset of action of atracurium is variable based on dosage and pH but can be expected to range between 5 and 15 minutes with increased dosage and lower blood pH shortening the time to onset [5, 6]. Duration of action is also variable and is dependent on dosage, pH, patient temperature, patient age, and type of inhalation anesthetic used [2,5–7]. Higher dosage, lower pH, increased patient temperature and age, and use of isoflurane may result in a longer duration of blockade. Atracurium dosages reported in sheep are 0.4 and 0.5 mg/kg and for swine are 0.4 and 2.5 mg/kg [68–10]. It should be noted that higher dosages than used in other species appear to be necessary in swine to achieve clinically useful paralysis. Dosages for other ruminant species have not been reported. However, reported and clinically useful dosages in horses, dogs, and cats range from 0.11 to 0.6 mg/kg [2, 11, 12]. The author has used atracurium in several ruminant species successfully at an initial dose of 0.4 mg/kg IV and subsequent doses when needed at 0.2 mg/kg IV. Cis‐atracurium is one of the isomers of atracurium and is considerably more potent. Thus, a fraction of the dosage compared to atracurium is necessary for clinical use. There are no published reports of cis‐aracurium use in ruminants. In horses, complete blockade has been achieved with 0.04 mg/kg. Onset and duration of action are considered to be similar to atracurium. Vecuronium, an aminosteroid, became clinically available around the same time as atricurium. Vecuronium has a similar clinical profile as atracurium without histamine release and has become a useful alternative to atracurium. However, in horses there are reports of resistance to its neuromuscular blocking abilities [13]. Vecuronium has been described in sheep and it appears that this species has much higher sensitivity to vecuronium than small animals and humans [14]. An appropriate dosage for small ruminants is likely around 0.025 mg/kg [15]. For swine, a vecuronium dosage of 0.4 mg/kg has been reported to be useful [16].
5
Neuromuscular Blocking Agents
5.1 Physiology of the Neuromuscular Junction
5.2 Mechanism of Action of NMBAs
5.2.1 Depolarizing NMBAs
5.2.2 Nondepolarizing NMBAs
5.3 Clinically Useful NMBAs
5.3.1 Atracurium
5.3.2 Cis‐atracurium
5.3.3 Vecuronium
5.3.4 Rocuronium
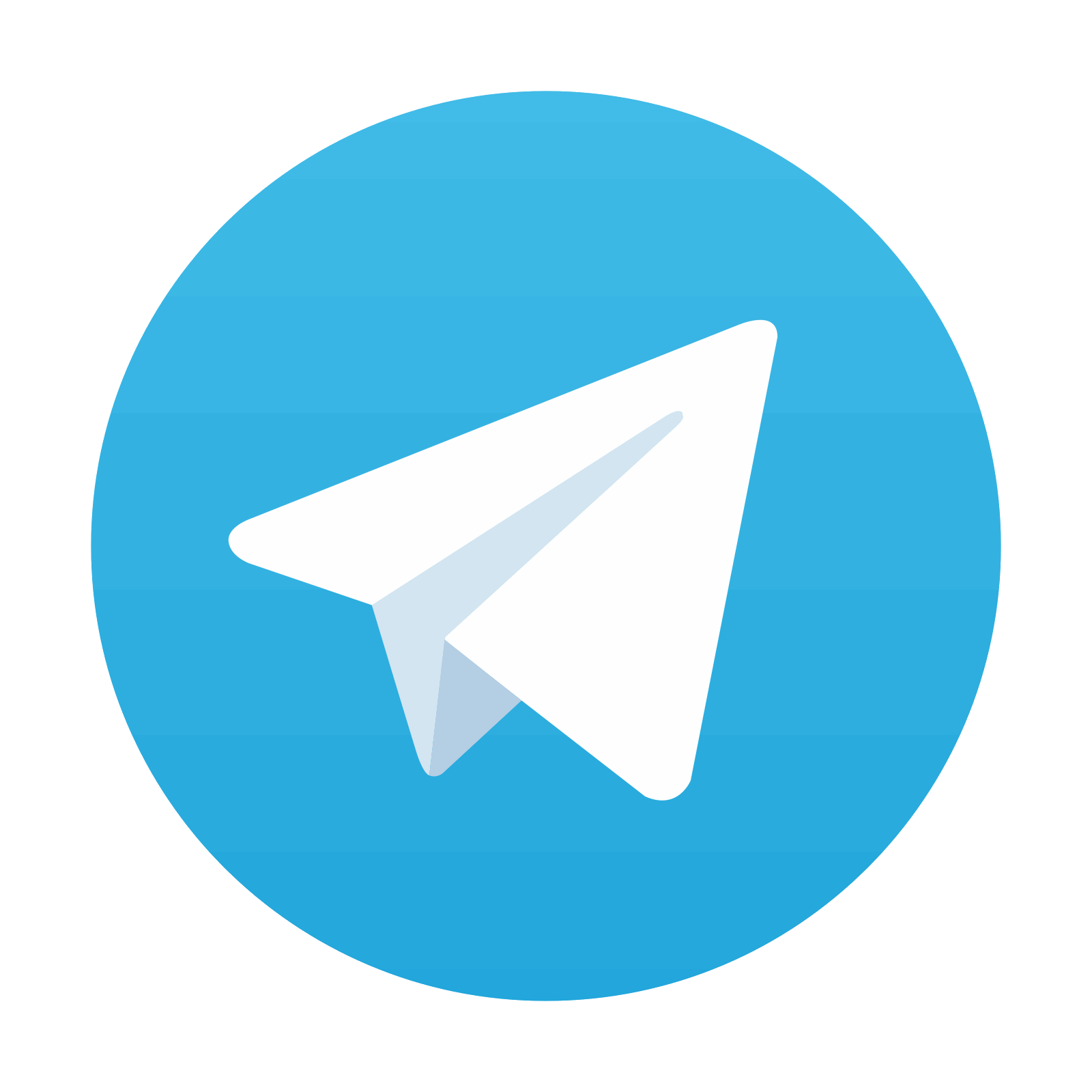
Stay updated, free articles. Join our Telegram channel

Full access? Get Clinical Tree
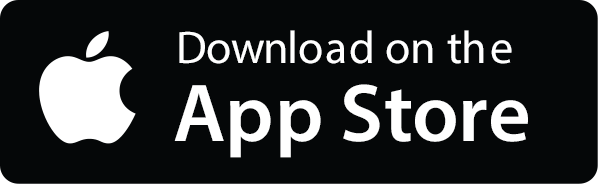
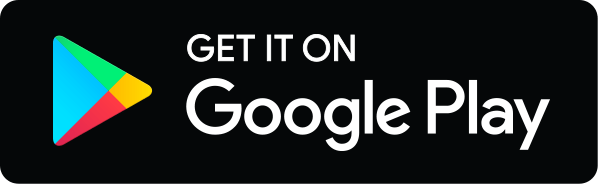