Chapter 23 Marcel Amstalden and Gary L. Williams Department of Animal Science, Texas A&M University, Texas, USA The neuroendocrine control of the estrous cycle involves the integration of multiple regulatory signals. These signals form an intricate network that permits the fine control of gonadotropin release required for the complex sequence of ovarian follicular development, steroidogenesis, and ovulation. Secretion of gonadotropin-releasing hormone (GnRH) into the hypothalamic–adenohypophyseal portal circulation is considered the primary endocrinological mechanism regulating gonadotropin synthesis and release. Internal factors (e.g., gonadal and metabolic hormones, growth factors and signaling molecules) and external factors (e.g., environmental chemicals, photoperiod and stressors) are perceived at the level of the central nervous system and control GnRH secretion directly or, more often, through intermediate pathways. Under stimulation by GnRH, gonadotropes synthesize and release luteinizing hormone (LH) and follicle-stimulating hormone (FSH). These two gonadotropins reach the gonads through the blood circulation and act in the ovary to stimulate follicle growth and maturation, ovulation of the preovulatory follicle, and synthesis of gonadal steroid and peptide hormones. Gonadal hormones, in turn, exert feedback actions at the central and adenohypophyseal levels to control the release of GnRH and gonadotropins. This intricate regulatory mechanism leads to dynamic changes in circulating concentrations of reproductive hormones during the estrous cycle (Figure 23.1). Figure 23.1 Relative changes in circulating concentrations of luteinizing hormone (LH), follicle-stimulating hormone (FSH), estradiol and progesterone during the estrous cycle in cows. The day on which estrous behavior is observed is denoted as day 0 of the estrous cycle. Circulating concentrations of progesterone increase after ovulation with formation and maturation of the corpus luteum, and remain elevated for the duration of the luteal phase. Release of prostaglandin (PG)F2α causes luteolysis, which is followed by a decrease in circulating concentrations of progesterone. The period of rapid follicular growth and maturation, and elevated concentrations of estradiol in circulation, that follows luteolysis characterizes the follicular phase. Elevated estradiol, in the absence of progesterone, leads to estrous behavior and the preovulatory surge of gonadotropin-releasing hormone (GnRH; top, graph on left). During the luteal phase, progesterone inhibits the frequency of pulsatile release of GnRH (top, graph in middle) and consequently the pulsatile release of LH. During the follicular phase, the escape from progesterone inhibition leads to increased frequency of GnRH (top, graph on right) and LH pulses, which supports follicular maturation and enhanced ovarian steroidogenesis. Fluctuations in circulating concentrations of FSH and estradiol are observed in association with initiation and progression of follicular waves. The length of the bovine estrous cycle ranges from 17 to 24 days, averaging 21 days. Day 0 of the estrous cycle is considered the day on which the female exhibits estrus (standing to be mounted) and other proceptive behavior such as mounting herdmates, restlessness, and vocalization. Estrus is induced by an elevation in circulating concentrations of estradiol-17β associated with enhanced follicular steroidogenesis during follicle development.1 Increased concentrations of estradiol reach a threshold 12–18 hours before onset of estrus, triggering a preovulatory surge of GnRH release and, consequently, a surge in gonadotropin release.2 Onset of the preovulatory surge of LH generally occurs coincident with the onset of estrus. Ovulation is induced approximately 30 hours after the onset of estrus and is followed by an abrupt decline in circulating concentrations of estradiol. Following ovulation, follicular cells undergo functional transformation into luteal cells, which synthesize and release progesterone.1 During corpus luteum formation and maturation, concentrations of progesterone in the circulation increase gradually to reach their peak approximately 8 days after ovulation. The period of progesterone dominance is defined as the luteal phase of the estrous cycle. During the luteal phase, mean circulating concentrations of LH are relatively low due to infrequent episodic release of LH. In contrast, concentrations of FSH and estradiol fluctuate in association with waves of follicular growth and atresia.3,4 At approximately day 17 of the estrous cycle in the cow, luteolytic pulses of prostaglandin (PG)F2α released by the endometrium cause luteal regression and a consequent decrease in circulating concentrations of progesterone.1 Therefore, the progesterone restraint of GnRH/LH release that characterizes the luteal phase declines and the frequency of episodic release of GnRH/LH increases. Increased gonadotropin support promotes follicle growth and maturation, enhanced follicular steroidogenesis, elevations in the circulating concentrations of estradiol and, ultimately, estrous behavior. The period characterized by low circulating concentrations of progesterone and enhanced follicular development leading to ovulation is termed the follicular phase. The cyclic changes in gonadotropin and ovarian hormone release, and resulting follicular activity, are usually recurrent, except when pregnancy is established or pathological conditions (e.g., undernutrition, disruption of normal luteolysis) are introduced. The dynamic changes in concentrations of circulating LH and FSH during the estrous cycle are largely representative of distinct changes in the pattern of episodic secretion of GnRH. During the luteal phase, the frequency of episodic release of GnRH, and the correspondent pulses of LH,5 is approximately one pulse every 4–6 hours.2 During the follicular phase, the episodic release of GnRH/LH is increased to approximately one pulse per hour.2,5 The follicular phase pattern of GnRH/LH release is essential for final follicle development and maturation, and enhanced steroidogenesis. The elevation in circulating concentrations of estradiol during late follicular phase leads to the preovulatory surge of GnRH. The GnRH surge is characterized by a sustained increase in concentrations of GnRH in the hypophyseal portal blood. At the onset of the surge, the pulsatile release of GnRH is of high frequency and high amplitude. With the progress of the surge, the pulsatile pattern of GnRH release switches to a continuous elevation of GnRH in the portal blood.2 This leads in turn to a massive discharge of LH from the adenohypophysis into the peripheral circulation, similar to that of GnRH in the portal circulation.2,5 Therefore, two modes of endogenous GnRH/LH secretion are characteristic of the estrous cycle: the tonic episodic release, and the preovulatory surge release.6 These patterns of GnRH/LH release are regulated primarily by estradiol and progesterone, and the negative and positive feedback effects mediated by them at the hypothalamic and adenohypophyseal levels. The cell bodies of neurons that synthesize GnRH in cattle are located in a continuum that extends from the preoptic area to the mediobasal hypothalamic region of the diencephalon.7,8 GnRH neurons project to the median eminence, where GnRH is stored in secretory vesicles and released adjacent to capillaries of the hypothalamic–hypophyseal portal vasculature on stimulation. Through this specialized vasculature that connects the hypothalamus and the adenohypophysis (Figure 23.2), GnRH reaches the adenohypophysis in functionally relevant concentrations, binds to the GnRH receptor on the surface of gonadotropes, and stimulates gonadotropin release. Disruption of the hypothalamic–hypophyseal vasculature by surgical lesion leads to decreased adenohypophyseal content and circulating concentrations of LH and FSH due to the lack of gonadotrope stimulation by GnRH.9,10 Activity of GnRH neurons and GnRH release in the median eminence are largely regulated by afferent neuronal signals and factors released by glial cells.11,12 Evidence for structural changes in glial cells that surround the capillaries of the median eminence also exists,13 and may contribute to the regulation of GnRH diffusion into the hypothalamic–hypophyseal circulation following a secretory episode. Figure 23.2 Diagram representing a sagittal view of the lower portion of the bovine brain and the hypothalamic areas involved in the neuroendocrine control of reproduction. In cattle, gonadotropin-releasing hormone (GnRH) neuronal cell bodies are distributed in a continuum that extends from the septum/preoptic area (POA) to the basal portions of the hypothalamus (a). GnRH neurons project to the median eminence (ME) where neuronal terminals are adjacent to capillaries of the hypothalamic–hypophyseal portal system. GnRH released in the ME reaches gonadotropes in the adenohypophysis (AP) and stimulates synthesis and release of luteinizing hormone (LH) and follicle-stimulating hormone (FSH). Low (b) and high (c) magnification images of GnRH-immunoreactive neuronal fibers that project basolaterally toward the median eminence and terminate adjacent to capillaries (*) of the zona externa of the ME. AHA, anterior hypothalamic area; ARC, arcuate nucleus; DMH, dorsomedial hypothalamus; MB, mammillary body; NP, neurohypophysis; OC, optic chiasm; pt, pars tuberalis of the adenohypophysis; POA, preoptic area; VMH, ventromedial hypothalamus; 3V, third ventricle. Positive and negative feedback actions of estradiol and progesterone at the level of the hypothalamus and adenohypophysis explain most of the alterations in the patterns of GnRH and LH release during the estrous cycle (Figure 23.3). The effect of progesterone in controlling the episodic release of GnRH/LH is well characterized: progesterone inhibits the frequency of LH release14 by its action at the level of the hypothalamus.15,16 However, the negative feedback effect of estradiol is more complex and appears largely additive to the action of progesterone.17 Estradiol positive feedback is the primary physiological mechanism driving the development of the preovulatory surge of GnRH and LH release during the late follicular phase.2,5,6 Interestingly, although progesterone can block the estradiol-induced LH surge in heifers and ewes,18,19 prior exposure to endogenous (luteal phase) or exogenous (pharmacological treatment) progesterone enhances the estradiol-induced LH surge.20 Figure 23.3 Patterns of release of luteinizing hormone (LH) during the estrous cycle in cows. Blood samples from the jugular vein were collected at 10-min intervals for 24 hours in four distinct phases of the estrous cycle in a cow. Modified with permission from Rahe et al.2 The mechanisms by which progesterone inhibits the frequency of episodic release of LH appear to be mediated by endogenous opioid peptides, particularly dynorphin.21 Studies in sheep have indicated that progesterone, by acting on progesterone receptor-containing dynorphin neurons located in the arcuate nucleus of the hypothalamus, inhibits the frequency of GnRH pulses via activation of endogenous opioid peptide neurotransmission. This effect appears to be mediated by the κ opioid receptor. Support for this mechanism of action is demonstrated by the observations that (i) intracerebroventricular injection of progesterone inhibits the pulsatile release of LH;16 (ii) antagonists of endogenous opioid peptide22 and of the κ opioid receptor,23 injected in the mediobasal hypothalamus, increase the frequency of LH pulses; and (iii) progesterone receptor-containing dynorphin neurons, located in the arcuate nucleus of the hypothalamus, project extensively to GnRH neurons in the mediobasal hypothalamus, but to a lesser extent to GnRH neurons in the preoptic area.23 Importantly, the population of GnRH neurons located in the mediobasal hypothalamus appears to be the major group of GnRH neurons activated during an LH pulse in sheep.24 This provides compelling evidence that the neuronal circuitry located in this area represents a pathway by which progesterone inhibits the frequency of GnRH/LH episodic release during the luteal phase. Although the mechanisms of progesterone negative feedback on regulation of LH release have not been extensively investigated in cattle, evidence indicates that endogenous opioid peptides have a role similar to that characterized in sheep.25 The estradiol negative feedback actions on LH release are more complex. During periods of elevated progesterone (e.g., luteal phase), estradiol enhances progesterone inhibition of LH pulsatility.16 This effect may involve direct actions of estradiol on endogenous opioid peptide neurons, including arcuate nucleus dynorphin neurons that are known to express estrogen receptor α and progesterone receptor in sheep.26,27 Because estradiol increases the expression of progesterone receptor in the arcuate nucleus of ovariectomized ewes,28 estradiol’s enhancement of progesterone negative feedback may involve increased responsiveness of hypothalamic neurons to progesterone actions. Estradiol negative feedback is also demonstrated by the ability of estradiol to decrease the amplitude of LH pulses during the follicular phase in ewes.29 However, this effect may be a consequence of the increased frequency of LH release observed during the late follicular phase2 or by inhibition of gene expression of the α-subunit of glycoprotein hormones (common to LH, FSH and adrenocorticotropin) and the β-subunit of LH.30,31 Estradiol appears to have a biphasic effect on secretion of LH during progression of the follicular phase. Administration of estradiol at physiological doses capable of inducing the preovulatory surge of LH leads initially to an acute reduction in the frequency of LH release.29,32 Evidence for this effect also exists during the late follicular phase of the estrous cycle.5 The brief period (~6 hours) of estradiol-induced inhibitory tone is followed by a considerable increase in the frequency and amplitude of GnRH/LH pulses and, subsequently, progression toward a large sustained elevation in concentrations of LH characteristic of the preovulatory surge (estradiol positive feedback; Figure 23.4).5,6 Although the mechanisms by which estradiol exerts the positive feedback are unclear, it appears to involve activation of estradiol-receptive neurons in hypothalamus and transmission of the estradiol signal to GnRH neurons for stimulation of the surge release at the median eminence.33 The ventromedial hypothalamus and the arcuate nucleus have been identified as critical sites for the positive effect of estradiol in ewes.34,35 Changes in norepinephrine, β-endorphin, glutamate and γ-aminobutyric acid (GABA), neurokinin B, and kisspeptin transmission may be implicated in the transmission of estradiol positive feedback. At the level of the adenohypophysis, estradiol enhances expression of the GnRH receptor.36 This effect is physiologically relevant because an increased response of the gonadotrope to GnRH during the late follicular phase might be critical for maintaining elevated secretion of LH during the preovulatory GnRH surge. Figure 23.4 Estradiol-induced gonadotropin-releasing hormone (GnRH) and luteinizing hormone (LH) surges in cows. Estradiol (1 mg) was injected intramuscularly at Hour 0. Cerebrospinal fluid (CSF) from the third ventricle and blood from the jugular vein were obtained at 10-min intervals. Concentrations of GnRH in the CSF (top) increased considerably and remained elevated for longer than 12 hours. Concentrations of LH increased consistently with elevations in GnRH and returned to pre-surge concentrations in approximately 10 hours. Modified with permission from Gazal et al.5 Prior exposure to progesterone enhances the estradiol-induced surge of LH release. This effect of progesterone appears to occur at both hypothalamic and adenohypophyseal levels. In ovariectomized ewes, the magnitude of the GnRH surge was greater in ewes pretreated with progesterone during an artificial estrous cycle than in ewes not preexposed to progesterone.37 Although evidence indicates that this effect occurs by alterations in GnRH secretion, direct effects in the adenohypophysis also occur. In ovariectomized ewes with the hypothalamic–adenohypophyseal connection surgically blocked, responsiveness to estradiol-induced surge of LH was enhanced in ewes pretreated with progesterone.38 Progesterone priming appears to influence responsiveness to estradiol feedback in cows as well.39,40 The control of FSH synthesis and release from gonadotropes is exquisitely regulated by GnRH and gonadal hormones, including steroid and protein hormones. Intra-adenohypophyseal factors may also have a role in regulating FSH secretion by paracrine actions.41 In addition, the existence of a hypothalamic factor with FSH-releasing activity distinct from GnRH has been proposed,42 although such a factor is yet to be identified. Similar to the release of LH, release of FSH has been shown to be pulsatile.43,44 However, using antisera produced against the β-subunit of FSH,45 which eliminates possible cross-reactivity with LH, yielded results in which distinct pulses of FSH release were difficult to detect in the peripheral circulation. This has been attributed in part to the long half-life of FSH in circulation.41 In addition, fluctuations in concentrations of ovarian peptides (inhibin, follistatin) and estradiol that occur during the estrous cycle play major roles in regulating the pattern of secretion of FSH in cattle.3,4,46 Nevertheless, studies in which concentrations of FSH were determined in blood samples collected from the hypophyseal portal vasculature in sheep have demonstrated that secretion of FSH is clearly pulsatile.41 Interestingly, although a considerable proportion of the FSH pulses coincide with episodes of GnRH release, many non-GnRH associated episodes are also observed. The GnRH-associated pulses of FSH observed in sheep occur coincidently with pulses of LH.41 In sheep, LH and FSH are cosynthesized in a subpopulation of gonadotropes,47 although the two gonadotropins are stored in distinct vesicles. Therefore, the coincident release of LH and FSH could reflect the concurrent exocytosis of readily releasable pools of vesicles containing gonadotropins. In cattle, however, there is a clearer distinction in FSH- and LH-synthesizing cells in the adenohypophysis.48 Nevertheless, FSH is clearly secreted following GnRH administration in cattle.39,49 In addition, the preovulatory surge of LH is accompanied by a surge in FSH.50,51 These observations indicate that although FSH and LH might be synthesized in distinct populations of gonadotropes, both types of gonadotropes are responsive to GnRH. However, the control of secretion of each gonadotropin appears independent, and the interaction of GnRH-dependent and GnRH-independent mechanisms are important for the fine control of FSH secretion. The mechanisms by which the non-GnRH associated pulses of FSH are generated remain unclear. Direct actions of gonadal hormones at the level of the adenohypophysis are believed to be a major regulatory mechanism. Peptide (e.g., inhibin) and steroid (e.g., estradiol) hormones act likely to control FSH synthesis and release at the level of the gonadotrope. Inhibin, a dimer of α and βA (inhibin A) or βB (inhibin B) subunits, is produced in follicular cells and acts as an endocrine factor regulating FSH release in gonadotropes. Inhibin suppresses FSH release in bovine pituitary cell cultures.52 The role of inhibin has also been shown in immunization studies. Basal circulating concentration of FSH and GnRH-stimulated FSH release is increased in ewes immunized against bovine inhibin.53 Similarly, mean concentrations of FSH increase in heifers immunized against inhibin, an effect associated with an increased number of small follicles present in the ovary.54 The effect of inhibin on the regulation of gonadotropin release appears specific to the control of FSH release because there is no clear effect of inhibin on the release of LH.55 In addition, it is unlikely that inhibin acts at the hypothalamic level for the control of GnRH secretion.56,57 Estradiol has been demonstrated to inhibit FSH synthesis and secretion in the adenohypophysis. The direct action of estradiol appears to occur through inhibition of FSH-β gene expression.58 However, studies investigating the replacement of estradiol and inhibin in ovariectomized ewes indicate that a combination of estradiol and inhibin negative feedback is required for full inhibition of the post-ovariectomy rise in concentrations of FSH.59 Additional ovarian hormones may contribute to the regulation of FSH release during the estrous cycle. Activin, a dimer of the inhibin β subunits (βAβA, βAβB and βBβB), and follistatin, a β-subunit-binding protein, are produced in follicular cells and can regulate FSH secretion. Although the endocrine actions of ovarian-derived activin and follistatin are unclear, these proteins, as well as inhibin, are synthesized in the adenohypophysis and may control FSH synthesis and release by paracrine mechanisms.60,61 Activin stimulates FSH synthesis and release, while follistatin inhibits activin actions by averting activin binding to the receptor. Inhibin, on the other hand, disrupts activin actions by binding to the activin receptor and inducing intracellular signaling via a β-glycan-mediated pathway. Therefore, the postovulatory elevation in circulating concentrations of FSH observed shortly after the preovulatory LH and FSH surges (Figure 23.1) is largely explained by changes in feedback actions of gonadal hormones at the level of the adenohypophysis following disappearance of the dominant follicle after ovulation, and decreased circulating concentrations of estradiol and inhibin. Increases in FSH in circulation at this time contribute to recruitment of the first follicular wave observed during the early luteal phase and at subsequent waves during the remainder of the cycle. The existence of a distinct molecule of hypothalamic origin with the ability to regulate FSH release has been proposed. Evidence in support of this hypothesis includes the observations that ablation of anterior hypothalamic neurons and their projections impair release of FSH, without clear effects on release of LH.62,63 In addition, inhibition of LH release without notable effects on FSH release has been observed after immunization against GnRH and inhibition of GnRH actions by administration of GnRH antagonists in sheep.41,64 Nevertheless, isolation and identification of a molecule that can be characterized as an FSH-releasing factor distinct from GnRH would be required for confirmation of this hypothesis. The nutritional control of gonadotropin release occurs primarily through the regulation of GnRH release in the median eminence. Severe nutrient restriction suppresses secretion of LH in reproductively mature and prepubertal cattle.65,66 However, short-term restriction of feed intake has a differential impact on gonadotropin release in mature and immature female cattle. In cows, the frequency of episodes of LH release is not affected by 2–3 days of fasting.66–68 In contrast, fasting for 48–72 hours reduces LH pulsatility in prepubertal heifers.69,70 Gonadal steroids have a marked effect on the nutritional regulation of gonadotropin release. Pulsatile release of LH is suppressed in ovariectomized estradiol-treated rats fasted for 48 hours.71 However, acute feed restriction does not suppress LH release in ovariectomized rats without estradiol replacement.71 Thus, gonadal steroid hormones interact with nutritional and metabolic signals to regulate gonadotropin release. Studies determining the concentrations of GnRH in blood samples collected from the hypothalamic–hypophyseal portal vasculature of normal-fed and feed-restricted ewes have demonstrated a considerable reduction in the frequency of GnRH pulses in response to feed restriction.72 The reduction in the frequency of LH pulses was greater than the reduction in the episodic release of GnRH because some low-amplitude pulses of GnRH were not accompanied by a pulse of LH. Therefore, diminished amplitude of GnRH pulses may also play a role in the overall reduction in mean concentrations of LH observed during undernutrition. A major consequence of low frequency of GnRH stimulation of gonadotropes in response to feed restriction is the decrease in synthesis of LH and GnRH receptors in the adenohypophysis.73 Pulsatile administration of GnRH in feed-restricted ewes restores circulating concentrations of LH to normal and increases pituitary expression of LH-β and LH-α genes.74 These observations are in concordance with the hypothesis that a high frequency of GnRH stimulation is necessary for optimal expression of the common α-subunit of adenohypophyseal glycoprotein hormones and the β-subunit of LH.75 Therefore, in conditions of low synthesis and storage of gonadotropins in the adenohypophysis and diminished numbers of GnRH receptors in gonadotropes, small-amplitude pulses of GnRH may not be sufficient to stimulate a clear episode of LH release. This may explain the observation that some small-amplitude pulses of GnRH are not followed by an episode of LH release.72 In addition, it is possible that small-amplitude pulses of GnRH prime the adenohypophysis for enhanced responsiveness to large-amplitude pulses that induce clear episodes of gonadotropin release.76 Nutritional and metabolic status are perceived largely at the level of the hypothalamus, although integration with signals controlling other areas of the brain, as well as peripheral organs, are important for the precise control of gonadotropin secretion and action in target tissues. The signals that mediate the nutritional control of gonadotropin release include nutrients (e.g., glucose, fatty acids, amino acids) and metabolic hormones (e.g., leptin, insulin, ghrelin). Glucose serves as a major energy source for the central nervous system and, along with fatty acids and amino acids, serve as signaling molecules to control cellular metabolic pathways.77–79 In sheep, insulin-induced hypoglycemia80 and treatment with 2-deoxyglucose,81 a competitive antagonist of glucose metabolism, inhibit secretion of LH. In cattle, hypoglycemia induced by phlorizin, an inhibitor of glucose transporter that increases renal excretion of glucose, was observed to lead to similar decrease in concentrations of LH.82 Although it is generally accepted that glucose is essential for normal hypothalamic function, the mechanisms by which glucose deficiency impairs the release of LH are unclear. Restoration of LH pulsatility in fasted monkeys occurs not only after feeding carbohydrate-based meals, which increase circulating glucose, but also after feeding protein/fat-based meals, which do not elevate blood glucose.83 Amino acids and fatty acids, in addition to serving as important precursor molecules for cellular biosynthesis, appear to also serve as signaling molecules for the control of reproductive neuroendocrine function.77 Glutamate and the glutamate-derived GABA are neurotransmitters that act directly on GnRH neurons to regulate firing activity and GnRH release.84,85 Hormones such as insulin, insulin-like growth factor (IGF)-1, ghrelin, and leptin are reliable markers of nutritional status and appear to play important roles in mediating the metabolic control of gonadotropin secretion.86 Leptin, a protein hormone synthesized and secreted primarily by adipocytes, has been extensively investigated as a metabolic signal for the control of reproductive neuroendocrine function in various animal species, including in cattle.87 Circulating concentrations of leptin correlate positively with adiposity88 and increase with elevations in body weight.89 In contrast, undernutrition decreases concentrations of leptin in the circulation. Treatment with leptin prevents the fasting-induced decrease in LH pulsatility in peripubertal heifers.70 In mature cows, although short-term fasting does not affect the frequency of LH release, leptin enhances LH secretion68 by acting at both the hypothalamus to increase the amplitude of GnRH pulses90 and at the adenohypophysis to increase sensitivity to GnRH.91 Mediators of leptin actions include neuropeptide Y (NPY) and proopiomelanocortin (POMC) neurons in the arcuate nucleus. These neurons contain leptin receptors.92 NPY is a potent stimulator of feed intake and feed restriction increases expression of the NPY gene.93 The anorexigenic actions of leptin are likely associated with the ability of leptin to decrease NPY expression.94 NPY inhibits GnRH release in cows5 (Figure 23.5). In contrast to its inhibitory effects on NPY gene expression, leptin enhances POMC expression.95 One of the peptide products of the POMC gene, α-melanocyte-stimulating hormone (α-MSH), has been shown to also exert direct stimulatory effects on GnRH neurons.96 Therefore, the actions of leptin on stimulating GnRH secretion appear to involve suppression of the inhibitory actions of NPY and stimulation of excitatory effects of α-MSH. Although NPY and POMC are believed to be major pathways involved in the control of GnRH release, it is likely that other neuronal networks contribute to the nutritional control of reproductive neuroendocrine function. Figure 23.5 Neuropeptide Y (NPY) inhibition of release of gonadotropin-releasing hormone (GnRH) in cows. The pulsatile release of GnRH (top panel) and luteinizing hormone (LH) (lower panel) is inhibited following intracerebroventricular administration of NPY (panels on right). Asterisk denotes detected pulses of GnRH and LH. Modified with permission from Gazal et al.5 Puberty is the process through which individuals acquire reproductive maturity. It involves physiological and behavioral changes that are largely driven by activation of gonadal function. In mammalian females, activation of the hypothalamic–adenohypophyseal axis, and the downstream effects of gonadotropins on gonadal function, support enhanced folliculogenesis and steroidogenesis (primarily estradiol synthesis) and leads to first ovulation.97 The onset of puberty is characterized by an increase in the episodic release of LH, resulting from a heightened frequency of GnRH release and stimulation of gonadotropes.98 Interestingly, exogenous estradiol induces a surge-like release of LH in prepubertal females,99,100 indicating that estradiol positive feedback is functional before reproductive maturation is complete. Therefore, a major limiting factor for the onset of puberty is the lack of high-frequency episodic release of LH necessary for enhanced estradiol synthesis that will lead to the preovulatory surge of GnRH and LH release. During the prepubertal period, estradiol negative feedback inhibition of episodic release of LH is enhanced. Ovariectomy in prepubertal heifers, and consequent removal of the major source of estradiol, is associated with a rapid increase in circulating concentrations of LH.101
Neuroendocrine Control of Estrus and Ovulation
Introduction
Functional anatomy of the hypothalamic–hypophyseal unit
Gonadal steroid control of gonadotropin secretion
Nutritional influences on hypothalamic–gonadotropic function
Pubertal development
Stay updated, free articles. Join our Telegram channel

Full access? Get Clinical Tree
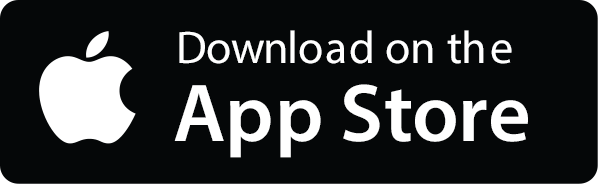
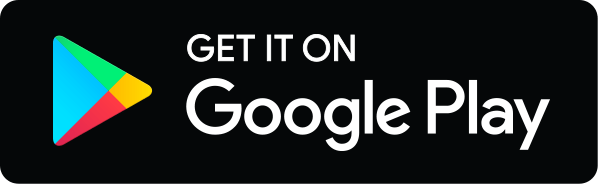