Grade
Description
1
Asymptomatic, mild headache, slight nuchal rigidity
2
Moderate-to-severe headache, nuchal rigidity, and/or cranial nerve palsy
3
Drowsiness, mild disorientation, and/or mild focal neurological deficits
4
Stupor and/or moderate-to-severe hemiparesis and/or dysphasia
5
Coma, decerebrate posturing, acute midbrain syndrome
Table 2
Grading of clinical state after aSAH according to the World Federation of Neurological Surgeons (WFNS)
Grade | Description |
---|---|
1 | Glasgow Coma Score (GCS) of 15, motor deficit absent |
2 | GCS of 13–14, motor deficit absent |
3 | GCS of 13–14, motor deficit present |
4 | GCS of 7–12, motor deficit absent or present |
5 | GCS of 3–6, motor deficit absent or present |
Ms. CD, age 45, right-handed African–American with Job’s syndrome, had a mild subarachnoid hemorrhage (SAH; Fischer’s grade 2; Hunt and Hess grade 2) from a right internal carotid artery (ICA) aneurysm. Cerebral arteriography confirmed the presence of a “mirror” aneurysm on the left ICA bifurcations. On the fourth day following SAH, the neck of the ruptured aneurysm was successfully clipped. Postoperatively, the patient transiently experienced right-sided hemiparesis attributed to a mild vasospasm of the left ICA, but she was discharged without neurological deficits on postoperative day 6. One month later, the aneurysm of the contralateral ICA bifurcation was electively clipped and a postoperative arteriogram confirmed complete occlusion of both aneurysms. As in the previously presented case, her GOS was 5 at the 6-month and 1-year follow-up visits. However, she struggled at work having memory and task-solving difficulties that were associated with anxiety and depression, both of which progressed to the point that she quit her job and went on disability.
These two patients, despite sharing the same diagnosis, corresponding severity of disease, and adequate and generally similar treatment but with obviously different outcomes, illustrate several points that are addressed in this chapter.
2 Assessment of Patient After Intracranial Aneurysm Repair
The initial issue is outcome assessment. Since 1938, when Dr. Walter Dandy clipped the first intracranial aneurysm (1), surgical and endovascular treatment has advanced dramatically because of the contributions of many neurosurgeons, such as the “giants” like Drs. G. Yasargil, V. Serbinienko, and G. Guglielmi, combined with the advances of technology (2). Nevertheless, since that time, intracranial aneurysm repair was, in the majority of cases, a lifesaving procedure. As a result, the goal of such treatment focused on assessing the patients’ physical well-being (Hunt and Hess, WFNS, Fisher, GOS; Tables 1–4) but not on their postoperative health, which the World Health Organization defined as “a state of complete physical, mental and social well-being and not merely the absence of disease or infirmity.”
0 | No blood present: No blood can be detected on CT |
1 | Mild: Thin localized layer of blood |
2 | Moderate: Thick layers of blood in two of the three subarachnoid cisterns, or in one, and on the cortical surface |
3 | Severe: Extended diffuse SAH with thick layers of blood in all arachnoid cisterns or in two and on cortical surface. Intracerebral hematoma >2 cm |
Score | Description |
---|---|
1 | Death |
2 | Persistent vegetative state |
3 | Severe disability |
4 | Moderate disability |
5 | Good recovery |
The mortality and morbidity related to treating intracranial aneurysms have declined significantly, establishing clipping and coiling as the standards of treatment (6). But a method of outcome assessment, e.g., the GOS, has remained the same for decades, despite a growing body of evidence that assessing patients’ mobility, speech, cranial nerves, short- and long-term memory, and/or sensory deficits (7) is inadequate for properly assessing the disease and its treatment impact on the patients’ fate (8, 9). Furthermore, the widely publicized advances of functional MRI and PET research focused on behavioral changes have brought an end to the myth of the “silent areas in the brain.” During the last 10 years, numerous studies have revealed that the brain regions regarded as “clinically silent” are not really silent. Nondominant hemisphere structures of the frontal, temporal lobes, and temporo-parietal junction and subcortical structures, such as the amygdala, striatum, etc., have been shown to regulate our social, moral, religious, and even political behaviors (10–12). These and other earlier observation of behavioral changes (13) after aneurysm treatment raised the question whether the GOS and the Quality Of Life (QOL) scale are proper tools to assess the outcome after this treatment. It becomes obvious that it is time to reevaluate these outcome measures and to develop more adequate tools allowing proper assessment consequences of both the surgical and endovascular treatments and vasospasm. This should facilitate a more accurate outcome prediction and allow for early intervention to prevent a poor outcome such as that of Ms. CD.
3 Sources of Neurobehavioral Changes After Aneurismal SAH
The second issue is that pathomechanisms that were responsible for such a dramatic difference in the outcome of these two relatively uncomplicated, successfully treated aneurysms remained undefined. For many years following the publication of the seminal paper by Weir (14), delayed cerebral vasospasm was recognized as “a single, more important cause of clinical deterioration and poor outcome after aneurismal SAH (aSAH) and successful treatment of intracranial aneurysm” (6, 15, 16). However, recently, there has been increased interest in other possible mechanisms that may contribute to the unsatisfactory results of the intracranial aneurysm treatment (17, 18). But, the question of what is the impact of initial rupture and subarachnoid clot formation on short- and long-term behavioral outcome remains unanswered. Also, it is still unclear how behavioral outcome is affected by surgery or endovascular treatment, whether there is a difference in the outcome between the patients who do or do not suffer SAH with or without vasospasm, as well as how the behavioral outcome is affected by development of postoperative delayed ischemic neurological deficits (DINDs) with or without coexisting vasospasm.
There are many mechanisms of brain injury that are unleashed by rupture of an intracranial aneurysm with violent high-pressure flow of highly oxygenated blood and formation of a blood clot in the subarachnoid space. Acutely, it is a dramatic increase of intracranial pressure (ICP) with a decrease of cerebral perfusion pressure (CPP) (19), with the possibility of developing acute hydrocephalus, intracerebral and intraventricular bleeding, “no-flow phenomenon,” as well as just recently confirmed development of early vasospasm (20). Subacutely, other insults to the brain occur as the result of different factors released locally from the blood, plasma, and clot, as well as the early inflammatory reaction, “no-reflow phenomenon,” electrolyte, and/or hormonal imbalance (e.g., the salt waste syndrome) (6, 21). The next aSAH-associated events occur in a delayed fashion (days 3–14) and these include delayed cerebral vasospasm (14, 22) and, for a long time considered being closely linked to it, clinical vasospasm (also referred to as DIND) (14). But, the findings of several recent studies revitalized interest in events other than vasospasm aSAH-related mechanisms (17, 18, 23) that can result in DINDs. Recently, this concept gained considerable interest after Dreier et al. (24, 25) proposed a new pathomechanism leading to DIND that does not require the presence of delayed cerebral vasospasm. These so-called spreading cortical ischemias (CSIs) that develop in the presence of blood in the subarachnoid space and decreased local availability of nitric oxide (NO) have recently been confirmed to exist in a patient after aSAH and are associated with clinical deterioration and the presence of CT-confirmed cortical infarctions (24–26). The chronic (occurring after more than 2 weeks after aSAH) reasons for clinical deterioration are hydrocephalus and emotional distress and/or depression. Of course, surgical trauma and blood flow changes evoked by the endovascular intervention add yet another insult due to decreased cerebral blood flow during cerebral arteriography (27) as well as an aneurysm repair both surgically or endovascularly (6, 13). However, the list of events that can affect the neurobehavioral outcome of aSAH and its treatments does not end here. The adjunct treatments of aSAH, such as CSF diversion via ventriculostomy, lumbar drainage or ventriculo-peritoneal shunt placement, administration of nimodipine, or triple H-therapy (28–31), may also impact neurobehavioral outcome.
There are also other issues that can influence outcome of the treatment, the neurobehavioral effects of which remain unknown. For instance, during the last 20 years, there has been increased success of treatment of aneurysms by endovascular therapies that lead to a decreased incidence of vasospasm. But most importantly, there is a significant increase of an incidental diagnosis of unruptured aneurysms, like in the case of AB (32–34), because of genetic studies as well as increased accessibility of CT/MRI. In the USA, even the slightest suspicion of intracranial disease leads to CT/MRI due to increased vigilance and awareness of symptoms of stroke after the successful “Brain is time” and “Decade of the brain” initiatives. This medically justifiable proactive attitude has significantly increased the number of patients whose aneurysms are treated before they rupture (34, 35). All these new developments have built new expectations from patients, their families, referring physicians, and rehabilitation therapist, especially with regard to outcome. Thus, it is clear that it is insufficient to assess only the patient after aneurysm repair by the GOS or even QOL scales. Patients and their families expect the patients’ posttreatment status to be adequately compared to “a state of complete physical, mental, and social well-being.” These expectations are shared also by the health providers building up a peer pressure to provide the outcome information addressing not only physical, but also social, behavioral, and economical aspects of life (6, 13).
4 Neurobehavioral Changes After Aneurismal SAH
Recent studies of neurobehavioralists and researchers, such as Hutter et al. (36), have brought the problem of neuropsychological consequences of aSAH to the forefront (9, 13, 37). Several studies have addressed (Table 5) this issue; nevertheless, no comprehensive and reliable conclusions can be drawn about the impact of acute, delayed, or chronic changes evoked by aSAH on neurobehavior.
Table 5
Clinical trials oriented toward recognition of arteriographical aSAH and vasospasm influence on neurobehavior
References | Leading cognitive dysfunction | Limitations |
---|---|---|
(38) | Moderate deficit in free recall | Caveat: Retrospective, small |
(39) | Free recall and short memory tests affected anterior communicating artery (ACoA) and vasospasm associated | Caveat: Retrospective, 2–14 years after SAH |
(40) | 31% of amnesia in the acute phase | Caveat: Retrospective, small, 23% of patient had the aneurysm trapped |
(41) | Cognitive defects and frontal lobe syndrome | Caveat: Retrospective, small, 1–7 years after aSAH and 1/3 with hydrocephalus |
(42) | The only prospective study; mild-to-moderate cognitive and memory dysfunction at GOS (5) | Caveat: Neurobehavioral dysfunctions unrelated to vasospasm, ischemia, or hydrocephalus |
(43) | 65% of the patients were impaired in at least one cognitive domain | Caveat: Retrospective, small |
Furthermore, most of these studies were focused on the presence of delayed vasospasm and its influence on neurobehavioral outcome (38, 40–43). They showed that 30–50% of all patients after aSAH suffer cognitive deficits, especially when tested for attention and memory (44), thus confirming a significant unfavorable effect of severe delayed cerebral vasospasm. But even the relatively simple issue of memory deficits in the setting of arteriographically confirmed delayed vasospasm after aSAH remains controversial because others (8, 39, 45) have not confirmed this observation. Of course, and it is not a surprise, there is no information about how early damage to the brain or endovascular and surgical aneurysm repair can affect neurobehavior. Nevertheless, as mentioned above, several factors beyond vasospasm have also been linked to the neurobehavioral deficits (Table 6).
There are several important conclusions emerging from these studies: (1) neuropsychological results predict the patient’s ability to resume work (47) and (2) the time frame of functional recovery remains unclear (44). Other important findings were (3) a significant discrepancy between a GOS 5 and poor neuropsychological results and (4) a lack of difference in the extent of neurobehavioral deficits between patients operated for aSAH and those with aSAH but without surgery (44). Furthermore, the International Study of Unruptured Aneurysms revealed that patients do better when operated upon before the aneurysm ruptures producing aSAH (49). These findings demonstrate the influence of early versus late aSAH-related events on outcome (17, 18, 20). Practical consequences of all these findings are difficult to overestimate. The patient whose clinical status was assessed at GOS 5 after aSAH and aneurysm treatment may still need significant neuropsychological diagnostics and rehabilitation. Thus, there is no doubt that establishing the cause and pathophysiology of reported neurobehavioral changes should facilitate the diagnostics and treatment options for aSAH. A properly designed experimental model could provide in-depth insight into causes, pathophysiology, and pathomechanisms of these deficits, thereby providing a tool to develop targeted treatments and rehabilitation.
5 Experimental Models to Study Outcome of Aneurismal SAH
For many years, we have developed excellent experimental models of SAH, but all were focused on studying the development, presence, and effects of delayed cerebral vasospasm (50). The focus was so narrow because these models were also supposed to address “vasospastic syndrome,” also known as “delayed cerebral dysfunction” (51) or DIND, since it was evoked by the presence of cerebral vasospasm (14). Recently, this dogma has been questioned and slowly abandoned (17, 18, 20). The new research approaches have refocused on the neurobehavioral changes evoked by aSAH acutely because of the severity of the initial SAH or the delayed development due to the prolonged presence of blood clot(s) on the surface of the brain. Both the acute and delayed mechanisms are at least as important as vasospasm and its neurological consequences in assessing the effects of treatment for intracranial aneurysms. Nevertheless, none of the models addresses the influence of these events on neurobehavior because all were developed to study delayed cerebral vasospasm (52).
5.1 aSAH Animal Models
There are numerous animal models that mimic the clinical situation of aSAH. A detailed description of these models can be found elsewhere. We also recommend two recently published excellent reviews on this topic (52, 53). Table 7 presents the summary of the models and their experimental use with references to the actual SAH-evoking procedure.
Table 7
Experimental models of aSAH and/or vasospasm
Species | SAH method | Effect | References | ||||
---|---|---|---|---|---|---|---|
Artery puncture intrathecal injection | Single puncture intrathecal injection | Double puncture intrathecal injection | Clot puncture intrathecal injection | SAH | Vasospasm | ||
Mouse | + | + | + | − | + | ? | |
Rat | + | + | + | − | + | +/− | |
Rabbit | + | + | + | − | + | +/− | |
Cat | + | + | + | + | |||
Pig | + | + | + | + | + | ||
Dog | + | + | + | + | + | + | |
Nonhuman primate | + | + | + | + | +/− | + |
All these animal models of aSAH mimic hemorrhage by a vessel puncture (intra- or extraluminal), single or double intrathecal blood injections, and clot placement in a mouse, rat, rabbit, cat, dog, pig, and nonhuman primate. But their relevance for studying aSAH-unleashed mechanisms leading to neurological and neurobehavioral dysfunction remains unknown or limited (52). It becomes clear that as we have sought to establish the cause of vasospasm all the models have been developed to address it. Among them, the nonhuman primate model with unilateral craniectomy, Sylvian fissure dissection, and clot placement (78) has been widely recognized as the most reliable and clinically relevant model of vasospasm (50, 53), but it has been inadequate to study the early post-SAH brain damage or DINDs. Other small animal models with disruption of the vessels or intrathecal injection of blood seem to better address the effects of acute aSAH-related events on brain injury (52). But, the issue of proper experimental models to delineate pathomechanism(s) of direct brain damage evoked by aSAH remains to be solved. Establishing the model(s) addressing these different pathomechanisms becomes even more urgent as the assessment of neurobehavioral changes after aSAH becomes a routine outcome measure. The next table (Table 8; after Hutter (44)) summarizes the neurobehavioral changes that were reported in patients after aSAH and treatment of aneurysms that should be addressed by the animal models.
Table 8
Possible neuropsychological and psychopathological changes after aSAH (modified after Hutter (44))
Visual field defects |
Neglect |
Defects of visual and spatial constructive capacities |
Distortions of face and object recognition |
Memory disorders |
Language disturbances (aphasias) |
Deficits in problem solving |
Apraxias |
Attention deficits |
Mental rigidity |
Dementia |
Deficits of concentration capacity |
Personality changes/emotional disorders |
Behavioral disorders |
Organic personality disorder |
Apathy and loss of interest |
Impairment of social judgment |
Aggressiveness or rage |
Affect lability, disinhibition, and/or syndrome (depression) |
Organic psychosocial maladjustment |
This list of neurobehavioral changes after aSAH is a basis for the animal tests (presented in Table 9) to assess the neuropsychological and neurobehavioral changes. We also describe their applicability to the existing SAH models and propose adequate model(s) to study aSAH-related mechanisms influencing behavioral and neurological outcomes.
Table 9
Behavioral test assessing signs and deficits in animal models of hemorrhagic stroke
Defects | Test | Animal species | ||||||
---|---|---|---|---|---|---|---|---|
Mouse | Rat | Dog | Cat | Rabbit | Pig | NHP | ||
Visual defects | No appropriate tests available | |||||||
Neglect | Test for neglect | |||||||
Visual spatial capacities | No appropriate tests available | |||||||
Distortions of face and object recognition | Matching/nonmatching tests | See below in memory models | ||||||
Memory | Morris water maze Radial arm maze Radial arm water maze Barnes maze Y- or V-maze T-Maze Other types of mazes Passive avoidance learning Active avoidance Matching/nonmatching tests Displacement Obstacle memory | (103) (114) | (52) (94) | (129) | (111) (138) (149) | (96) (120) (109) | (107) (146) | |
Language disturbances (aphasias) | No appropriate tests available | |||||||
Deficits of problem solving | Matching/nonmatching tests Go-trial reaction time (GoRT) task and stop-signal reaction time (SSRT) task Attentional set shifting Other types of mazes String pulling task | See above in memory models See below in attention deficits See below in attention deficits See above in memory models | ||||||
(152) | ||||||||
Apraxia | No appropriate tests available | |||||||
Attention deficits | Five-choice serial reaction time task Attentional set shifting GoRT task and SSRT task | (158) | ||||||
Mental rigidity | No appropriate tests available | |||||||
Dementia | See memory models above | |||||||
Deficits of concen-tration capacity | No tests available | |||||||
Personality changes/emotional disorders/behavioral disorders | Elevated-plus maze Open-field test Light–dark exploration test Social interaction test Shock probe defensive burying test Novelty-induced hypophagia | (116) (116) | (180) | |||||
Attentional set shifting | See above in attention deficits | |||||||
Affect lability, disinhibition, and/or syndrome (depression) Organic personality disorder | Forced swimming test Tail suspension test No appropriate tests available | |||||||
Apathy and loss of interest | No appropriate tests available | |||||||
Impairment of social judgment | No appropriate tests available | |||||||
Aggressiveness or rage | Social interaction test | See above in personality changes/emotional disorders/behavioral disorders | ||||||
Organic psychosocial maladjustment | No appropriate tests available |
6 Experimental Models of Neurobehavioral Changes
6.1 Visual Field Defects
Normal vision is essential for most of the below-described neurobehavioral tests. A detailed ophthalmic examination must be performed to differentiate pathologies in the eyes and the brain. No appropriate behavioral tests are available for precise evaluation of visual field defects.
6.2 Neglect
Cats, rats, and monkeys serve as models of neglect. The animal is placed in a special arena or testing/discrimination apparatus, where high-incentive stimulus is introduced first in front of the animal, than right and left from the animal. Failure to turn the head toward the high-incentive stimulus indicates the neglect, typically due to a lesion in the contralateral posterior and inferior parietal cortex or the superior colliculus (cat (80–82); rat (83–85); and primate (86–88)).
6.3 Defects of Visual and Spatial Constructive Capacities
No appropriate behavioral tests are available.
6.4 Distortions of Face and Object Recognition
The delayed nonmatching-to-sample test (DNMS) can be used for object recognition and is described in the “memory disorders” section.
6.5 Memory Tests
6.5.1 Morris Water Maze
The Morris water maze (MWM) is the most common rodent behavioral test used to evaluate several types of learning (i.e., procedural, matching to place, cue) as well as spatial, procedural, and working memory. Pigs can also be used in this test. The animal is placed in the center of the water tank (or water swim field) and allowed to swim to find a slightly submerged platform that cannot be seen while swimming. Animals are guided by the cues that are outside the pool and are trained to recognize these cues over several training practices before the test. Latency to reach the platform, distance swum, speed swim, and time spent in different areas of the tank serve as measures for this test (mice (89–92); rats (93, 94); rats and mice (95); and pigs (96)).
6.5.2 Radial Arm Maze
Rodents and pigs have been used in the radial arm maze (RAM) to study spatial memory/navigation; learning; working, reference, procedural, retrospective, and prospective memory; as well as conditioned-cue preference and visual discrimination. Several varieties of the test are available. The food-deprived trained animal is placed in the center of the maze with 8–17 equally spaced, door-controlled arms, which the animal has to enter to find a food or water reward placed in some of the arms. The maze is usually located in the room with visual cues. Errors in the first ten choices and/or total errors per session serve as measures for RAM (mice (92, 97); rats (94, 98, 99); rats and mice (95); and pigs (100–102)).
6.5.3 Radial Arm Water Maze
6.5.4 Barnes Maze
The Barnes maze represents a dry version of MWM with some elements of RAM. Rats and mice have been used in it. Several varieties of the test are available. The maze consists of a round open platform with 8–18 holes that appear identical around the perimeter. One of these holes leads to a rescue/escape cage under the platform. The animal is released in the center and is exposed to intense light or to a loud noise. In response to this intense stimulation, the animal searches for shelter and enters one or more of the 8–18 holes around the platform.
6.5.5 Context Conditioning
The first conditioning experiments by Ivan Pavlov (Russian physiologist, psychologist, and physician) with animals given a signal (light or buzzer) followed by food (testing first-order reflexes) laid the background for several areas of neurobiology, memory, and incentive behavior. His legacy was continued by a Polish neurophysiologist, Konorski, who significantly expanded understanding several areas of neurobiology by discovering secondary conditioned reflexes, operant conditioning, and other behavioral tests (104–106).
6.6 Y- or V-mazes
Rodents are typically evaluated in a Y-maze. New World monkeys have been tested in a V-maze. Cognition, short- and long-term memory, and spatial working memory are assessed in these mazes. Several modifications of the test can be conducted with different levels of difficulty and depending on the specific types of cognition tested. (1) In one variety of the test, animals are placed in a Y-shaped maze for a set period of time (typically, 6–8 min). (2) In another variety, one arm of the Y-maze is blocked and the animal is allowed to explore the two arms for 15–30 min, after which the animal is removed from the maze for a few minutes (or up to several hours), and then placed back into the maze, with all arms open, to explore for 5 min. To test long-term memory, this test can be repeated days to weeks later.
This test measures the number of arms entered and the sequence of entries. A high alternation rate suggests the presence of preserved cognition. Measured parameters include (a) the first arm entered—the animal should enter the previously blocked arm first on the second trial; (b) amount of time spent in each arm; and (c) total number of arm entries (rats (94, 99); mice (95, 97); and monkeys (107)).
6.7 T-Maze
Rats, mice, pigs, cats, and monkeys have been evaluated in T-mazes to study cognition, short-term memory, and spatial working memory. In rodents, normally, on the second trial, the initially unvisited arm should be visited first (spontaneous alternation).
The tested animal is started at the base of the T-shaped maze and allowed to enter one goal arm with the other arm being closed. The second trial is given in quick succession with both arms open. Different varieties of the test have been utilized (particularly for pigs and cats), adding L-shaped returns to the construction of the maze, additional trials, and various delays to test various aspects of memory and cognition (mice (97, 108); rats (94, 99); pigs (109, 110); cats (111); and monkeys (112, 113)).
6.8 Other Types of Mazes
A number of protocols utilizing context conditioning can be performed with the above-mentioned and other types of mazes [e.g., zero maze, cross-maze, elevated-plus maze (EPM), and 3D maze] to test different aspects of cognition, memory, and anxiety in rats, mice, and pigs (mice (92, 114, 115); rats (94, 99); rats and mice (95); pigs (116); and primates (117, 118)). An original 12-section maze has been used by Jansen et al. (119) to evaluate spatial memory in pigs. An original “triple T water maze” was successfully used by Hammel et al. (120) in pigs. Virtual computer mazes allowing concurrent imaging of the brain have been utilized in monkeys for various aspects of memory and problem solving (121–123).
6.8.1 Passive Avoidance Learning
The passive avoidance learning paradigm has been used to assess memory and learning to avoid noxious stimuli in rodents and monkeys. A rat or mouse is placed in the cage consisting of two compartments: one painted white and another—black. The floor of the black compartment can be electrified to provide mild foot shock. Rats and mice naturally prefer a dark compartment, but they must learn to avoid a mild aversive stimulus (in this case, mild foot shock) by remaining in the well-lit side of a two-chamber apparatus and not entering the dark, where it receives the aversive stimulus. Measures for passive avoidance learning in rodents include escape/avoidance latencies and percentage of failures in test and control groups.
Monkeys are presented with aversive or potentially dangerous stimuli (e.g., rubber snake, syringe, capture net, or large doll “staring” forward) and a range of fear-related behaviors, including defensive behavior, tension behavior, body postures, gaze direction, etc., are recorded (rats (52, 94); mice (92, 97, 114); and monkeys (124, 125)).
6.8.2 Active Avoidance
The active avoidance test has been used in rats, mice, pigs, dogs, and cats to evaluate learning/memory to avoid noxious stimulus. In rodents, it can be conducted in either a two-compartment apparatus, where the animal is required to cross to the adjoining chamber to escape shock, or in a single chamber with a single vertical pole that the mouse can jump onto when shock is administered. The animal learns to avoid shock based on the presentation of a light or other cue. In a unidirectional variant of the test, the animal is always shocked in the same compartment. In the bidirectional variant, the animal learns to monitor for cues in both compartments to predict shock.
6.8.3 Matching/Nonmatching Tasks
Fasted animals are rewarded with various treats if they choose the same or a different cue, one of the two, or more depending on the test, that they had a chance to examine before the test. Depending on the test, various delays may be introduced before or between cues. Typical tests include delayed matching-to-sample test (DMTS), DNMS, delayed matching-to-position test (DMTP), and delayed nonmatching to-position test (DNMP). Rats, mice, dogs, cats, pigs, and monkeys can be subjects for these tests.
Rodents are typically tested in a variety of operant chambers or in Y-maze (108, 132, 133). Wisconsin General Test Apparatus or WGTA, National Center for Toxicological Research (NCTR) Operant Test Battery (OTB) and various types of touch-screen computer-based systems, e.g., Cambridge Neuropsychological Test Automated Battery (CANTAB), are used for monkeys.
The percentage of errors and latency to make a choice (or to err) are measured. Matching/nonmatching tasks are used to assess working memory, short-term memory, visual–spatial learning/memory, object recognition, and problem solving (mice (108, 126, 134); rats (52, 135–137); pigs (109); cats (138); dogs (139–141); and monkeys (142–147)).
6.8.4 Other Memory Tests
Displacement
Here, the animal observes placement of a nonfood object (e.g., a toy) in one of the two bins and is then ordered to retrieve the object. Various delays, placement of a bin with an object on a rotating beam followed by 90° rotation of the beam, and placement of a screen between the animal and the rotating beam or darkness may be introduced between demonstration of the object and the command to retrieve it.
The test has been conducted in dogs and cats to assess working memory and cognition. The percentage of errors serves as a measure for this test (148, 149).
Obstacle memory
In this test, a cat is trained to walk on a slow treadmill and the dorsal surface of its hind paw is randomly (e.g., approximately every 20 steps), transiently, and gently touched/“hooked” creating hyperflexion. The degree of hyperflexion serves as a measure. The test has been designed to assess working memory in cats (150, 151).
6.9 Language Disturbances (Aphasias)
No appropriate behavioral tests are available.
6.10 Deficits in Problem Solving
Matching/nonmatching tasks are used—see above.
6.11 Go-Trial Reaction Time Task and Stop-Signal Reaction Time Task
See below in attention deficits.
6.12 Attentional Set Shifting Test
See below in attention deficits.
6.13 String Pulling Task
In this test, a dog attempts to retrieve a food treat connected via string by pulling the string. There is a transparent barrier between the dog and the treat, and the string is passed under the barrier to the dog. Either one or two strings under various angles might be available to the dog to manipulate, but the treat is attached only to one string. Successful retrieval of the treat required the animals to pull the appropriate string either by pawing or grasping with their jaws until the treat emerged from under the barrier.
Measures for this test are success rate and time required to solve the problem.
This is a means-end task and can be used to assess problem solving in a dog (152).
6.14 Attention Deficits
6.14.1 Five-Choice Serial-Reaction Time Task
The Continuous Performance Test (CPT) is the most characterized model of human attention; it has been used for more than 50 years to measure attention performance (153). The five-choice serial reaction time test (5-CSRTT) is the preclinical analog of the CPT (154).
Rats, mice, and monkeys have been tested in the 5-CSRTT. Rodents are typically tested in a chamber with five nose-poke holes with lights positioned in a horizontal line on one wall. On the wall, directly opposite the holes is a small opening with a reward dispenser. Before testing, the animal has to be food restricted and trained to nose poke for a food reward. During testing, numerous trials are given.
For monkeys, 5-CSRTT is a part of touch-screen computer-based system, the CANTAB. Accuracy and time to respond are measures for this test. Trials are scored as successes or failures. Successful trials are scored when the animal correctly nose pokes into the illuminated aperture.
6.14.2 Attention Set Shifting Test and Reversal Learning
The Attention Set Shifting Test (AST) represents the animal version of a diagnostic test used on humans in the clinic, the Wisconsin Card Sorting Test (WCST), and has been used on rats, mice, and monkeys.
Rodents are trained to dig in bowls for a food reward on a series of three simple discriminations (cues), e.g., texture, odor, and digging medium. Two bowls are presented in each trial, only one of which is baited. For the simple discrimination, the trained animal has to select the bowl in which to dig by salient cue (i.e., texture, odor, and digging medium). After the tested animal learns to associate a cue with a reward, the rules are changed or reversed and the animal has to learn a new association. For compound discrimination, new stimuli and cues are presented in pairs. Other distracting dimensions may be introduced to make the test more challenging.
For monkeys, AST can be conducted using touch-screen computer-based CANTAB and WGTA methodology. The number of trials required to master each type of discrimination is used as a measure for AST. Perceptual attention, problem solving, etiologic factors, and mechanisms involved in treating depression and anxiety are the behaviors that can be assessed with AST (rats (156, 159, 160); mice (161–163); and monkeys (164–167)).
6.14.3 Go-Trial Reaction Time Task and Stop-Signal Reaction Time Task (“Go-No-Go” Tasks)
Rats and monkeys have been used in Go-Trial Reaction Time (GoRT)/Stop-Signal Reaction time (SSRT) tests. In an operant conditioning chamber with right and left levers and a pellet dispenser, a rat is trained to press first the left lever, and then the right lever to receive a food reward (GoRT). For SSRT trials, the short sound may be periodically introduced between pressing left and right levers and the rat is food rewarded if it does not press the right lever if it hears the sound.
Monkeys have been trained to either lift or not lift the lever for a reward in response to auditory stimuli of various intensities and duration or visual stimuli of various colors (168). Additionally, monkeys have been trained to make or suppress saccade eye movements for a reward in response to a peripheral visual stimulus depending on the location of the stimulus and presence or absence of a delay (169, 170). Measures for GoRT/SSRT test are percent of expected outcomes and speed of the response to stop the process. GoRT/SSRT are used to assess attention dysfunction, behavioral inhibition, and problem solving (rats (156, 166) and monkeys (168–170)).
6.15 Mental Rigidity
No appropriate behavioral tests are available.
6.16 Dementia
See the memory models above.
6.17 Personality Changes/Emotional Disorders/Behavioral Disorders
6.17.1 Open-Field Test
Rats, mice, rabbits, dogs, pigs, cats, and monkeys have been evaluated in the open-field test. The animal is placed in a circle shaped in an open field (or in a play room in the case of monkeys) and specific activities and movements are evaluated. Videotaping or monitoring by automated computer programs may be employed to facilitate the evaluation. Behaviors assessed include exploratory locomotor functions. Behavioral reactivity is evaluated by measuring spontaneous activity.
Measures of the open-field test include locomotion (number of square crossings), rearing, grooming, stereotypical behaviors (licking, biting, and head weaving), preference for particular sections, and/or fecal movements. These can all be calculated to examine behavior and anxiety (mice (92, 97, 114); rats (52, 171); rabbits (172–174); dogs (175, 176); pigs (96, 116, 177); cats (178, 179); and monkeys (180)).
6.17.2 The Elevated-Plus Maze
Rats, mice, and pigs have been used in EPM to test for the presence of anxiety-like behavior. The maze consists of four arms, intersecting at a central platform, situated above the floor. The two “open arms,” situated opposite to each other, are essentially flat planks extending into space, and the other two opposing arms are enclosed by walls. For testing, the animal is placed in the center platform of the EPM. The animal freely explores the maze for 5 min, during which behavior is recorded.
6.17.3 Light–Dark Exploration Test
Light–dark exploration test involves placing rats, mice, or pigs in a large cage (chamber for pigs) divided into dark and light compartments, which are connected by a small aperture for 5–10 min. Transitions between compartments and time spent in each compartment are noted. The test screens for the presence of anxiety-like behavior (rats (185, 186); mice (114, 187); and pigs (116)).
6.17.4 Social Interaction Test
Experimental and naïve stimulus rats (or mice) are placed at opposite corners of the arena for 5 min. Behavior may be video recorded and analyzed. Time spent in active social behavior that is initiated by the experimental animal (e.g., sniffing, climbing on, crawling under, following, grooming, wrestling, and aggressiveness) is scored. The test screens for anxiety-like behavior (rats (159, 188, 189) and mice (114, 190–192)).
6.17.5 Attentional Set-Shifting Test
See above in attention deficits.
6.17.6 Shock Probe Defensive Burying Test
A rat or mouse is placed in a familiar test chamber filled with clean fresh bedding to a depth of 3–5 cm. A shock probe able to provide a brief electric shock is mounted on a wall of the cage above the surface of the bedding. The animal typically touches the probe soon after being placed in the test chamber. Duration of immobility and attempts to cover/bury the probe with bedding after being shocked are recorded for 5–15 min. The test screens for anxiety-like behavior (rats (159, 193, 194) and mice (195, 196)).
6.17.7 Novelty-Induced Hypophagia
After a 3-day training period, singly housed rats or mice are offered a palatable food source for 10–30 min in their home cage. Test can be repeated the next day in a novel cage. Food deprivation may be necessary for rats. Measures of this test include approach latency, eating or drinking latency, time spent eating or drinking, and volume consumed in predetermined time intervals. The test is used to evaluate the presence of anxiety-like behavior (rats (197, 198) and mice (114, 199)).
< div class='tao-gold-member'>
Only gold members can continue reading. Log In or Register a > to continue
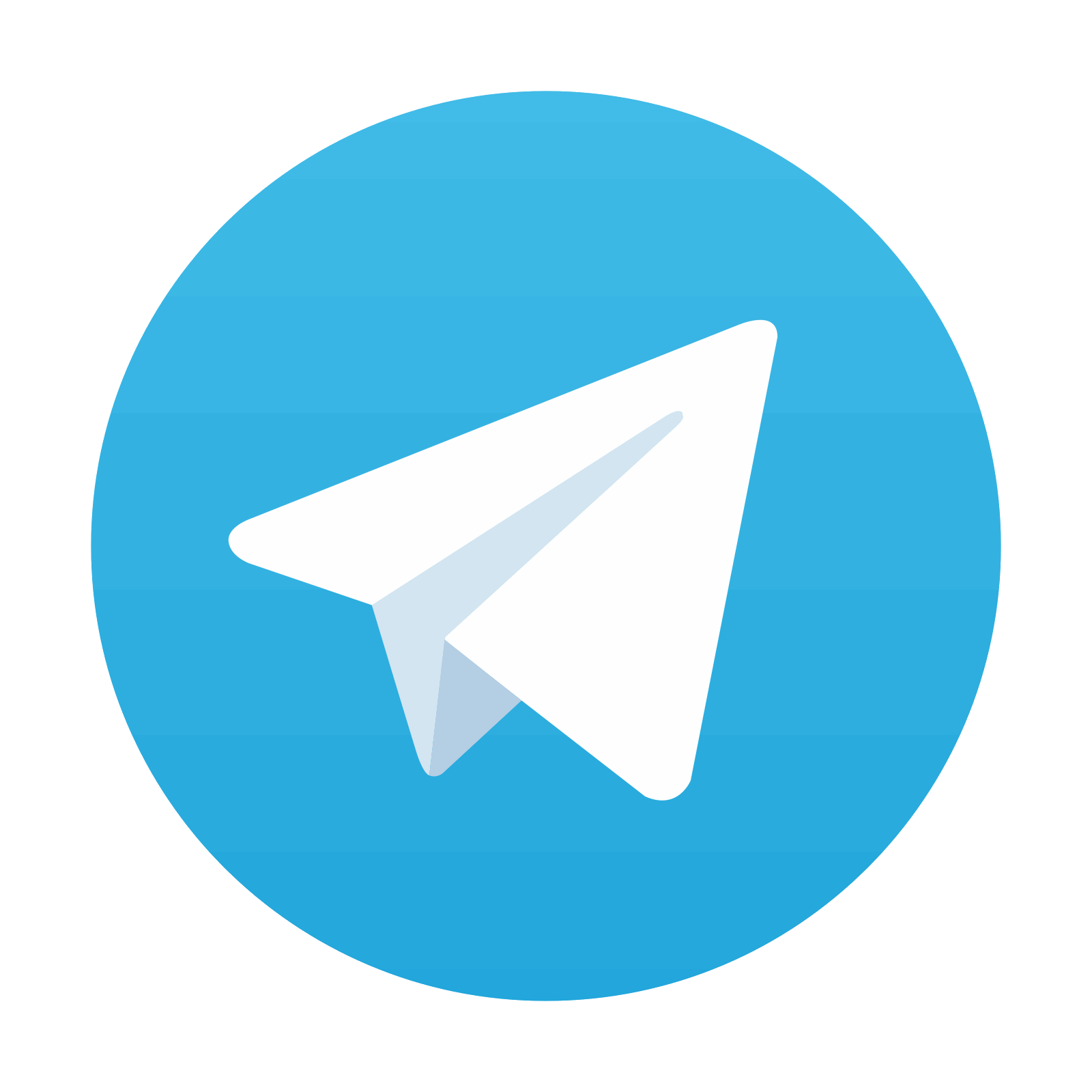
Stay updated, free articles. Join our Telegram channel

Full access? Get Clinical Tree
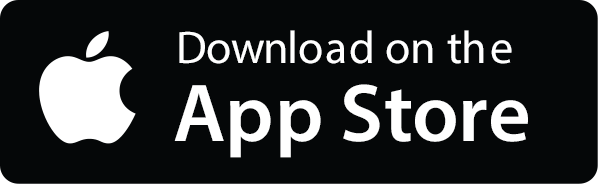
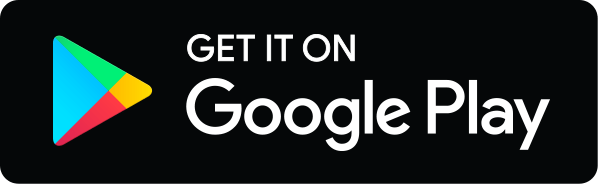