Revised from 6th edition of Veterinary Ophthalmology, Chapter 36: Neuro‐ophthalmology, by Aubrey A. Webb and Cheryl L. Cullen Neuro‐ophthalmology has emphasis on the animal’s head, the visual system, the cranial nerves, and those diseases that affect both systems. For this chapter, the material is presented based on the following: (i) basic neuroanatomy; (ii) the neuro‐ophthalmic examination; (iii) the neuro‐ophthalmic reflexes and their basic neuroanatomy; (iv) specific neuro‐ophthalmic syndromes; and (v) diseases in selected animal species that present with both ophthalmic and neurological clinical signs. Using careful observation of the patient from a distance permits one to not lose site of the forest for the trees! Upon first examining a patient from a distance, it may be clearly evident that the patient exhibits nystagmus, strabismus, or inappropriate pupil size(s). Nystagmus can be described as a rhythmic and involuntary movement of the eyes. Nystagmus may be pendular (spontaneous ocular movements without predilection for a fast or slow movement in any particular direction) and may represent abnormalities of the visual pathway, as is seen sometimes in Siamese, and other pointed‐coat colored cats. Nystagmus may also be in a particular direction and is described as being in the direction of the fast phase of the ocular movement. Spontaneous, pathological, nystagmus represents dysfunction of the vestibular system and may be either central or peripheral in origin. The central vestibular system is that portion of the nervous system located within the central nervous system (CNS) and includes the vestibular nuclei and the vestibulocerebellum (i.e., flocculonodular lobe and the fastigial nuclei of the cerebellum). The peripheral vestibular system neuroanatomically includes the bilaterally located vestibular apparatus (i.e., the utricle, saccule, and three semicircular canals within the petrous temporal bone) and the vestibular portion of the vestibulocochlear nerve. With respect to abnormalities of eye position that can be determined during a distant examination, these can be the result of vestibular disease or a disease process involving the extraocular muscles or the cranial nerves innervating the extraocular muscles (cranial nerves III, IV, and VI) (Figure 18.1). With respect to vestibular strabismus, this is most often evident when the patient’s head is held in extension. Normally, the eyes remain in a central position, though animals with vestibular disease may have a strabismus characterized by ventral strabismus ipsilateral to the side of the lesion. Figure 18.1 Drawing illustrating the insertions of the various extraocular muscles on the globe of the eye, and also indicates the innervation of the various muscles. Arrows indicate the direction of movement of the eye with contraction of the respective muscle. Anisocoria, or unequally sized pupils, is a useful finding during the distant examination. The presence of anisocoria, however, should be evaluated in conjunction with history, pupillary light reflex (PLR) and vision testing. During the distant examination, anisocoria should be observed in normal ambient light (photopic) and in dim light (scotopic) conditions. In so doing, it will become evident which eye is abnormal. In our common domesticated mammalian species, two groups of antagonistic muscles within the iris control pupil size, shape, and reaction to light. The dilator muscle consists of smooth muscle fibers distributed radially throughout the iris. The smooth iris sphincter muscle forms a ring around the pupillary margin. It has been shown that the iris sphincter and dilator muscles receive double reciprocal innervation by both the sympathetic and the parasympathetic systems. Specifically, it has been shown that cholinergic (parasympathetic) excitatory nerves causing contraction of the iridal sphincter work together with cholinergic inhibitory nerves to cause relaxation of the counteracting dilator muscles Interestingly, there are many differences among various species of animals with regard to the ciliary ganglion and the ciliary nerves arising from it. Specifically, two short ciliary nerves arise from the ciliary ganglion in the cat. The lateral one is called the malar nerve; the medial one is called the nasal nerve. The malar nerve innervates the lateral half of the iridal sphincter muscle, and the nasal nerve innervates the medial half of the sphincter. Meanwhile, the dog has five to eight short ciliary nerves. Therefore, parasympathetic denervation in the dog will cause pupillary dilation, while in the cat, lesions to either the nasal or the malar short ciliary nerves will result in sphincter hemiplegia and a D‐shaped or reverse D‐shaped pupil, respectively. Prior to our discussion of reflex and response testing in neuro‐ophthalmology, it is likely important to provide a definition of reflex and how this differs from other stimulus responses. A reflex is an almost instantaneous, transient, predictable reaction to a given stimulus. A reflex follows a typical reflex arc characterized by some sensory receptor, an afferent neuron, one or more interneurons, and an efferent neuron. Reflexes, unlike responses, are not learned and do not require input from “higher centers” in the brain. The PLR is a reflex where after the retina is stimulated by light a resultant constriction of the pupil ipsilateral (direct PLR) and contralateral (indirect/consensual PLR) to the stimulus results. The PLR is present as early as when the eyes are open after birth (10–16 days postnatally in puppies, 5–14 days postnatally in kittens), albeit PLRs may be initially sluggish and may not react similarly to the adult until maturation of the retina is complete (28 days postnatally in the puppy). The PLR is present immediately after birth in foals. The afferent pathway of the PLR runs through the cranial nerve II (optic nerve) to the optic chiasm to synapse bilaterally on neurons located in the pretectal nuclei (PTNs). These nuclei are located in the transition zone between the diencephalon and the midbrain. Axons from each PTN relay to both the left and the right parasympathetic nuclei of cranial nerve III (oculomotor nerve), though the majority of axons cross over and synapse in the contralateral parasympathetic nucleus. The parasympathetic nucleus of cranial nerve III is located in the tegmentum of the midbrain (Figure 18.2). Because neurons in each PTN send projections to the parasympathetic nuclei bilaterally, both the right and the left pupils will constrict in response to light stimulation of either eye. Since the afferent PLR pathway contains two levels of fiber crossings, first at the optic chiasm and later after exiting the PTN, in most species the direct PLR is stronger than the indirect one. More precisely, the direct PLR is stronger in those species that have more than 50% decussation at the optic chiasm because it is assumed that the same percentage of fibers that cross over at the optic chiasm cross again between the PTN and the parasympathetic nucleus at the tegmentum. In species such as birds, in which all the optic nerve fibers cross over, there is no consensual PLR. However, in some avian species, a thin interorbital septum separating the two eyes may allow light shone into one eye to pass through and stimulate the contralateral retina, resulting in a pseudo‐indirect PLR. Figure 18.2 Drawing illustrating the neuroanatomical pathway for the PLR. The swinging flashlight test is done by moving a focal light source from the tested eye to the opposite eye to check the direct and indirect light reflexes. In a clinically normal animal, the pupil that is not directly stimulated will partially constrict (due to the consensual reflex) and will then constrict further when it is directly stimulated by the swinging flashlight. If the pupil dilates during direct light stimulation instead of performing the expected constriction, the swinging flashlight test is said to be positive for the eye with the dilating pupil. The Marcus–Gunn sign is the name ascribed to the pupillary reflex observed during a positive swinging flashlight test. A positive swinging flashlight test is pathognomonic for unilateral retinal disease or unilateral prechiasmal optic nerve disease (or both). Such a lesion does not prevent the constriction resulting from the indirect PLR when the unaffected eye is stimulated. However, when the flashlight is swung to the affected eye, the loss of the indirect stimulation (combined with lack of direct stimulation) causes mydriasis. Therefore, this test is used to differentiate lesions at these locations from other neurological causes of anisocoria. The swinging flashlight test is also considered to be positive if, as the light shifts from the normal to the abnormal eye, the direct stimulus is no longer sufficient to maintain the previously evoked degree of pupillary constriction; therefore, both pupils dilate while maintaining the relative anisocoria usually present in domestic animals with optic nerve disease. A positive swinging flashlight test should be followed by a cover–uncover test done in a normal room light where the alternation of light stimulus is done by using the examiner’s hand to cover and uncover the examined eye. This test is done to eliminate the influence of scatter illumination. This reflex is characterized by bilateral, partial eyelid blink in response to a very bright light shone into each eye separate. Closure of the eye contralateral to the eye being stimulated is less obvious, and possibly absent, when compared to the ipsilateral eye. Importantly, animals having facial nerve paresis or paralysis may have a reduced or absent dazzle reflex on the ipsilateral side to the facial nerve lesion. The dazzle reflex is present as early as one to two days postnatally in puppies and kittens. Though the anatomical path of the dazzle reflex has not been elucidated in animals, evidence from the human literature suggests that it is present when the optic nerve is intact to the level of the midbrain, and particularly to reflex centers in the rostral colliculi and/or the supraoptic nuclei of the hypothalamus (Figure 18.3). The reflex also requires association fibers between these nuclei to the facial nuclei as well as intact facial nerves. The dazzle reflex is particularly useful when the pupils cannot be observed to evaluate the PLR (e.g., in cases of severe corneal edema or hyphema). Because the exact anatomical pathway of this reflex has not been described, this test should not be used as the only tool for localizing subcortical lesions in the visual pathway. The menace response is performed by making a threatening movement toward the eye being evaluated, remembering to not touch the patient or cause stimulation of the cornea or eyelashes. An appropriate response is characterized by the patient blinking, retracting their globe, and/or turning their head away from the menacing stimulus. As opposed to a simple reflex, the menace response is learned. This response takes longer to develop compared to a simple reflex like the PLR and may not be present for up to four weeks postnatally in puppies and kittens, or 8 and 14 days postnatally in lambs and goat kids, respectively. The menace response is not complete until two to three weeks. The afferent component of the central visual pathway consists of optic nerve axons projecting to the lateral geniculate nucleus (LGN) as the optic tract, after having decussated varying amounts (species dependent) at the optic chiasm (Figure 18.4). After having projected to neurons within the LGN, optic tract axons synapse on neurons within the LGN. In turn, LGN neurons project as the optic radiation to the visual cortex in the occipital lobe where an image is perceived (see Chapter 4). From the visual cortex, the visual information is transmitted through communication fibers to different regions of the cortex and finally to the primary motor cortex, which initiates the efferent component of the menace response. Figure 18.3 Drawing illustrating the postulated pathway of the dazzle reflex – a subcortical reflex blink associated with a bright light stimulus. As suggested earlier, the menace response involves cerebral cortical integration and interpretation, and therefore is not a reflex. Rather, it is a cortical response that requires the entire peripheral and central visual pathways, as well as the visual cortex and the facial nucleus of cranial nerve VII, to be intact for the response to occur. In addition to the neuroanatomical structures required to elicit a positive menace response, the menace response requires functional integrity of additional neuroanatomical structures. In all domestic species, it has been observed that diffuse cerebellar cortical degenerative lesions cause bilateral absence of the menace response without any associated visual deficits or facial nerve dysfunction. The palpebral reflex is elicited by touching the skin of the lateral and medial canthi of the eye, separately, and observing an appropriate response after each touch. An expected and appropriate response is a blink of the eyelids following a single touch. The portion of this reflex is via the ophthalmic and maxillary branches of cranial nerve V (trigeminal nerve) for medial and lateral canthus stimulation, respectively. The efferent component of the reflex is via cranial nerve VII (facial nerve). The palpebral reflex is reportedly present as early as two to four days postnatally in the puppy, and one to three days postnatally in the kitten. Palpebral reflexes are present immediately after birth in foals. Figure 18.4 The visual pathways, demonstrating how each side of the visual field is represented within the opposite occipital (visual) cortex. As the degree of binocular vision in different species decreases, a greater proportion of optic nerve fibers decussate at the optic chiasm. The corneal reflex is elicited by touching the surface of the cornea with a relatively noninjurious object (e.g., cotton‐tipped applicator or fiber from a Cochet–Bonnet esthesiometer) and then observing an expected and appropriate eyelid blink. As with the palpebral reflex, the afferent arm of this reflex is mediated via cranial nerve V (trigeminal), while the efferent arm is mediated by the cranial nerve VII (facial). The cornea is highly sensitive to touch and painful stimuli. Branches of the long ciliary nerves enter the corneal stroma in a radial manner from the sclera, episclera, and conjunctiva. In the dog, it has been demonstrated that the central cornea is the most sensitive region, followed by the nasal and temporal cornea. This pattern also exists in humans. Those regions of the cornea that are less exposed to potential injury (i.e., the dorsal and ventral corneal regions near the lid margins) are the least sensitive. In diseases such as diabetes mellitus, it has been demonstrated that corneal touch threshold (CTT) is greater in diabetic compared to nondiabetic patients. This implies that diabetes mellitus likely induces a diabetic neuropathy involving the long ciliary nerve of the cornea and these patients may be more prone to corneal injury. The vestibulo‐ocular reflex is a reflex that permits stabilization of an image on the retina during movement of the head. Without an appropriate vestibulo‐ocular reflex, images would appear blurred. The vestibulo‐ocular reflex is present for rotational or translational movement of the head and assists in stabilizing images on the retina (as exemplified in the above‐mentioned example). This reflex results in a rapidly adjusting movement of the eyes in a direction opposite that of the direction of the head movement. It is this alternation between slow and fast phases that comprises the normal physiological nystagmus that is evaluated clinically in veterinary medicine. Like the example mentioned earlier, the vestibulo‐ocular reflex is evaluated by inducing a physiological nystagmus by rotating the patient’s head in clockwise and counterclockwise directions. The expected and normal response is that the patient will slowly move its eyes, relative to the orbit, in a position opposite that of the head being turned. A fast, compensatory, movement of the eyes will occur toward the direction of the head rotation. For obstacle course testing, the patient is placed in an unfamiliar environment and allowed to move freely. Within the environment, there are randomly placed objects that the patient must navigate through during their exploratory behavior. Obstacle course testing is performed in well lit (photopic) and dimly lit (scotopic) conditions. In instances when the patient is believed to have hemianopia, having the patient navigate the obstacle course with each eye separately blindfolded can be valuable. For canine patients who are seemingly nervous, it is often times valuable to have the owner present and calling the patient’s name on the side of the room opposite that of the patient. The examiner watches for and notes any evidence of the patient bumping into or stumbling over objects, or signs of them being apprehensive while navigating. If such behaviors happen consistently, this leads the examiner to believe there is a problem with the patient’s vision. Numbers can also be assigned to the various obstacles in the maze course; therefore, the test results can be quantified. In patients that are small enough, the animal is held up, supporting its sternum and abdomen, and the patient is brought toward the edge of a flat hard surface. Importantly, the flat surface should be able to be seen by the animal. A normal response is for the animal to attempt to place its limbs on the top of the flat surface. If an animal fails to attempt to place its limb on the surface, this implies that the surface was not seen. One must keep in mind that the animal should be alert and strong enough to attempt to place its limb, however. It is important to recognize that the patient’s limb should not touch the edge of the flat surface. Animals that are blind, without any evidence of other neurological disease, will attempt to step up when their limb makes contact with the edge of the hard surface. When this happens, one is evaluating tactile placing and not visual placing. Though not traditionally considered a part of the neuro‐ophthalmic examination, quantitative testing of tear production provides relevant information concerning the function of the parasympathetic nervous system. Specifically, measurement of aqueous tear production can be done by way of the Schirmer tear test type I (see Chapter 4). The preganglionic parasympathetic neurons responsible for lacrimal secretion originate from the parasympathetic nucleus of the facial nerve (i.e., the rostral salivatory nucleus) located within the rostral portion of the medulla oblongata. The lacrimal gland’s preganglionic parasympathetic fibers run as part of the facial nerve through the facial canal of the petrous temporal bone. Within the facial canal, some of these preganglionic fibers branch off as the major (greater) petrosal nerve. The major (greater) petrosal nerve exits the temporal bone and synapses on neurons (postganglionic neurons) within the pterygopalatine ganglion. With respect to lacrimal gland innervations, postganglionic parasympathetic axons join with branches of the trigeminal nerve – the zygomatic nerve (a branch of the maxillary nerve) and the lacrimal nerve (a branch of the ophthalmic nerve). Both zygomatic and lacrimal nerves innervate the lacrimal gland and hence are important in tear production. As is hopefully apparent, some instances of diseases affecting the trigeminal nerve (e.g., trigeminal neuritis) may result in reduced tear production. Another branch of the facial nerve (branched while still within the facial canal), the chorda tympani, runs through the middle ear and also carries preganglionic parasympathetic fibers, and these fibers join the mandibular branch of the trigeminal nerve to ultimately synapse in the submandibular and sublingual ganglia that, in turn, send postganglionic fibers to their respective salivary glands. Given that preganglionic parasympathetic fibers run through the middle ear, it is possible for preganglionic fibers to become affected during diseases like otitis media, thus resulting in neurogenic keratoconjunctivitis sicca (KCS). Sensory innervation to the lacrimal gland is provided by the lacrimal nerve, the first branch of the ophthalmic nerve after it enters the orbit through the orbital fissure. The nerve runs to the lateral part of the orbit and gives branches to the lacrimal gland, to other deeper structures, and then to the skin of the lateral canthus of the eye. In some instances, arriving at a neuroanatomical diagnosis, that is, identifying where along the neuroanatomical pathway a lesion is located, one must provocatively test the nervous system pharmacologically. Here, we describe the pharmacological approach to differentiating between pre‐ and postganglionic lesions of the parasympathetic and sympathetic nervous systems, respectively. The basis for pharmacological testing, whether parasympathetic or sympathetic, relies upon (i) the ability of neurotransmitter to be released from the postganglionic neuron or (ii) the phenomenon of denervation hypersensitivity. In order to differentiate between pre‐ and postganglionic parasympathetic lesions, the following series of tests can be attempted. The drugs used include the following: In order to differentiate between pre‐ and postganglionic sympathetic lesions, the following series of tests can be attempted. The drugs used include the following: Localization of the lesion is, as previously stated, one of the most important steps in reaching a diagnosis (Table 18.1). Without an accurate neuroanatomical diagnosis, one will be unable to formulate an appropriate differential diagnostic list or diagnostic plan. Behavior can be defined as the motor and autonomic manifestation of physiological processes. Accepting this, and understanding that various components of the nervous system are responsible for various behaviors, though of course, the nervous system is very “plastic” and not “hard‐wired.” Arriving at a neuroanatomical diagnosis is key prior to formulating a list of differential diagnoses. The list of Braund’s clinical syndromes includes the cerebral syndrome, dicephalic syndrome, unilateral vestibular disease, midbrain, and others (Boxes 18.1–18.3 and Table 18.2). Horner’s syndrome is that cluster of clinical signs referable to the loss of ocular sympathetic innervation (Figure 18.5), and occurs most frequently in the dog. Remember that the sympathetic nervous pathway to the eye essentially involves presympathetic neurons from the brain to the sympathetic preganglionic neurons located in the intermediolateral gray matter of spinal cord segments T1–T3, and finally postganglionic sympathetic neurons located in the cranial cervical ganglion. Postganglionic sympathetic fibers innervate the ciliary body, iris dilator and iris sphincter muscles (keep in mind reciprocal iris innervations), the smooth muscles of the periorbita, and Müller’s muscles of the upper and lower eyelids. Normal sympathetic tone in these smooth muscles keeps the globe slightly protruded, the palpebral fissure opened, the third eyelid retracted, and the pupil partially dilated. When there is a disease process affecting the sympathetic innervation, however, the following signs are found with variable expression: Cavernous sinus syndrome is that group of clinical signs that refers to disease processes involving the cavernous sinus (see Box 18.3). The cavernous sinus is a venous sinus located bilaterally on the floor of the middle cranial vault and extending from the orbital fissure to the petro‐occipital canal. Each cavernous sinus is connected rostrally and caudally by rostral and caudal intercavernous sinuses, respectively. Neuro‐ophthalmically important structures that are in close proximity to the cavernous sinus include cranial nerves III, IV, and VI, and the ophthalmic and maxillary branches of cranial nerve V. In addition, postganglionic sympathetic axons are also found near the cavernous sinus. It is this close association with the cavernous sinus, and the constellation of clinical signs that are referable to these nerves’ dysfunction, that this syndrome gets its name. It should be recognized also that cavernous sinus syndrome can be either unilateral or bilateral, albeit bilateral cavernous sinus syndrome is rarer. The clinical signs found in cavernous sinus syndrome typically include the following: Table 18.1 Neuroanatomical location of various nuclei and ganglia important in neuro‐ophthalmology.
18
Neuro‐ophthalmology
Neuro‐ophthalmic Examination
The Distant Examination
Nystagmus
Strabismus
Anisocoria and Pupil Size
Reflex and Response Testing
Pupillary Light Reflex
Swinging Flashlight Test
Dazzle Reflex (Photic Blink Reflex)
Menace Response
Palpebral Reflex
Corneal Reflex
Vestibulo‐ocular Reflex and Physiological Nystagmus
Vision Testing
Obstacle Course (Maze Testing)
Visual Placing
Schirmer Tear Testing
Pharmacological Testing
Parasympathetic Lesions
Sympathetic Lesions
Neuroanatomical Lesion Localization
Braund’s Syndromes
Horner’s Syndrome
Cavernous Sinus Syndrome
Cranial nerve or brain nuclei
Origination
Entry/exit to/from the cranial vault
Termination
Function
Cranial nerve II (optic nerve)
Retinal ganglion cells in retina
Optic canal
Lateral geniculate nucleus
Rostral colliculi
Thalamic nuclei
Note: variable decussation at the optic chiasm (species dependent)
Vision
Afferent arm of PLR
Afferent arm of menace response
Afferent arm of dazzle reflex
Motor nucleus of cranial nerve III (oculomotor nucleus)
Tegmentum (floor) of the midbrain
Orbital fissure
Medial, dorsal, and ventral recti muscles
Ocular movements
Caudal to PTN
Ventral oblique muscle
Rostral to trochlear nucleus
Levator palpebrae superioris muscle
All ipsilateral to the nucleus
Parasympathetic nucleus of cranial nerve III (Edinger–Westphal nucleus)
Tegmentum (floor) of the midbrain
Orbital fissure
Ciliary ganglion
Efferent arm of PLR – pupillary constriction via constriction of ciliary muscle of iris (sphincter pupillae muscle)
Caudal to PTN
Rostral to trochlear nucleus
Motor nucleus of cranial nerve IV (trochlear nerve)
Caudal mesencephalon at level of caudal colliculi
Orbital fissure
Dorsal oblique muscle on side opposite the location of the nucleus
Ocular movement
Caudal to oculomotor nuclei
Motor nucleus of cranial nerve VI (abducens nerve)
Rostral medulla oblongata
Orbital fissure
Lateral rectus and retractor bulbi muscles ipsilateral to the nucleus
Ocular movement and retraction
Motor nucleus of cranial nerve V (trigeminal nerve)
Pons at level of middle and rostral cerebellar peduncles
Canal for the trigeminal nerve in petrous temporal bone and then exits with the mandibular branch of the nerve via the oval foramen
Masseter, temporal, pterygoid, rostral digastricus, and mylohyoid muscles
Motor to muscles of mastication
Sensory ganglion of cranial nerve V (trigeminal nerve)
Neuron cell bodies of the mandibular, ophthalmic, and maxillary branches are located in trigeminal ganglion located in the canal for the trigeminal nerve in the petrous temporal bone
Receiving sensory information from the head
Canal for the trigeminal nerve in the petrous temporal bone
Various somatic efferent nuclei within the brainstem
Spinal tract of the trigeminal nerve
Mandibular branch – sensory to cheek, mandibular teeth, and tongue
Ophthalmic branch – sensory to orbit, medial part of upper eyelid, and dorsum of the rostral nose
Maxillary branch – sensory to most of face, cheek, lateral aspect of nose, and lateral aspect of eyelids
Motor nucleus of cranial nerve VII (facial nerve)
Rostral medulla oblongata
Stylomastoid foramen via the facial canal in the petrous temporal bone
Muscles of facial expression
Caudal portion of the digastricus muscle
Motor to muscles innervated
Parasympathetic nucleus of cranial nerve VII
Rostral medulla oblongata
Preganglionic traverse in the facial canal and exit with the maxillary and mandibular branches of the trigeminal nerve
Preganglionic: synapse in pterygopalatine ganglion
Postganglionic: innervate lacrimal, palatine, and nasal glands
Lacrimation
Nasal wetting
Cranial nerve VIII (vestibular portion)
Pons and medulla oblongata
Internal acoustic meatus
Rostral, medial, lateral, and caudal vestibular nuclei
Minority to fastigial nucleus and flocculonodular lobe of the cerebellum
Coordinate eye, neck, trunk, and limb position with position and movements of the head
Maintain balance
Lateral geniculate nucleus
Diencephalon
Not applicable
Visual cortex (lateral, caudal, and medial aspects of the occipital lobe)
Project visual information from optic tracts to visual cortex
Pretectal nucleus
Mesencephalon
Not applicable
Bilateral projections to parasympathetic nuclei of cranial nerve III (Edinger– Westphal nuclei)
Maintenance of the PLR
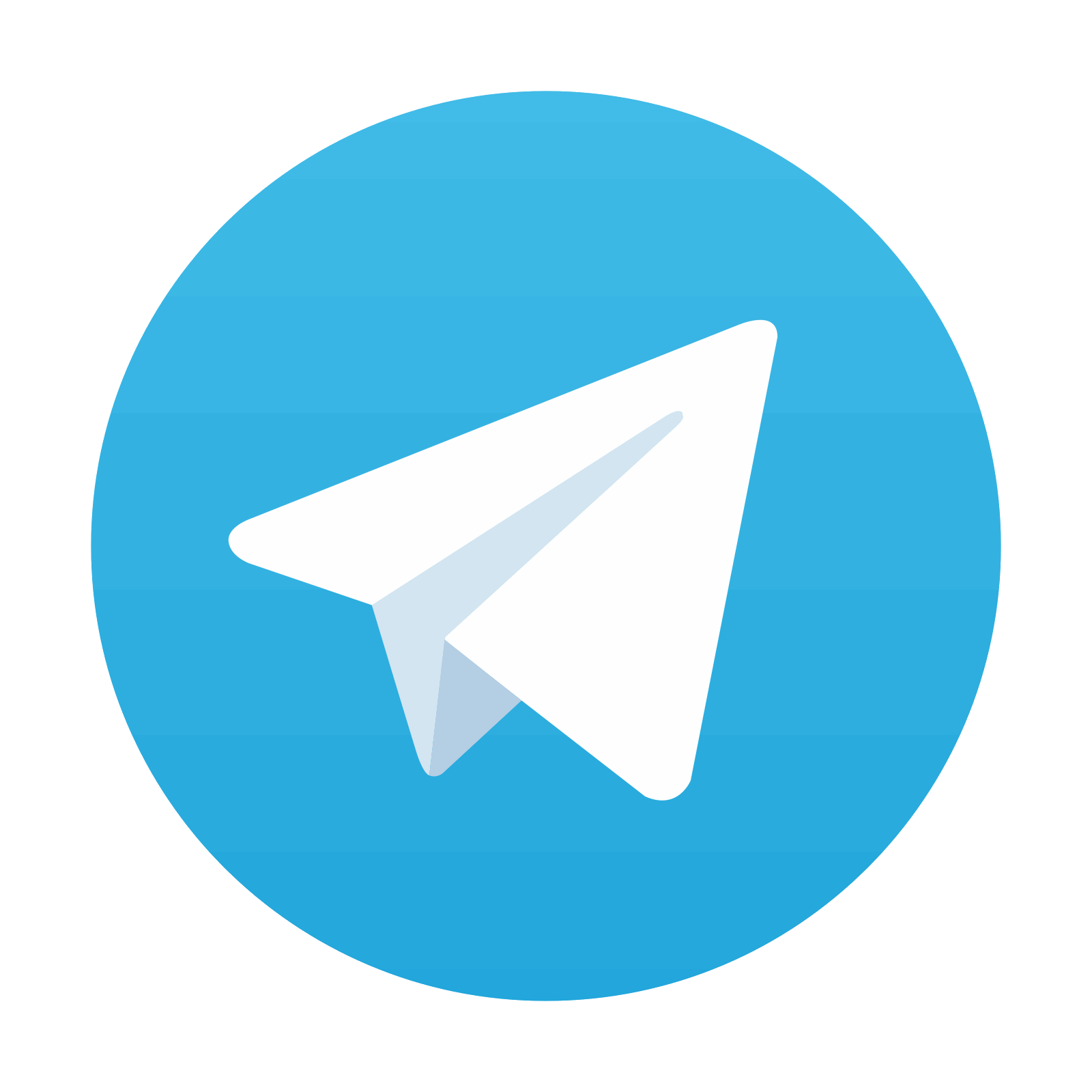
Stay updated, free articles. Join our Telegram channel

Full access? Get Clinical Tree
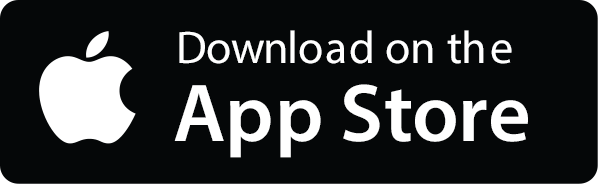
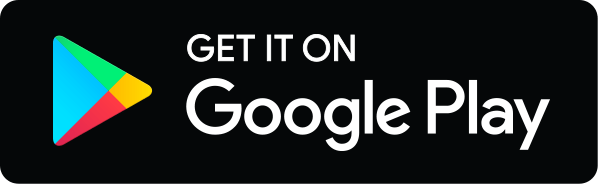