CHAPTER 7 DNA evidence suggests that over the long evolutionary history of the nematodes, the transition from a free- living lifestyle to animal parasitism may have taken place on several separate occasions. Consequently, the parasitic nematodes are a very diverse group. Chapter 6 described one cluster of related superfamilies (the ‘bursate’ nematodes). These are characterised by the presence on the male tail of a prominent cuticular structure, the bursa. The story now moves on to the nematode superfamilies of veterinary importance that do not display this feature – the ‘nonbursate’ nematodes. Most are only distantly related to one another and so their biological characteristics are correspondingly disparate. This chapter also includes a brief description of the Acanthocephala (‘thorny-headed worms’) which are unrelated to the Nematoda but are included in the vague colloquial term ‘roundworm’. Reference is also made to another worm-like group that does not fit comfortably into the structure of this book: the leeches, which are more closely related to earthworms than to nematodes. There are six nonbursate superfamilies of particular interest (see Figure 7.1), although others may be encountered less commonly in veterinary practice. The Spiruroidea and Filarioidea are related but other nonbursate superfamilies are so different from each other that each is best considered as a separate entity. Figure 7.1 Overview of the nonbursate nematode superfamilies. This superfamily is a transitional group in which the majority of species are free-living but some have started to exploit opportunities provided by parasitism. Most members of the group spend their whole life-cycle in inanimate habitats such as soil or decaying vegetation. Some are parasitic on or in plants (the potato eel-worm, for example). A few are basically free-livers but will parasitize animal tissues if a chance arises (i.e. they are facultative parasites). Pelodera and similar genera can invade the skin of dogs or other animals lying on damp bedding and may cause dermatitis. The usually saprophytic Halicephalobus is particularly dangerous as it can penetrate through the skin of immunocompromised animals to breed prolifically in kidney, CNS and other tissues, forming tumour-like lesions. It is an occasional cause of ataxia in horses and, rarely, a human infection. There is just one genus that has adopted parasitism as an essential (obligatory) component of its life-cycle: Strongyloides. This must have proved a successful strategy as there are many Strongyloides species affecting a wide range of hosts. Humans and domesticated animals each have their own Strongyloides species (e.g. S. westeri in horses, S. papillosus in ruminants, S. ransomi in pigs etc.). Cross-infection between hosts is uncommon, although some strains of a dog species, S. stercoralis, are thought to have zoonotic potential. Strongyloides adults collected from their animal host are small (< 1 cm) and thread-like resembling trichostrongyloids (see Figure 6.20). Closer inspection, however, will fail to detect the presence of any bursate males – in fact, there are no male worms in the animal host, only females (as will be explained below). If confirmation of identity is needed, the Strongyloides pharynx, at almost a third of the total body length, is much longer than the trichostrongyloid equivalent. The eggs are thin-shelled and half the size of a typical strongyle egg (see Figure 7.2). Each already contains a first-stage larva when it is passed in faeces. Figure 7.2 Strongyloides egg. Help box 7.1 The life-cycle of Strongyloides is unique in that it involves a parasitic cycle and a free-living cycle. In some species these alternate; in others external stimuli may influence the route taken by successive generations. Remarkably, free-living adults do not resemble their parasitic siblings, being much stouter and having a differently shaped pharynx (presumably reflecting different food sources and feeding mechanisms). Parasitic adults live close to the mucosa of the small intestine while free-living adults inhabit soiled bedding or other humid, nutrition-rich environments. The life-cycles of the various Strongyloides species vary in detail but are broadly similar. The equine species S. westeri is used as an example (see Figure 7.3): Figure 7.3 Life-cycle of Strongyloides westeri: a – larvated egg; b – L3 (infective or free-living); Parasitic cycle: c – infective L3 infects mare or foal by skin penetration; d – transmammary infection of foal; e – larvated eggs passed in faeces; Free-living cycle: f – free-living L3 develops to adult; g – larvated eggs produced (details in text which uses same lettering as shown above). Redrawn after Jacobs, 1986 with permission of Elsevier; larval and adult stages after Grove, 1989 with permission of Taylor and Francis. (In some species, e.g. S. stercoralis in dogs, the egg hatches in the intestine and the L1 is passed in faeces.) Patent infections are commonest in very young animals and may be associated with diarrhoea or dysentery, particularly in piglets and puppies. Often, however, infections are symptomless even though faecal egg-counts are high. Species such as S. stercoralis, with eggs that hatch before leaving the host, can give rise to persistent infections, especially if the animal is immunocompromised. In this case, larvae that develop to the infective stage within the host digestive tract penetrate the intestinal wall or perianal skin. This is known as ‘autoinfection’. When animal owners report seeing a ‘roundworm’, they are most often referring to an ascarid. This is understandable as ascarids are big fleshy worms (see Figure 7.4) that are very obvious if present in faeces or vomit. On a smaller scale, the larvae of one group of ascarids, the anisakids, are sometimes found in herring and other fish. These are not only unsightly but occasionally cause human health problems (see Section 9.3.1). Figure 7.4 Ascarid worms (Parascaris from a horse). Reproduced with permission of M.K. Nielsen. Adult ascarids live in the lumen of the small intestine. They are typically between 5 and 40 cm long (depending on species, sex and stage of development) and pinkish-white or ivory-white in colour. Close inspection of the head reveals three prominent lips around the mouth (see Figure 7.5). Figure 7.5 En face (head-on) view of the head of an ascarid showing three large lips. The females are very prolific, each producing hundreds of thousands of eggs per day. The eggs are almost spherical, so they appear round when seen under the microscope (see Figure 7.6). At 85–100 μm, they are just a little bigger than a standard strongyle egg (Practical tip box 6.2). They have a thick protective shell. This is smooth-surfaced, but some species have an additional albuminoid layer which gives the surface a rough or sculptured appearance. Figure 7.6 Ascarid eggs: left – a smooth-shelled species (Toxascaris; unembyonated); right – a rough-shelled species (Toxocara; embryonated). Ascarid eggs are unembryonated when passed into the environment. If conditions are favourable, an infective larva will develop inside each. The egg does not hatch until swallowed by a host. Extra information box 7.1 The ascarids tend to have complicated life-cycles. An understanding of these is necessary in order to appreciate the epidemiology of associated disease and to design sensible control programmes. The following paragraphs describe the life-cycles of three ascarids in order of ascending complexity. The first is the poultry ascarid, Ascaridia, which is ‘nonmigratory’ (i.e. the larvae do not migrate around the body). It is one of the simplest of the ascarid life-cycles (see Figure 7.7): Figure 7.7 Life-cycle of a nonmigratory ascarid, Ascaridia: a – eggs passed in faeces; b – infective larva develops inside egg; c – earthworm transport host; d – bird swallows embryonated egg or earthworm; e – larva develops to adult worm in intestine (details in text). Eggs redrawn after Ackert, 1931 from Mozgovoi, 1953 with permission of Nauka. Extra information box 7.2 After entering the final host, the larvae of some ascarid species undergo an extensive journey through the body, called hepatotracheal migration, before establishing in the small intestine. An example is the equine roundworm, Parascaris (see Figure 7.8): Figure 7.8 Life-cycle of a migratory ascarid, Parascaris: a – egg passed in faeces and embryonates on ground; b – infective egg is swallowed and hatched larva enters hepatic portal veins; c – larva carried to liver then via blood to heart and lungs; d – larva ascends trachea; e – larva is swallowed and develops to adult worm in small intestine (details in text). Egg redrawn after Antipin, 1940 from Mozgovoi, 1953 with permission of Nauka. The preparasitic life-cycle of Parascaris is very similar to that of Ascaridia (see Figure 7.7) except that the egg is brown with an irregular albuminous coating. The most complex ascarid life-cycles are those that involve ‘somatic larvae’ as well as migrating larvae. The difference between migrating and somatic larvae is that the former are motile and continue to develop while travelling around the body of their host. Somatic larvae, on the other hand, come to rest in host tissues such as liver, kidney, CNS or musculature and go into a state of arrested development. They do not grow and they do not start to develop until they are reactivated. They are nevertheless metabolically highly active, producing large amounts of excretory/secretory (ES) substances that modulate host immune responses as well as a thick mucin that spreads over the cuticle to protect against cellular and antibody attack. This coating is left behind when the larva moves on. These activities provoke the formation of small granulomatous nodules. An example of an ascarid that utilises somatic infection as an important component of its life-cycle is Toxocara canis, one of the ascarids found in dogs and other canids (see Figure 7.9). The preparasitic development of T. canis is similar to that described for Parascaris (see Figure 7.8) as is the subsequent hepatotracheal migration that occurs in puppies. Somatic infection occurs when embryonated eggs are swallowed by a paratenic host or a mature dog. The consequences of this are outlined in the following paragraphs. Figure 7.9 Life-cycle of Toxocara canis (illustrating somatic infection): a – eggs embryonate on ground; b – embryonated eggs infective for pups, adult dogs and paratenic hosts; c – somatic larvae accumulate in bitch; d – pups infected by activated somatic larvae in utero and while suckling; e – adult worms establish in the intestine of pups (and occasionally older dogs) and eggs passed in faeces. In any warm-blooded animal that is not a dog, fox or other canid, T. canis eggs hatch and larvae migrate via the liver and heart to the lungs, as happens in the initial stages of hepatotracheal migration (see Figure 7.8). In the lungs, however, they stay in the blood-stream and are returned to the heart to be distributed around the whole body via the general circulation. They settle out in various somatic tissues and become arrested in their development (see Figure 7.10). They now wait for the paratenic host in which they reside to be eaten by a dog, fox, wolf, dingo, etc. If and when this happens, the larvae are released within the digestive tract of their new host and can resume their normal developmental behaviour. Figure 7.10 A Toxocara larva in a brain section from an infected mouse. Reproduced with permission of C. Strube. The category ‘any warm-blooded animal that is not a dog, fox or other canid’ includes humans and somatic infections can establish in people just as in any other paratentic host. T. canis is therefore a potentially zoonotic pathogen (see Section 9.3.2). When infection takes place in dogs older than about two months of age, only a very small proportion (if any) of T. canis larvae undergo hepatotracheal migration. Most become somatic larvae in the same way they would if they were in a paratenic host. In this case, however, they are waiting for a reactivation stimulus. This happens only after the host becomes pregnant. The greatest numbers of somatic larvae are activated in late pregnancy, some during lactation, but others remain dormant until subsequent pregnancies. Activated larvae migrate to the placenta or mammary glands and are thereby transferred to foetuses in utero (‘transplacental transmission’) or to neonates via colostrum or milk (‘transmammary transmission’). Once in the foetus or puppy, the larvae undergo hepatotracheal migration. The first adult worms reach maturity in the small intestine in the third week of independent life. The horizontal transmission of ascarid infections within host populations is usually via the faecal-oral route, although paratenic hosts can be an important supplementary pathway for species that parasitize predators (e.g. the feline species Toxocara cati). The time taken for eggs passed in faeces to reach the infective (embryonated) stage varies from weeks to months depending on temperature and humidity. Once introduced onto a property, ascarid infection can be very persistent. This is because embryonated ascarid eggs can remain viable for 5 years or more in protected surroundings (e.g. in moist soil or in humid cracks and crevasses). Furthermore, eggs with an albuminoid coat are sticky and will adhere to skin, structural surfaces, boots etc. Consequently, casual cleaning routines will be ineffective, especially as the thick eggshell protects the larva within from most disinfectants. The eggs will, however, succumb to desiccation and direct sunlight. Ascarid infections induce a protective immunity, although this generally takes many months to develop. Consequently, the proportion of host animals harbouring patent intestinal infection and the number of worms present declines with age. Nevertheless, the fecundity of the worms is so great that just a small number of older carrier animals can ensure perpetual contamination of premises. Immunity is, however, ineffective against somatic larvae. Thus, somatic infection can persist into adulthood enabling vertical (transplacental and/or transmammary) transmission to take place. This adds an extra dimension to the epidemiology of those ascarid species that include somatic larvae in their life-cycle. The consequences of this will be described in the Toxocara section below. Different stages of the ascarid life-cycle provoke different pathological effects. The adults of most species are so big that they can cause catastrophic blockage and even perforation of the intestine if present in large enough numbers. Smaller numbers may provoke diarrhoea and sometimes vomiting. The presence of just a few worms can adversely affect the growth-rate of the host at an early age, but is more likely to be asymptomatic in older animals. Larval migration is marked by parasitic pathways which attract intense eosinophilic infiltration. Passage through the liver induces fibroblastic responses appearing as white spots. Large numbers of larvae in the lung may induce allergic reactions giving rise to nasal discharge and other respiratory signs. Damage to the lungs may exacerbate preexisting pulmonary bacterial or viral infection or provide a gateway for secondary infection. Finally, the presence of ascarids may interfere with postvaccination immunity to some other pathogens. Ascaris suum is the most important macroparasite of pigs (swine) worldwide (see Table 7.1). A very closely related species, A. lumbricoides, is a significant human health problem wherever public hygiene standards fall below ideal. These two ascarid species are mostly restricted to their own respective hosts but cross-infection can occur. If embryonated Ascaris eggs are swallowed by animals other than their natural final host (for example, lambs grazing a former pig pasture), larvae will migrate to the liver and perhaps to the lungs, but will not establish in the intestine. Table 7.1 Some important ascarids * Probably of little or no zoonotic importance. Adult female Ascaris grow to 40 cm long, but Parascaris equorum, in horses and other equids, is even bigger – up to 50 cm. P. equorum is ubiquitous and can be troublesome in younger horses. The life-cycle of Ascaris is very similar to that of Parascaris (see Figure 7.8), although the prepatent period is a little shorter. Hepatotracheal migration occurs within the final host, but there is no transplacental or transmammary transmission. Each phase of the parasitic life-cycle can result in economic or welfare problems in pigs, including all the direct and indirect effects described in the ‘General pathogenicity’ section above. Even small numbers of A. suum larvae passing through the liver can trigger a fibroblastic response (see Figure 7.11). The lesions are roughly spherical, except where they meet the liver surface, and they sometimes have a haemorrhagic centre. They vary from several millimetres to a couple of centimetres in diameter with white strands radiating out between adjacent liver lobules. The pathological term for these ‘milk-spots’ is ‘chronic focal interstitial hepatitis’. The lesions resolve in about six weeks leaving a very small nodule. Figure 7.11 Milk-spot lesions in pig liver. Reproduced with permission of A. Daugschies. There is a complex relationship between immunity and ‘milk-spots’. On exposure to A. suum, pigs develop a protective immunity over a period of months. Initially, migrating larvae pass through the liver and lungs, and it is not until they arrive back in the intestine that they succumb to immune attack. Thus, it is not an unusual occurrence in an abattoir to find ‘milk-spots’ in the livers of younger pigs even though they have no adult worms in the intestine. As pigs become more solidly immune, larvae are killed before they reach the liver, and ‘milk-spots’ are therefore observed less frequently in slaughtered sows. Sows nevertheless play an important role in the epidemiology of ‘milk-spot’ livers. Despite their immunity, a small proportion of sows do carry a small number of adult worms in their alimentary tract. As female A. suum are such prolific egg-layers, the farrowing house can quickly become contaminated. As a result, the susceptible piglets acquire infection, carry it with them to the fattening house and perpetuate the problem. As adult horses rarely harbour patent infection, the transmission of P. equorum is generally from one year’s foals to the next via contaminated pastures. Foals are often infected in their first month of life but eggs do not appear in the faeces for a further 10–12 weeks (i.e. the prepatent period). Light infestations are asymptomatic but perpetuate the transmission cycle by contaminating the pasture anew. In heavier infections, lung damage by migrating larvae may provoke coughing and nasal discharge, while intestinal worms contribute to unthriftiness or weight-loss. As the worms are so large, obstructive impaction or even perforation of the small intestine can occur in extreme cases with severe or fatal consequences. Toxocara can be severely detrimental to the welfare of young animals. It is also responsible for human disease. There are three major species: T. canis in dogs and other canids, T. cati in cats and other felids, and T. vitulorum in buffalo and cattle. The dog and cat ascarids are cosmopolitan, but the bovine species is found mostly in warmer climates. Although each species can, as a general rule, establish as an adult worm only in its own final host, all are indiscriminate in their choice of paratenic hosts. The pathogenic role of T. canis in human tissues is well documented (see Section 9.3.2), but the extent to which T. cati and T. vitulorum contribute to human disease is ill-defined. T. canis and T. cati adults grow to about 10–18 cm in length (see Figure 7.12) but T. vitulorum is longer (up to 30 cm). The deep brown eggs are described as subglobular (i.e. not quite spherical). The sticky albuminous layer is sculptured so that the surface of the egg appears rough under light microscopy (see Figure 7.13). Figure 7.12 Toxocara canis in the small intestine of a puppy. Figure 7.13 Toxocara egg. If left untreated, almost all puppies will harbour T. canis to a greater or lesser extent. Most of the worms are acquired prenatally, but these are later supplemented by the ingestion of larvae passed with colostrum and milk. Further infection can take place by swallowing embryonated eggs from the environment. Feral canids are exposed to yet another source of infection once they start to take solid food – somatic larvae in the tissues of prey animals such as mice. The prepatent period of T. canis is 4–5 weeks, but eggs start to appear in the faeces of puppies after the second week of life. This apparent anomaly occurs because activation of somatic larvae in the bitch happens during the last three weeks of gestation. Prenatally acquired larvae have therefore started their hepatotracheal migration in the pups prior to whelping. Infected pups excrete (literally) millions of T. canis eggs in their faeces during the suckling period. Help box 7.2 From the age of about six weeks, pups start to lose their intestinal T. canis population spontaneously, a phenomenon well-known to all dog breeders (see Figure 9.20). During this period, a progressively greater proportion of ingested larvae become arrested in somatic tissues rather than taking the hepatotracheal route. Thus, only a minority of dogs over 4–6 months of age harbour patent infection and they are likely to have no more than a few adult worms. Their T. canis egg-output is correspondingly low but sufficient nonetheless to ensure widespread environmental contamination. Higher egg-counts are, however, encountered in suckling bitches as these often experience a temporary increase in their intestinal worm burden. Adult foxes, too, tend to carry greater numbers of intestinal T. canis than most domestic dogs. As with many other ascarid infections, a few worms do no discernible harm, but greater numbers can stunt growth and cause serious, and sometimes fatal, disease (see Section 9.2.1). T. cati infects cats and other felidae. Its biology is in many respects similar to that of T. canis but there are only three routes of transmission – transmammary, embryonated eggs and infected paratenic hosts. As there is no prenatal infection, kittens are first infected during suckling and eggs will not appear until the end of the prepatent period (i.e. when the kittens are around 8 weeks old). Help box 7.3
Nematoda (‘roundworms’) part 2: nonbursate nematodes and anthelmintics
7.1 Nonbursate nematodes
7.1.1 Nonbursate superfamilies
7.1.2 Rhabditoidea
Strongyloides
Parasitic phase of life-cycle:
Free-living phase of life-cycle
Pathogenicity
7.1.3 Ascaridoidea (ascarids)
General characteristics
General life-cycle
Hepatotracheal migration
Somatic infection
T. canis in paratenic hosts
T. canis in older dogs
General epidemiology
General pathogenicity
Important ascarids
Ascaris and Parascaris
Genus
Host
Hepatotracheal migration?
Paratenic host?
Vertical transmission?
Zoonotic importance
More information in Section:
Ascaris
Pig, human
Yes
No
No
▼
8.3.1
Parascaris
Equidae
Yes
No
No
9.1.1
Toxocara
Dogs, cats, cattle
Yes
Yes
Yes
▼▼▼
9.2.1; 9.3.2
Toxascaris
Dogs, cats
No
Yes
No
*
Ascaridia
Poultry
No
No
No
8.4.1
Heterakis
Poultry
No
No
No
8.4.1
Ascaris suum
Parascaris equorum
Toxocara
Toxocara canis
Toxocara cati
Stay updated, free articles. Join our Telegram channel

Full access? Get Clinical Tree
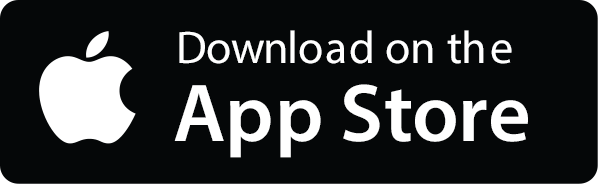
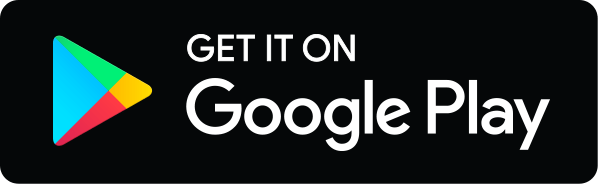