Fig. 7.1
Posterior facial vein graft interpositioned to the femoral artery, showing (a) completed anastomoses (interrupted 11-0 sutures, 8 per anastomosis), with forceps adjacent to small ligated branch in middle of graft and (b) graft with flow immediately after completing the anastomoses (flow is from left to right). The dilated vein is approximately 0.6 mm in diameter, while the femoral artery is 0.2 mm in diameter
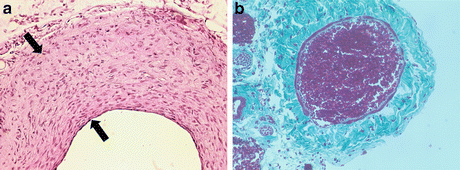
Fig. 7.2
(a) H&E cross section of proximal portion of vein graft (4-week harvest), showing neointimal extent between black arrows. (b) Masson stain of cross-sectioned posterior facial vein prior to harvest from the neck (blood filling lumen to hold it open); note very thin medial layer (one-cell layer thick)
7.2.5 Patch Graft Models
A model of vein oval patch graft to an artery has been described by two groups, using autologous transplantation of a piece of jugular vein to replace a portion of resected arterial wall. Although not a true vein bypass-analogous graft, this model provides some insight into the response of a vein wall in an arterial environment. Shi and associates [20] used a single incision in the ventral neck to access both the jugular vein for obtaining the graft and exposure of the carotid artery for placement. Sakaguchi and colleagues [21] transferred the same graft to the abdominal aorta. Both groups described using 11-0 suture in a single, continuous running stitch for engraftment into an arteriotomy/side window.
7.3 Technical Comparisons
The various combinations of donor vein and recipient artery selection and the method of graft anastomosis are limited, though this may play a profound role in the subsequent response designed to mimic clinical vein grafting (Table 7.1). Zhou et al. [15] and Zhang et al. [16] both use the same vessels, grafting the IVC into the carotid artery but with very different anastomotic techniques. Diao et al. [17] and Salzberg et al. [18] used a suturing technique that differed from that of Zhang et al.[15], placing interrupted stitches in end-to-end fashion rather than continuous running stitches in end-to-side configuration and adding variations in graft/recipient combinations. In the model developed in my lab [11], perhaps the smallest vein used, the posterior facial vein is grafted into the smallest of arteries in these models – the common femoral artery – using interrupted end-to-end suturing. The patch graft models use more common vessels but deviate from true interpositional vein grafting. Though none of these models replicate clinical vein graft-to-artery scenarios, the femoral artery model can be argued to share more features of lower extremity artery bypass surgery, involving a muscular-type artery that in fact matches a clinical site for these procedures.
Table 7.1
List of published murine vein graft models and their relative characteristics, merits, and expected/estimated outcomes
Model | Ring-coupled | End-to-side suturing | End-to-end suturing | End-to-end suturing | End-to-end suturing | Patch graft | Patch graft |
---|---|---|---|---|---|---|---|
Originator | Zou et al. [15] | Zhang et al. [16] | Diao et al. [17] | Salzberg et al. [18] | Cooley [11] | Shi et al. [20] | Sakaguchi et al. [21] |
Recipient artery | Carotid | Carotid | Abdominal aorta or carotid | Abdominal aorta | Femoral artery | Carotid | Abdominal aorta |
Donor vein | Inferior vena cava | Inferior vena cava | External jugular vein | Inferior vena cava | Posterior facial vein | External jugular vein | External jugular vein |
Anastomosis | Mechanical ring | Continuous 11-0 suture | Interrupted 11-0 suture | Interrupted 11-0 suture | Interrupted 11-0 suture | Continuous 11-0 suture | Continuous 11-0 suture |
Procedure time (graft harvest + interpositioning) | Total = 60 min | Total = 20 + 90 min | 68 min aortic clamp time | 30–50 min aortic clamp time | Total = 5 + 45–55 min | 50 min | Total = 40 min |
Success rate (patency) | 86–90% | >95 % with experience | 89 % | (83 %) | 70–80% with experience | 87 % | 92 % |
Vein: artery diametric ratio | Not determined | 2.3:1 | 1.9:1 (carotid) 1.4:1 (aorta) | Not determined | ~3:1 | Not applicable | Not applicable |
Neointimal thickness | (~220 μm) | 91 μm | Not determined | 110 μm | ~103 μm | Not determined | Not determined |
Lumen area reduction | Not determined | (~16 %) | (~20 %) | Not determined | ~78 % (proximal end) | Not determined | ~65 % |
The suture-based techniques [11, 15–18] offer the most clinical analogy, though the ring-coupled approach of Zou and colleagues [15] is the simplest to perform. An inherent weakness of this model is the abrupt diametric transitions from the arterial ends where they enter the vein through the inner diameter of the ring, creating a 90° geometric jump to the larger diameter of the outer edge of the ring. This deviation from the other models, which attempt to create a smoother transitional interface from artery to vein and back to artery, may lead to potentially non-pathophysiologic results, at least in terms of paralleling clinical vein graft responses.
Another consideration is the handling and treatment of the vein graft, from harvest to arterial flow. Dilating or otherwise stretching the vein may match what some clinical surgeons do, but there has been an effort over the past 30 or more years to minimize handling and related sources of iatrogenic trauma to the vein – the so-called no-touch technique [22–24]. The ring-coupled method describes mechanically stretching the IVC over the ring for its placement. Though the rest of the published models lack details on vein handling, in the case of my model, the handling is nearly no-touch, with only the needle passage having a direct trauma to the vein wall; the vein is isolated from the neck without touching or flushing it, allowing incubation in a heparinized solution to gently clear the blood without clotting and using the same solution at the recipient site to float the vein open for identifying the lumen and to facilitate stitch placement. Whether the other models use a similar approach would be valuable to learn.
The technique of experimental vein grafting for model selection must take two other aspects under consideration: time for the procedure (i.e., throughput) and success rate (acute graft patency). The published accounts for the procedural times differ in their start and stop points (Table 7.1), though most models describe times that appear to lead to total times of 40–70 min, with perhaps longer times for the end-to-side model of Zhang and colleagues [16]. These times are clearly dependent upon operator experience and microsurgical proficiency. In the case of my model, the initial effort took 2.5 h, but rapidly fell to under 1.5 h, and approximately 1 h with further experience; this time includes all steps, from graft harvest in a donor to final recipient site closure.
Another consideration is the acute success rate – graft patency following the procedure (Table 7.1). Most models report greater than 85 %, approaching 100 % for some [16] as experience is gained. The model I developed is an exception, with success rates slightly lower at 70–80 %, even with extensive experience. There thus may be trade-offs between speed of completing the procedure and successful outcome.
The major outcome measure for which these models were primarily designed is neointimal inward remodeling of the graft wall – the major complication of clinical vein grafting. As mentioned previously, this neointimal growth is often mistakenly attributed to the influence from simple transference of venous tissue to arterial flow/shear and pressure. Many studies in larger animal models of vein graft neointimal development have found relatively little net wall thickening, with very few models showing true stenotic lesion formation. The primary induction of excessive neointimal growth occurs at sites of perturbed or oscillatory flow and not at high-shear, laminar flow regions. Thus, in evaluating murine vein grafts, analysis should be directed at models and sites within models where such perturbed flow can be expected.
The transitions from the artery to the vein and back from the vein to the artery are the most likely sites of predicted flow alterations. Indeed, some of the reported models have shown such differential responses, with greater neointimal thickening in the graft adjacent to the anastomotic junctures [11, 16]. Another criterion for predicting such altered flow is the diametric ratio of the vein to the artery (Table 7.1). This is seen to be approximately 2:1 for the IVC grafts placed into the carotid artery, with a lower ratio for placement into the aorta. The posterior facial vein grafted into the femoral artery has an even greater ratio of ~3:1, which is further supported by the strong difference in neointimal response in the proximal and distal portions of the graft in comparison to the mid-graft regions.
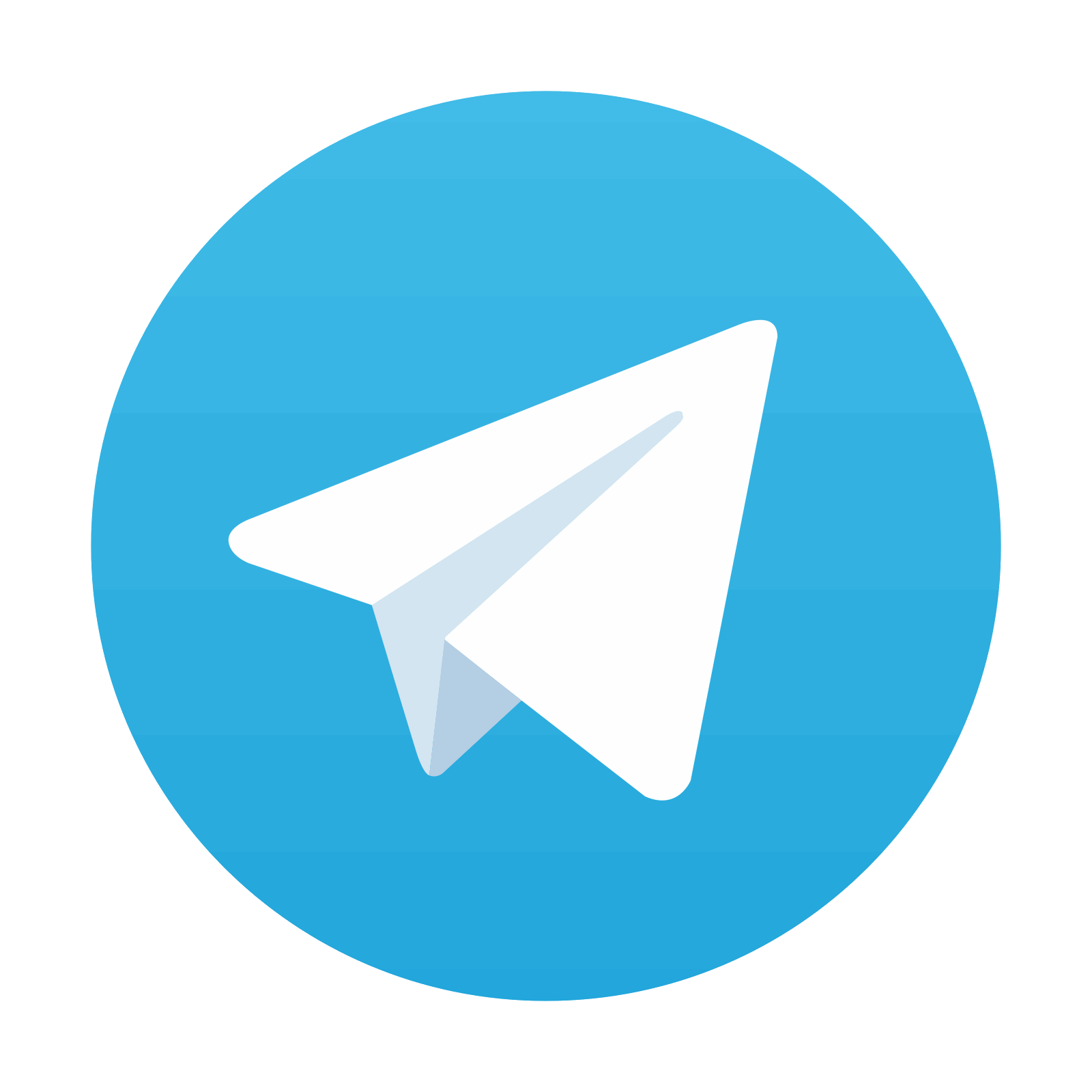
Stay updated, free articles. Join our Telegram channel

Full access? Get Clinical Tree
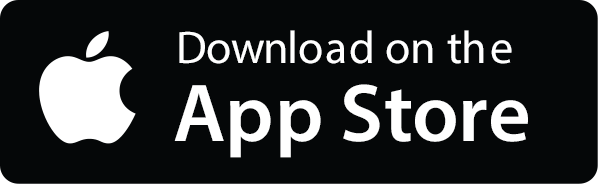
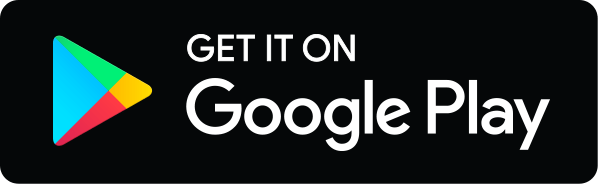