17 Morbilliviruses in Sea Mammals Universitat Autònoma de Barcelona, Barcelona, Spain In the last three decades new morbilliviruses have emerged as a serious threat to marine mammal populations. Recognition of morbilliviruses as infectious agents in marine mammals dates from the late 1980s, when two nearby simultaneous distemper epizootics occurred in the Baikal Lake, affecting Baikal seals (Phoca sibirica) (Grachev et al., 1989; Osterhaus et al., 1989a, 1989b; Visser et al., 1990), and in the North and Baltic Seas, affecting harbour seals (Phoca vitulina) and grey seals (Halichoerus grypus). Canine distemper virus (CDV) caused the epizootic at Baikal Lake, whereas the seals in northern Europe were affected by a previously unknown morbillivirus, different from CDV, called phocine distemper virus (PDV) (Cosby et al., 1988; Kennedy et al., 1988a; Visser et al., 1990). The next known morbillivirus epizootic in marine mammals affected Mediterranean striped dolphins (Stenella coeruleoalba) and common dolphins in the Black Sea (Delphinus delphis ponticus) from 1990 to 1994 (Domingo et al., 1990; Birkun et al., 1999). This mass mortality was caused by a new member of the morbillivirus genus, called dolphin morbillivirus (DMV) (Domingo et al., 1990). A previous epizootic affecting bottlenose dolphins (Tursiops truncatus) on the east coast of the USA in 1987–1988 (Geraci, 1989) was re-evaluated, and it was concluded that a morbillivirus closely related to DMV had a primary role in the die-off (Lipscomb et al., 1994, 1996). The identification of DMV drew attention to a morbillivirus previously found in six harbour porpoises (Phocoena phocoena) in the Irish Sea in 1988 (Kennedy et al., 1988b, 1991). The virus was characterized and proposed as a new species of the genus morbillivirus, called porpoise morbillivirus (PMV), closely related to DMV, distinct from other terrestrial morbilliviruses and also from PDV (McCullough et al., 1991; Trudgett et al., 1991). A lethal morbilliviral infection in long-finned pilot whales (Globicephala melas) was reported on the New Jersey coast (Taubenberger et al., 2000), caused by another phylogenetically related but distinct morbillivirus, termed pilot whale morbillivirus (PWMV). In addition to epizootic outbreaks, sporadic cases of systemic morbilliviral disease have been reported in both seals and cetaceans (Daoust et al., 1993; Duignan et al., 1993; Jauniaux et al., 2000; Yang et al., 2006; Stone et al., 2011; Mazzariol et al., 2012), and unusual forms of localized central nervous system (CNS) infection have been described in the striped dolphin (Domingo et al., 1995; Soto et al., 2011a). The new morbilliviruses in marine mammals, considered by some to be four different species (Taubenberger et al., 2000; Van de Bildt et al., 2005), are classified today by the International Committee on Taxonomy of Viruses (ICTV, 2011) in two species: cetacean morbillivirus (CeMV, including the strains DMV, PMV and PWMV) and PDV. This terminology will be used throughout this chapter. Morbilliviruses are distributed in marine mammals worldwide, and the number of infected pinniped and cetacean species is high (Barrett et al., 1995; Kennedy, 1998; Van Bressem et al., 1999, 2001). Like other morbilliviruses in terrestrial mammals, they are capable of causing epizootics, with high morbidity and mortality, when entering into a naïve population (Kennedy, 1998). Mass mortalities in marine mammals where morbilliviruses have been demonstrated as the causative agent are shown in Table 17.1. Although the first documented epizootic was recorded in 1987 (Lipscomb et al., 1994), serological evidence of morbillivirus infection in marine mammal populations dates from 1973 (Henderson et al., 1992). In addition, single lethal cases or discrete mortality events related to morbillivirus infection have been detected worldwide, for example, in seals from Canada and the USA (Daoust et al., 1993; Duignan et al., 1993; Earle et al., 2011), in harbour porpoises (Phocoena phocoena) from England and Scotland (Kennedy et al., 1992) and in bottlenose dolphins from Australia (Stone et al., 2011). Collectively, these data point to the enzootic circulation of morbilliviruses in many pinniped and cetacean species (Van Bressem et al., 2001; Kennedy, 1998). Susceptibility to morbillivirus in different marine mammal species, population density and animal migratory movements may influence circulation of morbillivirus in pinniped and cetacean populations (Duignan et al., 1995; Kennedy, 1998). Thus, harp seals (Phoca groenlandica) are likely a PDV reservoir, and their southward migratory movements may have precipitated the 1988 epizootic in harbour and grey seals in northern Europe (Dietz et al., 1989; Markussen and Have, 1992). Similarly, pilot whales have been incriminated in the introduction into the Mediterranean Sea of CeMV, responsible for two mass mortalities in Mediterranean dolphins (Kennedy, 1998; Fernández et al., 2008). The second CeMV epizootic of 2006 extended from the strait of Gibraltar eastward through the Mediterranean Sea (Raga et al., 2008). In this event the mortality in dolphins was preceded by deaths in pilot whales (Fernández et al., 2008). The pilot whale population resident in the strait of Gibraltar, which is subjected to intensive monitoring and photo identification, suffered between summer 2006 and summer 2007. In this period, the survival rate was reduced by 21.2%, which increased mortality from 51–52 deaths to 77–78 deaths. This increase in mortality was attributed to CeMV (Gauffier, 2008). The re-emergence of morbillivirus epizootics in marine mammals has been described on several occasions, for example in Mediterranean dolphins and northern European seals. Factors leading to re-emergence of mass mortalities in previously affected areas are poorly understood. A build-up of susceptible individuals is probably required for the emergence of a new epizootic. It is conceivable that juvenile animals are affected once they lose maternal immunity (Ross et al., 1994). The available data regarding northern seal populations points to this possibility (Jensen et al., 2002). A similar situation has been observed for CeMV in the Mediterranean Sea after the 1990–1994 epizootic. A review of archive material from necropsies of striped dolphins performed during the inter-epizootic period did not find evidence of CeMV-induced lesions or antigens, thus supporting the view that CeMV had apparently not circulated in that population (Soto et al., 2011b). Also, in limited serological studies, the proportion of susceptible individuals in the population had increased (Van Bressem et al., 2001). Table 17.1. Morbillivirus epizootics in marine mammals. Distemper in seals can also be caused by CDV (Grachev et al., 1989; Lyons et al., 1993; Mamaev et al., 1996). The epizootic in Baikal seals in 1987 stressed for the first time the pathogenic potential of CDV in seals (Grachev et al., 1989; Osterhaus et al., 1989a), demonstrating that CDV may also be a threat for other seal species. On the other hand, PDV has been shown experimentally to be infectious for dogs (Jäger et al., 1990), mink (Blixenkrone-Møller et al., 1989) and ferrets (Nielsen et al., 2008). Whether PDV may also infect other terrestrial carnivore species is unknown. The natural host range for CeMV is mainly restricted to cetaceans, although CeMV strains have been detected in seals (Van de Bildt et al., 2000, 2001; Mazzasiol et al., 2013). Subsequent to both CeMV epizootics in the Mediterranean Sea, unusual cases of CeMV infection affecting only the CNS have been observed in striped dolphins (Domingo et al., 1995; Soto et al., 2011a; Di Guardo et al., 2013). In these cases, infection with CeMV was restricted to the CNS, as showed by immunohistochemistry (IHC) and reverse-transcriptase-polymerase chain reaction (RT-PCR) (Soto et al., 2011a; Di Guardo et al., 2013). It has been suggested that this CNS-localized form in striped dolphins could be similar to a human disease, subacute sclerosing panencephalitis (SSEP) (Domingo et al., 1995; Soto et al., 2011a), which is caused by a replicative defective measles virus (MeV) (Connolly et al., 1967; Garg, 2008). Therefore, these forms would appear to be epidemiologically irrelevant regarding transmission of CeMV in the population. Nevertheless, observed impact on the population through direct mortality could be high in the two or three years after the epizootic wave (Soto et al., 2011a). Experimental data on pathogenesis of marine morbillivirus infection are scarce, and pathogenesis is mainly deduced from natural disease investigation and from similarities with morbillivirus infections in terrestrial mammals (Kennedy, 1998). Transmission of CeMV and PDV in nature probably occurs by the oronasal route, through direct contact with oculonasal and oral secretions of infected animals, or through airborne droplets at short distance. However, there is no experimental evidence supporting this hypothesis. It is also unknown if infected animals excrete morbillivirus in faeces and urine, as occurs in dogs with distemper. In harbour seals experimentally infected with PDV by the nasal route, no virus could be isolated from faecal samples of affected individuals, although viral isolation also failed from tissue homogenates of seals dying from infection between 11 and 16 days post-infection (Harder et al., 1992). PDV antigen was detected in intestinal lymphoid tissue and in colonic epithelial cells, supporting the possibility of viral secretion in faeces (Harder et al., 1992; Pohlmeyer et al., 1993). Pinnipeds spend part of their biological cycle in terrestrial environments, highly aggregated, and faeces and urine from infected animals might be relevant for transmission. Other routes of infection, such as trans-placental, lactation or venereal routes, could be postulated but there is no direct evidence supporting these possibilities. As in dogs (Beineke et al., 2009), the tonsils (pharyngeal in seals and laryngeal in dolphins) in marine mammals may constitute a primary site of replication for morbillivirus (Harder et al., 1992; Soto et al., 2011b). From the primary point of entry the virus may disseminate systemically to other organs. The distribution of virus and lesions follows the same regular pattern in natural cases of disease in the different marine mammal species. Lung, lymphoid organs and the CNS are the main targets (Kennedy et al., 1989; Domingo et al., 1992), regularly showing cellular damage with viral inclusions and viral antigen. The consequence of lymphoid tissue damage is immunosuppression, which facilitates secondary opportunistic infections by fungal agents, Toxoplasma gondii and herpesvirus in dolphins (Domingo et al., 1992; Lipscomb et al., 1994, 1996; Schulman et al., 1997; Bellière et al., 2010; Mazzariol et al., 2012; Soto et al., 2012), and by herpesvirus and bacterial in infections in seals (Osterhaus and Vedder, 1988; Gulland et al., 1997; Kennedy, 1998). The course of PDV infection has been monitored in experimentally infected seals. In one experiment harbour seals were intra-nasally infected with PDV, and clinical signs, viraemia and immunological response were recorded (Harder et al., 1992). All the infected animals showed fever starting between day 3 and 6 post-inoculation. Afterwards watery diarrhoea, respiratory disease and central nervous signs were observed. Six out of ten animals died within 11–16 days post-infection, despite supportive treatment. The other four seals recovered. Viraemia was cell associated and PDV could be isolated from buffy coat cells starting from day 5 post-infection and lasting until day 17 post-infection or until death of the animal. PDV antigen could be detected in several tissues, including the respiratory and gastrointestinal tracts, CNS and lymphoid organs. PDV neutralizing antibodies were first detected on day 7 post-inoculation, with exponential increase afterwards, reaching a plateau within the fourth week post-infection. The duration of immunity after natural or experimental PDV infection is unknown, but immune seals are protected from clinical disease when experimentally challenged with a moderate dose of PDV (Harder et al., 1990). Pups born from infected mothers show maternally derived antibodies, which decrease progressively with time and are no longer detectable at approximately 10 weeks of age (Harder et al., 1992, 1993). Other predisposing or triggering factors in marine mammal morbillivirus epizootics have been a matter of intense debate for decades in the scientific community (Van Bressem et al., 2009). Several studies have focused on the influence of xenobiotics on the immune system of cetaceans (Beineke et al., 2010). Some authors have claimed that pollutants, such as polychlorinated biphenyls (PCBs), and starvation were important co-factors in seal and cetacean morbillivirus epizootics (Aguilar and Borrell, 1994; Olsson et al., 1994; de Swart et al., 1995; Kajiwara et al., 2008). In dolphins it has been suggested that PCBs lead to depressed immunocompetence and increased susceptibility to viral infection (Aguilar and Borrell, 1994; Osterhaus et al., 1995). Morbilliviruses are primary pathogenic agents and are able to cause epizootics in their respective hosts without requiring predisposing factors (Appel et al., 1981; O’Shea, 2000). In an experimental study, seals fed with PCB did not show any difference in the outcome and course of PDV infection compared with non-PCB-fed animals (Harder et al., 1992). However, the PCB load of these animals appeared to be lower than the PCB load of free-ranging seals. In humans and dogs, MeV and CDV are known to cause chronic latent infections, termed respectively subacute sclerosing panencephalitis (SSEP) and old dog encephalitis (ODE) (Axthelm and Krakowka, 1998; Garg, 2008; Headley et al., 2009). In these rare diseases, after an initial systemic infection, virus latently infecting the CNS reactivates several years later, causing a localized, progressive and fatal neurologic disease. It has been suggested that similar chronic latent infections occur in striped dolphins after CeMV epizootics (Domingo et al., 1995; Soto et al., 2011a). In these cases morbillivirus infection was limited to the brain, with absence of lesions and viral antigen in extraneural sites, such as respiratory and lymphoid systems. In SSPE, mutations in the P, M, F and H genes that affect the functionality of viral proteins have been determined (Cattaneo et al., 1989; Wong et al., 1989), rendering MeV unable to form infective virions. Whether similar changes also occur in CeMV in these striped dolphins remains to be determined. Clinical signs of morbilliviral infection have been recorded mostly in pinnipeds, but little information is available in cetaceans. Clinical signs of seals affected by PDV are similar to those in dogs infected by CDV (Osterhaus and Vedder, 1988). Weight loss, lethargy, fever, serous or purulent oculonasal secretion, conjunctivitis and dyspnoea have been reported. Other signs also observed are diarrhoea, abortion, subcutaneous emphysema (which increase buoyancy, impairing diving) and increased tolerance to human presence. Neurological signs such as tremor and abnormal posture have been also described (Kennedy, 1998; Philippa et al., 2009). Dermatologic changes, such as alopecia and crusting, have occasionally been reported (Lipscomb et al., 2001). Affected cetaceans may show poor body condition, lethargy, tremors and abnormal respiratory and cardiac rate. Some animals present disorientation and abnormal swimming with circling (Piza, 1991; M. Domingo, Barcelona-Spain, 2008, personal communication). Dolphins stranded alive with morbilliviral infection died after several hours or a few days with no response to clinical treatments (Piza, 1991). Morbillivirus-induced lesions are very similar in pinnipeds and cetaceans, and occur principally in lungs, lymphoid tissues and the CNS. Poor body condition and an absence of food in stomach are frequently found in affected animals. In lungs, lesions consist of multiple areas of dark-coloured lung tissue, alternating with inflated, pale zones, which are often only evident in severe cases. Normal lungs in cetaceans do not fully collapse when the chest is opened, due to the cartilaginous reinforcement of airways and the presence of smooth muscle sphincters up to the respiratory bronchioles. Nevertheless, most authors describe absence of pulmonary collapse in morbilliviral disease, both in seals and cetaceans. The lung may show areas of consolidation due to suppurative bronchopneumonia, commonly found in seals but not frequently found in cetaceans. Interstitial (interlobular and subpleural) pulmonary emphysema is frequently found in seals, which may extend to the mediastinum and neck fascia and subcutis. Parasitic pneumonia with nodular lesions is usually present. Pulmonary lymph nodes may appear enlarged and oedematous. Erosive stomatitis has been described mainly in cetaceans (Kennedy et al., 1989, 1991; Domingo et al., 1992; Kennedy, 1998; Fernández et al., 2008; Rijks et al., 2008; Philippa et al., 2009). Dermatitis, with alopecia and crusting, has been described in a hooded seal (Cystophora crystata) and a harp seal (Phoca groenlandica) (Lipscomb et al., 2001). The load of epizoic parasites was increased during epizootics in dolphins due to the reduced mobility caused by the disease (Aznar et al., 2005). Macroscopic lesions associated with opportunistic fungal infections have been consistently observed in cetaceans during CeMV epizootics. Large focal haemorrhagic-necrotizing mycotic lesions were observed in the lungs and brain (Domingo et al., 1992; Kennedy et al., 1992; Lipscomb et al., 1994; Soto et al., 2011b). Toxoplasma behaves as an opportunistic condition in the western Mediterranean striped dolphin population, with subclinical infection in around 11% of the individuals (Cabezón et al., 2004), and reactivation during CeMV epizootics. Lesions due to Toxoplasma gondii appear as small necrotic foci in lymph nodes and lung, but may not be always macroscopically evident (Domingo et al., 1992; Soto et al., 2011b). Morbillivirus infection in marine mammals usually causes pneumonia, encephalitis and lymphoid necrosis and depletion (Kennedy et al., 1989, 1991; Domingo et al., 1992; Taubenberger et al., 2000; Fernández et al., 2008). This triad of lesions in the lung, brain and lymphoid organs are also highly compatible with morbillivirus infection in terrestrial mammals (Appel et al., 1981; Caswell and Williams, 2007). Affected animals usually present bronchiolo-interstitial pneumonia, characterized by infection and necrosis of the bronchiolar and alveolar epithelial cells, and exudation of mononuclear leukocytes in the alveolar septa and alveolar spaces. Necrosis of epithelial cells is followed by regeneration of type II pneumocytes and re-epithelization. Multinucleate syncytial cells are frequently observed in bronchiolar and alveolar lumens (see Plate 1). Nuclear and cytoplasmic eosinophilic inclusions are easily recognized in epithelial cells and syncytia (Kennedy et al., 1989, 1991; Baker, 1992; Domingo et al., 1992; Kennedy, 1998; Rijks et al., 2008). Secondary bacterial suppurative bronchopneumonia is a frequent consequence of immunosuppression in seals (Kennedy, 1998). Generalized lymphocytolysis and lymphoid depletion are prominent findings in seals and cetaceans infected by morbillivirus. Lymph nodes, spleen, thymus and Peyer’s Patches are usually affected. In dolphins, the laryngeal tonsil (Cowan and Smith, 1999) also shows similar lesions (Soto et al., 2011b). There is severe loss of lymphocytes and syncytial cells may replace lymphoid tissue. In pinnipeds, unlike in cetaceans, syncytial formation is a rare finding in these tissues. Cell debris and distended blood vessels are visible. Nuclear and cytoplasmic eosinophilic inclusion bodies can also be observed in syncytial or mononuclear cells (Baker, 1992; Domingo et al., 1992; Kennedy, 1998; Jauniaux et al., 2000; Rijks et al., 2008). Entry of the virus into the CNS induces a non-suppurative meningoencephalitis characterized by neuronal degeneration and necrosis, with perivascular mononuclear inflammatory infiltrates. Neuronal damage is associated with gliosis and formation of neuronophagic nodules. Viral inclusions may be seen in neurons and glial cells. In dolphins, syncytia may be found also in the CNS in low number, but they are rare in seals. Severe patchy demyelinization may be seen in seals (Kennedy et al., 1989, 1991; Domingo et al., 1992; Kennedy, 1998; Fernández et al., 2008; Rijks et al., 2008). Inflammation and syncytial cells with viral inclusions can be found sporadically in other organs, such as mammary epithelium, hepatic biliary ducts, renal pelvis, urinary bladder, pancreatic ducts and gastrointestinal tract (Domingo et al., 1992; Kennedy, 1998; Jauniaux et al., 2000). Skin lesions have been associated with morbillivirus infection in seals, with hyper-keratosis, epidermal hyperplasia with formation of syncytial cells, inflammatory infiltrates and viral inclusions (Lipscomb et al., 2001). Histopathological lesions in the different affected organs are usually associated with the presence of viral antigen that can be revealed by immunohisto-chemical staining. Syncytial cells, mono-nuclear leukocytes, pneumocytes (see Plate 2), neurons, glial cells, ependymal cells, keratinocytes and epithelial cells in other organs, such as mammary gland, male reproductive tract and the epithelium overlying the laryngeal tonsil (see Plate 3), show cytoplasmic and nuclear immunostaining (Domingo et al., 1992; Kennedy et al., 1989, 1991; Kennedy, 1998; Fernández et al., 2008). In dolphins, secondary fungal infections affecting the brain and lungs appear as haemorrhagic-necrotizing pneumonia and encephalitis, with growth of fungal hyphae in the tissues and in vessels, causing vasculitis and thrombosis (Domingo et al., 1992; Lipscomb et al., 1994, 1996; Kennedy et al., 1992; Soto et al., 2011b). The usual pattern of microscopic findings associated with toxoplasmosis in CeMV-infected Mediterranean striped dolphins include granulomatous necrotizing lymphadenitis, adrenalitis, pneumonia and encephalitis. Toxoplasma cysts can be visualized in affected tissues, and toxoplasma antigen can be demonstrated by immunohistochemistry in inflammatory lesions (Domingo et al., 1992; Soto et al., 2011b). Opportunistic herpesvirus infection in dolphins can cause lymphoid depletion, interstitial pneumonia and nonsuppurative encephalitis, with the formation of syncytial cells and nuclear viral inclusions in different tissues (Soto et al., 2012). In harbour seals with herpesvirus infection, hepatic and adrenocortical necrosis, interstitial pneumonia and non-suppurative encephalitis can be observed (Borst et al., 1996; Gulland et al., 1997). Cases of CeMV-infection restricted to the CNS lack the characteristic microscopic lesions of morbilliviral disease occurring in lungs and lymphoid tissues. Therefore, diagnosis is based on recognition of inflammatory brain lesions, and detection of CeMV-antigen or nucleic acid in the CNS (Domingo et al., 1995; Soto et al., 2011a). Lesions in the brain are often chronic in nature, with perivascular mononuclear inflammatory infiltrates, spongiotic changes in affected cerebral cortex and malacia in some cases. Distribution of lesions and CeMV antigen is variable, being in general most prominent in the cerebral cortex than in the thalamus or brain stem. The cerebellum was usually unaffected. In some cases, CeMV-antigen may be widespread in brain regions in neuronal bodies and processes, showing no or very low inflammatory changes. Diagnosis of morbilliviral disease in marine mammals is based on detection of specific microscopic lesions in target organs (see above), demonstration of morbilliviral antigen and/or viral nucleic acid in tissues and virus isolation (Kennedy et al., 1989; Domingo et al., 1992; Barrett et al., 1993; Nielsen et al., 2008). Monoclonal antibodies (MoAbs) have been raised by several groups for identification of morbillivirus in cell culture and tissues. For example, MoAb panels against CDV were applied to tissues of infected animals at the beginning of the North Sea seal epizootic, and absence of reaction with some of the individual MoAbs pointed clearly to a new aetiological agent causing the die-off (Örvell et al., 1990; Rima et al., 1992). Similarly, a MoAb panel against PDV was used in dolphin tissues infected with CeMV, showing a different staining pattern than the one in seals infected with PDV. In addition, antibodies, monoclonal as well as polyclonal, have been applied for diagnosis in immunohistochemical techniques to demonstrate morbillivirus antigens in tissues of affected animals (Kennedy et al., 1988a, 1989; Likhoshway et al., 1989; Domingo et al., 1992; Lipscomb et al., 1994). Antibodies against CDV, PDV or MeV may show cross reactivity with other viruses within the genus. For example, PDV has been successfully detected using anti-CDV or anti-MeV antibodies (Kennedy et al., 1988, 1989), and CDV and PDV have been successfully detected using anti-PDV antibodies (Stanton et al., 2004). CeMV has been demonstrated with anti-PDV or anti-CDV antibodies (Domingo et al., 1992; Soto et al., 2011b), but PDV and CDV could not be detected using anti-CeMV (Stanton et al., 2004). In our experience, sensitivity of immunohistochemistry is high enough for routine diagnosis of CeMV field cases. Taking into account the known gradient of mRNA transcription of morbilliviruses, decreasing from the N-protein mRNA to the L-protein mRNA (Ray and Fujinami, 1987), immunohisto-chemical techniques based on the viral N protein may show advantages over techniques based on other viral proteins that are transcribed later. Several protocols for molecular diagnosis of morbillivirus infection in marine mammals have been developed. The most frequently used techniques, with targeted gene, amplicon size, viral specificity and reference, are shown in Table 17.2. Most of these techniques use primers designed on conserved regions of the targeted genes. Classic protocols are based on conventional RT-PCR, but quantitative (qRT-PCR) techniques have also been developed for both PDV and CeMV. Table 17.2. RT-PCR techniques for detection of morbilliviruses in marine mammals.
17.1 Introduction
17.2 Epidemiology
17.3 Pathogenesis
17.4 Clinical Signs
17.5 Pathology
17.5.1 Macroscopic lesions
17.5.2 Microscopic lesions
17.6 Diagnosis
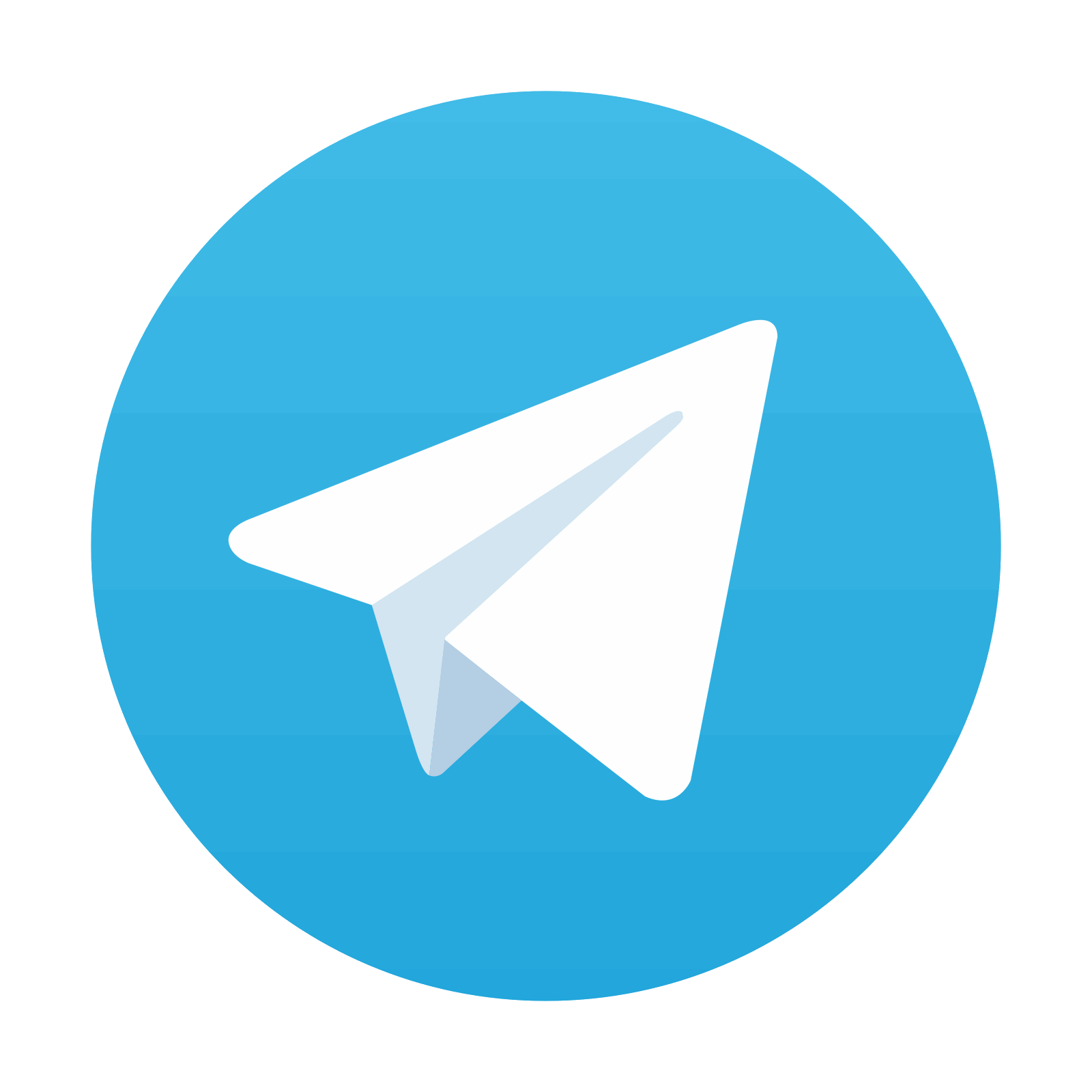
Stay updated, free articles. Join our Telegram channel

Full access? Get Clinical Tree
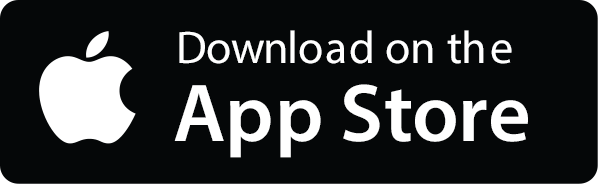
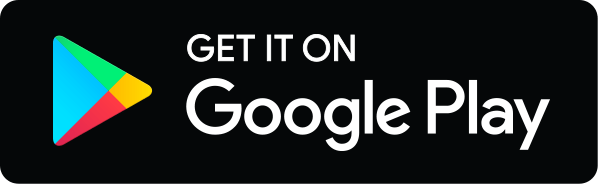