13 Leticia Mattos de Souza Dantas and Sharon L. Crowell‐Davis University of Georgia, Athens, GA, USA Monoamine oxidase (MAO) is an enzyme of the outer mitochondrial membrane that occurs in a variety of tissues, including the heart, the liver, kidneys, the spleen, platelets, the peripheral nervous system, and the central nervous system (CNS) (Obata et al. 1987). In the CNS, MAO, primarily MAO‐B, catabolizes the oxidative deamination of catecholamines, including dopamine, norepinephrine, epinephrine, β‐phenylethylamine (2‐phenylethylamine), and serotonin. In the intestinal tract and liver, MAO, primarily MAO‐A, is also important in catabolizing exogenous amines, for example, tyramine, derived from various foods and drugs. The name is not entirely accurate since MAO enzymes can also deaminate long‐chain diamines (Gerlach et al. 1993). MAO inhibitors prevent the action of MAO‐A, MAO‐B, or both. Drugs in this category, while classified according to their action of inhibiting MAO, also have a variety of other actions, many of which enhance the activity of catecholamines. For example, l‐deprenyl, in addition to inhibiting MAO activity, inhibits presynaptic catecholamine receptors, inhibits the uptake of catecholamines, induces the release of catecholamines from their intraneuronal stores, and stimulates action potential‐transmitter release coupling (Knoll et al. 1996). Thus, they should not be considered solely as drugs that just have a simple and specific activity. They are also likely to exhibit substantially different actions on different species because there are species differences in the ratios of MAO‐A to MAO‐B, both overall and in given organ systems. For example, in humans and monkeys, dopamine in the brain is a substrate of both MAO‐A and MAO‐B (Glover et al. 1977; O’Carroll et al. 1983). As a consequence, basal levels of brain dopamine increase with chronic administration of l‐deprenyl in these species (Riederer and Youdim 1986; Boulton et al. 1992). MAO‐B has little effect on dopamine metabolism in the rat brain, however. Thus, dopamine levels in the brain of the rat are less affected by l‐deprenyl treatment than those of humans (Kato et al. 1986; Paterson et al. 1991). The guinea pig appears to be a better model of dopamine metabolism by MAO in humans than either mice or rats (Ross 1987). Human platelet MAO is primarily or entirely of the B form, while dog platelet MAO is of both the A and B forms (Collins and Sandler 1971; Donnelly and Murphy 1977; Obata et al. 1987). As a consequence of these species variations in the metabolism, initiation of use of a MAO inhibitor in a new species should be done cautiously. MAO‐B inhibitors, specifically selegiline, have been shown to increase the life span when given to healthy mice, rats, hamsters, and dogs (Knoll 1988; Knoll et al. 1989; Milgram et al. 1990; Kitani et al. 1992; Ivy et al. 1994; Ruehl et al. 1997b). (−)Deprenyl was found to enhance anti‐oxidant enzyme activities not only in the brain’s dopaminergic regions but also in extra‐brain tissues such as the heart, the kidneys, the adrenal glands, and the spleen (Kiray et al. 2007, 2008, 2009). Several studies have also observed mobilization of many humoral factors and enhancement of natural killer (NK) cell functions with (−)deprenyl administration (Kitani et al. 2002). Selegiline also increases the survival of human patients with Parkinson’s disease (Birkmayer et al. 1985). Selegiline likewise causes a decreased accumulation of lipofuscin in various parts of the brain of aging rats (Amenta et al. 1994a, 1994b, 1994c; Zeng et al. 1994; Tseilikman et al. 2009). It also appears to facilitate activation of astrocytes that are associated with increased secretion of trophic factors, resulting in increased neuronal survival and growth (Biagini et al. 1994). It blocks the pathological changes induced by the neurotoxin 1‐methyl,4,phenyl‐1,2,3,6‐tetrahydropyridine (MPTP) (Battistin et al. 1987). Selegiline’s protective central nervous system and anti‐aging effects also seem to be due to its action potentiating the activity of the free radical scavenging enzymes, and possibly to a counteraction of free radicals and a membrane‐stabilizing action (Takahata et al. 2006; Subramanian and James 2010a, 2010b). Medications that significantly inhibit MAO‐A exist but are not used in the treatment of behavioral and mental problems in animals. Only the MAO‐B inhibitor selegiline will be discussed in detail. Therefore, all discussion of contraindications, side effects, adverse drug interactions, and treatment of overdose is presented in coverage of that drug. Selegiline is an irreversible inhibitor of MAO. It has a substantially greater affinity for MAO‐B than for MAO‐A and therefore functions as a selective MAO‐B inhibitor when given at clinically appropriate doses (Knoll and Magyar 1972; Yang and Neff 1974; Glover et al. 1977; Pfizer Animal Health and Product Information 2000). It was the first drug to be developed that was specific in its inhibition of MAO‐B (Knoll 1983). Inhibition of MAO happens in two stages: an initial reversible reaction followed by a second irreversible reaction (Heinonen et al. 1994). However, selectivity is not absolute, and rare patients may exhibit signs of MAO‐A inhibition. In average adult humans, the selectivity of selegiline’s MAO inhibition seems to disappear at a dose of about 30–40 mg total dose per day (Somerset Pharmaceuticals Inc. 2003). Following administration of 1 mg kg−1 of selegiline PO, absorption in dogs is rapid, with peak plasma concentration occurring after 20–30 minutes. Measurable concentrations are detectable in the plasma up to three hours later. The absorption half‐life is about 41 minutes whereas the elimination half‐life is about 78 minutes (Mahmood et al. 1994). Selegiline also inhibits the reuptake of dopamine, norepinephrine, and serotonin into presynaptic nerves, inhibits dopamine autoreceptors, increases the turnover of dopamine, reduces oxidative stress caused by the degradation of dopamine, increases free radical elimination by enhancing superoxide dismutase and catalase activity, potentiates neural responses to dopamine by the indirect mechanism of elevating phenylethylamine, a neuromodulator of dopaminergic responses, and enhances scavenger function in the CNS. At clinically appropriate doses, it does not have the “cheese effect,” that is, it does not potentiate the hypertensive effects of tyramine, which is characteristic of MAO‐A and mixed MAO inhibitors (Lai et al. 1980; Knoll 1983; Fagervall and Ross 1986; Knoll 1987; Heinonen and Lammintausta 1991; Berry et al. 1994; Fang and Yu 1994; Hsu et al. 1996; Pfizer Animal Health and Product Information 2000). Nevertheless, it is probably best to avoid regular use of cheese as a treat for dogs on selegiline, since a rare dog may, as happens in humans, exhibit a cheese response despite selegiline generally being very MAO‐B specific. Selegiline has three principal metabolites: l‐(−)amphetamine, l‐(−)methamphetamine, and N‐desmethylselegiline (Reynolds et al. 1978a, 1978b; Philips 1981; Yoshida et al. 1986; Dirikolu et al. 2003), and some of its pharmacological actions appear to be the result of the sympathomimetic properties caused by its metabolites (Fozard et al. 1985). See Chapter 15, CNS Stimulants, for further discussion of amphetamine. Phenylethylamine, a modulator of catecholamine neurotransmission in the CNS, is a substrate of MAO‐B, and levels of this molecule also increase following treatment with selegiline (Philips and Boulton 1979; Philips 1981; Paterson et al. 1990; Durden and Davis 1993). A study by Schrickz and Fink‐Gremmels (2014) found that selegiline did not inhibit the function of the drug transporter P‐gp in the dog. Selegiline is used to treat Parkinson’s disease, in which it potentiates the effects of L‐dopa, and Alzheimer’s disease (see, e.g. Tariot et al. 1987; Parkinson Study Group 1989, 1993; Tariot et al. 1993; Olanow et al. 1995; Sano et al. 1997; Heikkila et al. 1981). Selegiline is also used to treat major depressive disorder and anxiety disorders such as social phobia and panic disorder (Stahl 2011, 2013). Selegiline is contraindicated in patients with a known history of sensitivity to this drug. Severe CNS toxicity, potentially resulting in death, can ensue from combining selegiline with various other drugs, particularly tricyclic antidepressants (TCAs), for example, amitriptyline, clomipramine, and selective serotonin reuptake inhibitors (SSRIs), for example, fluoxetine and paroxetine. The phenomenon is called serotonin syndrome (see Chapter 19 for further discussion). The detailed mechanism of this serious drug interaction is poorly understood. Therefore, these drugs should never be combined. Because of medication half‐life, no TCA or SSRI should be given for at least two weeks following discontinuation of selegiline. Due to fluoxetine’s long half‐life, selegiline should not be given for at least five weeks following discontinuation of that drug. Even after a five‐week washout period, MAO inhibitors should be initiated with caution and the patient closely monitored, because metabolites of fluoxetine may remain in the system for longer periods of time and still induce serotonin syndrome (Coplan and Gorman 1993; Pfizer Animal Health and Product Information 2000; Somerset Pharmaceuticals 2003). Selegiline should not be given with potential MAO inhibitors including amitraz, a topical ectoparasiticide (Pfizer Animal Health and Product Information 2000). Possible drug interactions have been observed in dogs concurrently on metronidazole, prednisone, and trimethoprim sulfa. Combining selegiline with meperidine, a synthetic narcotic analgesic, is also contraindicated in humans due to the occurrence of stupor, muscular rigidity, severe agitation, and elevated temperature in some patients receiving this combination. While it is not known if this will occur in veterinary patients, it is recommended that this combination also be avoided in nonhuman animals as well (Somerset Pharmaceuticals 2003). Also, in humans, concurrent use of MAO inhibitors in conjunction with α‐2 agonists sometimes results in extreme fluctuations of blood pressure (Somerset Pharmaceuticals 2003). Blood pressure monitoring is therefore recommended in veterinary patients concurrently given selegiline and any α‐2 agonist. A study that investigated the influence of selegiline (10 mg daily−1, orally for one week) on vascular alpha‐1‐ and alpha‐2 adrenoceptor responsiveness in conscious unrestrained dogs showed that treatment induced vascular alpha‐1 and alpha‐2 adrenoceptor‐hyposensitivity (probably associated with the increase in sympathetic tone) (Pelat et al. 2001). In one clinical trial of dogs treated with selegiline, 4% of the study population experienced events sufficiently adverse to result in a reduction of dose or withdrawal from medication. Side effects experienced by these dogs included restlessness, agitation, vomiting, disorientation, diarrhea, and diminished hearing. Also, during clinical trials conducted on dogs as a part of safety and efficacy testing, three dogs showed an increase in aggression (Pfizer Animal Health and Product Information 2000). Studies to date have not identified any mutagenic or chromosomal damage potential. No evidence of teratogenic effects was identified in rats given 4, 12, or 36 mg kg−1 selegiline daily during pregnancy or in rabbits given 5, 25, or 50 mg kg−1 during pregnancy. However, in the two higher doses given to rats, fetuses exhibited a decreased body weight. At the highest dose given to rabbits, there was an increase in resorption and percentage of postimplantation losses, with a concurrent decrease in the number of live fetuses. In another study in which pregnant rats were given 4, 16, or 64 mg kg−1 daily, there was an increase in the number of stillbirths and a concurrent decrease in pup body weight, the number of pups per dam, and pup survival. At the highest dose (64 mg kg−1) no pups survived to postpartum day 4 (Somerset Pharmaceuticals Inc. 2003). In early overdose, induction of emesis or gastric lavage may be helpful, otherwise provide supportive treatment. Convulsions and other signs of CNS overstimulation should be treated with diazepam. Avoid use of phenothiazine derivatives and all CNS stimulants. Treat hypotension and vascular collapse with IV fluids. Because selegiline is used to treat an irreversible degeneration of the CNS, it should not be discontinued in patients that respond to it. It is worth noting that in veterinary medicine, it is recommended that treatment is initiated as early as possible for better results in controlling clinical signs of cognitive decline (Overall 2013) even though long‐term studies are lacking (Studzinski et al. 2005). The term cognitive dysfunction syndrome (CDS), as used in veterinary clinical behavioral medicine, refers to geriatric onset changes in behavior that cannot be attributed to medical conditions such as neoplasia or organ failure in dogs and cats. In dogs, a number of categories of behavior may be altered. First, dogs with CDS often exhibit various behaviors that suggest disorientation, for example, they may wander around the house in an aimless fashion, appear not to be able to find something, such as their bed, or get stuck behind open doors. Second, there is often altered social interaction. Usually, this is noted as a decrease in social interaction with human family members, other pets in the household, or both. Third, there is a loss of prior learned behaviors, including house‐training and basic obedience cues, such as “sit.” Fourth, sleep habits change. Total sleep increases, but nighttime wakefulness may develop. Finally, overall activity, particularly purposeful activity, decreases. Clinical signs are progressive (Bain et al. 2001; Neilson et al. 2001) and early treatment is warranted. A detailed discussion on CDS is beyond the scope of this book but age‐related behavioral changes have a high prevalence among geriatric dogs and cats and should not be overlooked (Azkona et al. 2009). Consult Araujo et al. (2005), Landsberg (2005), Head et al. (2008), Landsberg et al. (2012), and Cory (2013) for comprehensive reviews. Histologic lesions identified postmortem in the brains of dogs with CDS closely resemble lesions in the brains of humans with Alzheimer’s disease (Cummings et al. 1996b). Specifically, dogs that exhibited geriatric behavior problems before death have been identified as having meningeal fibrosis, lipofuscinosis, generalized gliosis, and ubiquitin‐containing granules in the white matter upon postmortem histological examination (Ferrer et al. 1993). There are also age‐related cerebral vascular changes and gliosis, dilation of the ventricles, and thickening of the meninges (Uchida et al. 1992; Shimada et al. 1992). β‐Amyloid plaques develop in the brains of old dogs that are similar to those found in the brains of humans with Alzheimer’s disease (Cummings et al. 1993). Deficits in discrimination learning, reversal learning, and spatial learning are strongly associated with degree of deposition of β‐amyloid in the dog brain (Cummings et al. 1996a). Dogs with CDS have hypothalamic–pituitary–adrenal axis dysregulation that occurs without typical signs of Cushing’s syndrome or other medical conditions expected to activate the hypothalamic–pituitary–adrenal axis (Ruehl et al. 1997a). Geriatric cognitive decline also occurs in the cat, although it is not as well studied as in the dog. A study by Zhang et al. (2006) found that the thickness of the molecular layer and total cerebellar cortex of older cats was significantly decreased when compared to young adults. The granular layer was increased and the density of neurons in each layer was significantly lower as well. Astrocytes were significantly denser with hypertrophy of cell bodies, and Purkinje cells showed fewer neurofilament immunoreactive dendrites. The authors concluded that these findings might underlie the functional decline of afferent efficacy and information integration in the aging cerebellum. Other reported pathological changes in the aging cat brain are neuronal loss with cerebral atrophy, widening of sulci, and increases in ventricular size. Similar to dogs, perivascular changes such as microhemorrhage or infarcts in periventricular vessels, increase in oxidative damage, and diminished cholinergic function have also been reported. Dogs and cats show Aβ brain deposition and pre‐tangle pathology with increasing age, but cats demonstrate more diffuse Aβ plaques than humans with Alzheimer’s disease and dogs with CDS (Head et al. 2005; Gunn‐Moore et al. 2006; reviewed by Landsberg et al. 2012). Among the most frequent behavioral changes in feline CDS are spatial or temporal disorientation, altered social interactions, changes in sleep–wake cycles, house‐soiling with inappropriate urination or defecation, changes in activity, and increased vocalizations (Gunn‐Moore et al. 2007). The mechanisms by which selegiline reverses CDS are not fully understood. It increases dopamine activity by several mechanisms, inhibition of MAO‐B (a dopamine metabolizer), increasing the impulse‐mediated release of catecholamines, decreasing presynaptic dopamine reuptake, increasing concentrations of phenylethylamine, which potentiates dopamine action, and increasing synthesis of aromatic L‐amino acid decarboxylase, which results in increasing dopamine synthesis (e.g. Heinonen and Lammintausta 1991; Knoll et al. 1996; Jurio et al. 1994). Selegiline also decreases free radical production and increases the activity of superoxide dismutase, which scavenges free radicals (Carillo et al. 1994). These actions are beneficial to the aging brain since free radicals contribute to the pathogenesis of neurodegenerative disorders (Gerlach et al. 1993). Better results with selegiline treatment are generally reported when the medication is started early in the progression of the disease and clinical signs are still mild (Overall 2013). Nonetheless, only a subset of dogs seems to benefit from selegiline use, with minimal clinical improvement and no research has established long‐term benefits in dogs or cats (Studzinski et al. 2005). Doses for treating cats and dogs with selegiline are given in Table 13.1. Although selegiline is not approved for use in cats, signs of Alzheimer’s disease‐like pathology have been reported in them (Cummings et al. 1996b) and signs of CDS are common in aging cats (Gunn‐Moore et al. 2007; Landsberg et al. 2012). Cats have been treated with up to 10 times the therapeutic dose with no toxicity (Ruehl et al. 1996), and geriatric cats treated with selegiline for signs of cognitive decline have shown improvement (Landsberg 1999). In dogs, selegiline hydrochloride is used to treat CDS at a dose of 0.5–1.0 mg kg−1 given once daily in the morning, and pituitary‐dependent hyperadrenocorticism is treated at a dose of 1.0–2.0 mg kg−1 daily. It has also been shown to have anticataleptic activity in research dogs, though this effect is due to activity of the stimulant metabolites rather than by MAO‐B inhibition (Milgram et al. 1993; Nishino et al. 1996; Nishino and Mignot 1997
Monoamine Oxidase Inhibitors
Action
Overview of Indications
Specific Medications
I. Selegiline Hydrochloride
Clinical Pharmacology
Uses in Humans
Contraindications
Side Effects
Overdose
Discontinuation
Other Information
Effects Documented in Nonhuman Animals
Cats
Dogs
Stay updated, free articles. Join our Telegram channel

Full access? Get Clinical Tree
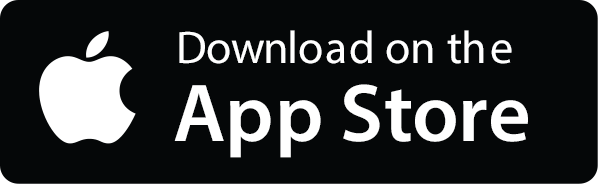
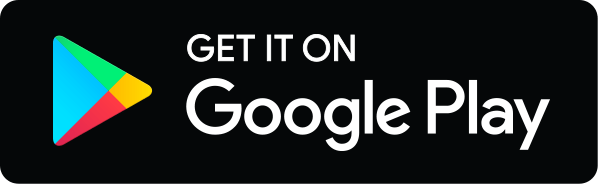