Michelle M. Dennis1,2, Kinga Molnár3, György Kriska4,5, and Péter Lőw3 1 Center for Conservation Medicine and Ecosystem Health, Department of Biomedical Sciences, Ross University School of Veterinary Medicine, Basseterre, St Kitts and Nevis 2 Department of Biomedical and Diagnostic Sciences, University of Tennessee College of Veterinary Medicine, Knoxville, TN, USA 3 Department of Anatomy, Cell and Developmental Biology, Eötvös Loránd University, Budapest, Hungary 4 Institute of Biology, Eötvös Loránd University, Budapest, Hungary 5 MTA Centre for Ecological Research, Danube Research Institute, Budapest, Hungary Gastropoda is the largest group in the phylum Mollusca, comprising over 25 000 genus‐group names that encompass snails, conch, whelks, cowries, abalone, limpets, slugs, sea hares, and nudibranchs among others (Bouchet et al. 2017; Bouchet and Rocroi 2005). Of the oldest and most evolutionarily successful animals, gastropods are globally distributed and well represented in marine, freshwater, and terrestrial habitats, and their taxonomic diversity is exceeded only by insects (McArthur and Harasewych 2003). The taxonomy, complex and highly debated, is presently under revision, allowing new insights from genetic studies to integrate with the classic phenetics approach. The revised classification approximates evolutionary relation more accurately and is arranged into clades according to common ancestors (Bouchet et al. 2017). Despite these changes, terms based on abandoned subclasses remain in use where they are morphologically informative. For example, opisthobranchs are gastropods with gills to the right and behind the heart, prosobranchs are those with gills in front of the heart, pulmonates are those with lungs instead of gills, and gymnomorphs are those without shells. The life span differs greatly among gastropods. Most of clade Heterobranchia, which includes the majority of terrestrial gastropods, live around one year. In contrast, clade Caenogastropoda often live much longer, even decades. For example, the queen conch (Lobatus gigas) is thought to become sexually mature after 3–4 years and have longevity of up to 30–40 years. Similar to other molluscs, the first gastropod life stage consists of free‐swimming ciliated trochophore larvae. The next development stage is the veliger, characterized by the growth of lobes, and a torsion process that is unique to gastropods. The body twists 180°, such that the anus and visceral mass become located just behind the head. Over time, currents may disperse these larval phases across long distances. After a period ranging from weeks to months, the veliger metamorphoses into a settled, crawling juvenile. Growth is typically rapid until reaching sexual maturity. Gastropods reproduce sexually although some parthenogenetic species exist. They are typically dioecious but some are simultaneous hermaphrodites, such as most pulmonates, or sequential hermaphrodites, such as members of superfamily Calyptraeoidea. Some simultaneous hermaphrodites may be capable of uniparental reproduction (Leonard et al. 2007). Sequential hermaphrodites are usually protandric (male‐to‐female change), but protogyny (female‐to‐male change) also occurs. Most species have anatomic structures that permit internal fertilization but external fertilization also occurs, particularly in primitive marine species. Gastropods typically copulate during a spawning season, some species showing seasonal movement patterns to meet their mates, and some displaying curious courtship or mating behaviors. Pulmonate snails and slugs may shoot calcareous “love darts” into their mates thought to promote sperm survival (Davison et al. 2005). Apophallation, where the penis is occasionally severed during copulation and then eaten by the partner, is poorly understood and best documented in terrestrial slugs (Leonard et al. 2007). In many gastropods, a storage organ of the female reproductive tract holds sperm prior to fertilization. A mucus‐ and nutrient‐rich secretion (“egg capsule”) typically encases fertilized eggs, which may be stored in the uterus until oviposition. Usually, an egg mass is produced at oviposition which may have been fertilized by more than one individual. Females of some species brood developing larvae within anatomic pouches, the mantle cavity, or just inside the shell. Most gastropods are iteroparous, having multiple reproductive cycles over a lifetime. Semelparity occurs in association with annual life span, particularly observed in the clade Heterobranchia (Zając and Kramarz 2017). The adult gastropod diet varies nearly as wide as their taxonomy. The radula, a tongue‐like chitinous structure used for prehension of food, is configured to suit the diet, so much so that its morphology can be used for species identification. Herbivorous and detritivorous species use the radula to scrape food from surfaces. Predatory carnivorous cone snails use a specialized harpoon‐like radula supplied by a venom gland to paralyze prey. Other predatory marine snails use the radula to drill through the shells of echinoderms or other molluscs. Gastropods have been an important food source since early human history. Today gastropod agriculture and fisheries are highly valued in international and artisanal markets, including queen conch (L. gigas) in the Caribbean, abalone (Haliotis spp.) in the Pacific, and periwinkles (Littorina spp.) and garden or Roman snails (Helix spp.) in Europe. Additionally, many cultures value jewelry or decorations made from the shells of gastropods. Neurobiologists use the nudibranch Hermissenda crassicornis and the Californian sea hare (Aplysia calfornica) as laboratory animals to study learning and memory. On the other hand, there are numerous problematic invasive gastropods, some representing serious agricultural or ecosystem pests while others are devastating vectors of parasitic diseases. Examples include the Spanish slug (Arion vulgaris), the giant African snail (Lissachatina fulica), apple snails (family Ampullariidae), the Budapest slug (Tandonia budapestensis), the Chinese mystery snail (Bellamya chinensis), the white garden snail (Theba pisana), and the veined rapa whelk (Rapana venosa), among many others. Gastropods are medically significant due to their role as intermediate hosts for flatworm parasites including several species of freshwater snails for schistosomes of birds, humans, and other mammals; pond snails (family Lymnaeidae) for the sheep liver fluke (Fasciola hepatica); and several species of terrestrial snails and slugs for the serious zoonoses caused by the rat lungworm (Angiostrongylus cantonensis). The presence of a well‐defined head and foot, and asymmetric organs, a feature resulting from the unique torsion process that occurs during embryonic development, characterize the gastropods. The anus and visceral mass (containing much of the digestive and reproductive tracts, kidney, and heart) lie dorsal to the head and to one side of the median plane of the body. Many organs that are paired in other molluscs, such as gill (or ctenidium), gonad, and kidney (or nephridium), are undeveloped or lacking on one side (usually the left) of the body. Some gastropods, particularly opisthobranchs and pulmonates, detort in later developmental stages such that these features may be less obvious (Gosliner 1994). Many gastropods have a shell, in which case it is usually spiraled with a right‐handed aperture (Figure 4.1) (Hyman 1967), but may be absent or reduced, especially in opisthobranchs. It is typically composed of calcium carbonate as aragonite, sometimes containing calcite, in an organic protein matrix termed conchin. An operculum, a horn‐like or calcareous process attached to the posterior foot, serves to close the shell’s aperture in prosobranchs (Voltzow 1994). Figure 4.1 Shell of Lobatus gigas. The apex (arrow) of the shell was formed first and is at the caudal aspect of the shell, at the tip of the spire. The final whorl (W) of the shell opens to form the aperture (asterisk) through which the head and foot protrude and may be closed by an operculum. The aperture usually opens on the right side, as pictured here, and is bordered by inner and outer (arrowhead) lips. Figure 4.2 (a) Helix pomatia in anesthetized state. The head (arrowhead) and foot (arrow) of the gastropod merge to form the body, which extends beneath the mantel (M). (b–d) The necessary cuts to dissect the body. (b) The first cut of the dissection under the mantle edge. (c) The second cut follows the rectum (R) to the highest level of the pulmonary chamber. (d) The third cut goes from the visceral mass forwards through the body wall along the dorsal midline to the dorsal lip of the mouth. Typically, the gastropod head bears a pair of eyes, tentacles (or rhinophores), and buccal mass (or proboscis, i.e., mouth and pharynx) (Figure 4.2). Within the final whorl of the shell, the mantle lines the inner shell, forming a space which encloses the head, gill (or lung), and openings of the digestive, excretory, and reproductive tracts; this space is termed the mantle (or pallial) cavity (Figure 4.3). The mantle that forms the roof of the mantle cavity contains the pallial complex (collectively, the gill[s], osphradium, and hypobranchial glands). The nervous system consists of paired ganglia connected by a nerve loop throughout the head, foot, and visceral mass (Hyman 1967; Little 1965). The gastrointestinal tract courses in a U‐shape from the buccal mass, through the body, then the visceral mass, finally terminating in the mantle, near the head. Gastropods have an open circulatory system where hemolymph flows through sinuses rather than endothelium‐lined capillaries (Jones 1983). The heart, made of a single ventricle and auricle and lying within a pericardial sac, works to direct hemolymph flow from the organs to the kidney and gill, and then back to the heart. Hemocyanin is the copper‐based oxygen‐carrying pigment that gives hemolymph its blue tinge. Marine gastropods have a gill for respiration, whereas terrestrial and freshwater gastropods have a pallial lung (Figure 4.4) (Luchtel et al. 1997). The lumen of the kidney is continuous with the pericardial space through the renopericardial pore, and empties into the mantle cavity through the nephridiopore, adjacent to the anus (Voltzow 1994). There is profound variation in the reproductive anatomy of gastropods such that only generalizations can be provided here (Hyman 1967). The gonad (or ovotestis in hermaphrodites) is always solitary and confluent with the digestive gland. In lower gastropods that fertilize externally, the reproductive system consists of the gonad and a gonoduct that opens into the kidney. In male gastropods that fertilize internally, sperm pass through a sperm duct (or vas deferens) to the prostate, where mucous secretion suspends sperm (Reed 1995a). Sperm exit through the gonopore, and in some species, through a penis (or verge) on the right side of the head. The penis attaches to the female bursa copulatrix. In the female, ova pass through a duct (oviduct) from the gonad to a uterus (or ampulla, pallial oviduct) (Hyman 1967; Reed 1995b). There may be an adjacent seminal receptacle (or spermothecae) where sperm can be stored. Secretion from nidamental glands (variously termed mucus, jelly, albumen, or capsule glands, depending on the nature of the secretion) encapsulates eggs and forms the egg mass. The uterus may store fertilized eggs until they pass down the ovipositor groove at oviposition. In the hermaphrodite, the ovotestis produces eggs and sperm simultaneously (Duncan 1958). A hermaphrodite duct leaves the ovotestis and branches into a sperm duct and oviduct. The sperm duct will proceed to a prostate and penis, whereas nidamental glands, a bursa copulatrix, and potentially a seminal receptacle, supply the oviduct. Figure 4.3 Two female Lobatus gigas, removed from shell; (a) mantle cavity unopened, and (b) mantle cavity opened. The operculum (O) is attached to the foot (F) which is continuous with the head, bearing two eyestalks (E) and a buccal mass (B). A verge is present on the body of males, and an egg groove (Eg) on the body of females. The visceral mass is viewed once the animal is removed from the shell and from its exterior, the digestive gland (D), stomach (S), and gonad (Go) are evident. The mantle (M) forms a cavity that contains the gill (G), hypobranchial glands (Hg), rectum (R), and distal reproductive tract (U). Further dissection is required to reveal the heart (H), kidney (K), nephridial gland (N), and intestine (I). Figure 4.4 Dissected Helix in water cover displaying the internal organs. AG, albumen gland; BC, bursa copulatrix; BS, bursa stalk; BSD, bursa stalk, diverticulum; CeNR, circumesophageal nerve ring on pharynx; DG, digestive gland; DS, dart sac; E, esophagus; EP, epiphallus; Fl, flagellum; GA, genital atrium; H, heart in pericardium; HD, hermaphrodite duct; K, kidney; L, lung; ME, mantle edge; MuG, mucous gland; Om, ommatophore; Ovo, ovotestis; P, penis; Ps, pneumostome; PV, pulmonary vein; R, rectum; SG, salivary gland; SI, small intestine; St, stomach; Sod, spermoviduct; Up, ureter, primary; Us, ureter, secondary; V, vagina; VD, vas deferens. This section provides an overview of the microscopic anatomy of each major tissue with the ambitious aim of summarizing the basic features across a broad and diverse taxonomic group. There is a need for research that clarifies fundamental differences among clades and readers should be mindful that some species may not strictly confine to the generalizations made here. The well‐studied pulmonate Roman snail (Helix pomatia), a subject of interest for the chapter’s authors and arguably one of the most economically important gastropods, is used as a prototypical example. Integument covers the entire surface of the gastropod’s soft tissues, including head, foot, body, mantle and, although not apparent grossly, even the outer visceral surfaces. The role of the integument is to provide a protective barrier. It may also facilitate respiration sensation, locomotion, absorption of nutrients, osmoregulation, secretion of substances, and reproduction. Secretions include mucus, defensive substances, and shell components. The integument consists of an epithelial surface, commonly referred to as epidermis. Regardless of location, histologically the epidermis comprises a single layer of tall cuboidal to columnar epithelial cells supported by a basement membrane (Figure 4.5). The epithelium consists of epidermal cells (the generic columnar cell of the epidermis), ciliated cells, and mucocytes, all of which bear a microvillus border that may be difficult to discern histologically. There may also be other types of secretory cells, pigmented cells, and sensory cells. Similar to other molluscs, pigments present in gastropod tissues including the epidermis are diverse and can include carotenoids, indigoids, melanin, porphyrins, and bilichromes (Fox 1983). Secretory cells may form intraepidermal glands (for example, hypobranchial gland, Figure 4.6), or may invaginate to form tubules or subepidermal glands. In opisthobranchs, a wide variety of secretory cells are observed. Some may project well into the subepidermal connective tissue, and be relatively large and distended with discrete vesicles such that they might not be recognizable as cells unless the nucleus is in section. In some species, epidermal cells or associated glands may show bioluminescence (Tsuji 1983) and in others, harbor symbiotic dinoflagellates (zooxanthellae) (Wa¨gele and Johnsen 2001). A dermis is not distinct in gastropods and thus, the epidermis is supported by connective tissue blending with and similar to that found throughout the body. Although not readily apparent with HE stains, sensory neurons and their processes lie just beneath the epidermis. The body surface of Helix is covered with a single‐layered columnar epithelium equipped with both cilia and microvilli. Large mucus‐secreting and mucus‐storing cells extend deep into the subepithelial matrix, surrounded by individual smooth muscle cells (SMC) and connective tissue. The body, visceral mass, foot, mantle, and shell are bound together by connective tissue. In prosobranchs connective tissue is typically fibrous and frequently contains abundant mucus‐producing and glycogen‐storing cells called “Leydig” (or vesicular or chondroid) cells (Figure 4.7) (Luchtel and Deyrup‐Olsen 2001; Voltzow 1994). Cells containing deposits of calcium and other minerals are also not uncommon. In opisthobranchs, connective tissue is similar. Stellate cells predominate, intermingled with glycogen‐storing Leydig cells and a few muscle fibers, and may also contain calciferous cells, plasmatic cells, rhogocytes (Wa¨gele 1998), and pigmented cells (Figure 4.7b) (Gosliner 1994). Nerves and hemolymphatic vessels are present throughout connective tissue. Comparable special cell types have been identified in the connective tissue of terrestrial gastropods including rhogocytes, granular cells, and hemocytes. Rhogocytes are large, usually spherical to elongate in shape, but occasionally branched and somewhat irregular in form. Their most characteristic feature is the cell surface forming a system of surface cisternae that is partly enclosed yet communicates with the extracellular space. The name rhogocyte – from Greek rhogos which means “slit” – refers to the slits on the cell surface leading to these cisternae. The slits are bridged with fine, fibrillar diaphragms. Rhogocytes possess numerous lysosomes, lipid droplets, granules, and abundant rough endoplasmic reticulum. They may have extensive glycogen stores that can displace the spherical nucleus to a peripheral position. These cells play an important role in metal ion metabolism, possibly involved in the recycling of respiratory pigments and in metal detoxification. The large amount of glycogen found in rhogocytes suggests that these cells may also be involved in transport or storage of nutrients (Skelding and Newell 1975). Granular cells are characterized by cytoplasm that contains glycoprotein granules that sometimes pack the cells enough to deform the nuclei. The granules originate from the Golgi apparatus and are secreted by exocytosis (Steinbach 1977). Hemocytes are described in section 4.3.8.2. Figure 4.5 Comparison of integument in (a) mantel of Littorina ziczac, 400×, HE; (b) body of Coralliophilia sp., 400×, HE (inset: 1000×, HE); (c) body of Aplysia californica, 200×, HE. The epidermis consists of simple columnar ciliated epithelial cells, including many secretory cells and pigmented cells. Inset of (b) shows higher magnification of epidermal cells and more than one type of secretory cell. The epidermis may be sinuated forming gland‐like invaginations (arrows). Opisthobranchs often have subepidermal gland cells (white arrowheads). The subepidermal connective tissue is similar to that found throughout the body, fibrous and often interspersed with pigment cells (black arrowheads). Source for (c): Elise LaDouceur. Figure 4.6 Hypobranchial gland of Cittarium pica. The gland is composed of columnar epithelial cells and mucocytes that form fronds supported by connective tissue septae. 400×. HE. Figure 4.7 (a) Connective tissue of the body of Lobatus gigas. Fibrous connective tissue contains many vacuolated Leydig cells (asterisks) and myocytes (arrows). 400×. Masson’s trichrome stain. Inset left: Myocytes are difficult to differentiate from collagen with HE stain. 400×. HE. Inset right: Leydig cells contain glycogen. 400×. PAS. (b) Connective tissue of the body of Aplysiacalifornica. Fibrous connective tissue containing Leydig cells (asterisks) and plasmacytic cells (arrows). 400×. HE. Source for (b): Elise LaDouceur. The mantle is a fold of body wall that covers the visceral mass. It consists of muscle and connective tissue with an integument surface. In shelled gastropods, the mantle lines the inner shell surface of the last whorl and, at its anterior aspect, forms a skirt around the mantle cavity. It may also form a siphon to draw water into the mantle cavity, bear tentacles or papillae, or have lobes that can extend to cover the shell. In opisthobranchs, the mantle can similarly extend to form flaps. In addition to being a protective barrier from the environment, it is responsible for shell formation, mucus production, sensation, and may have roles in respiration and assimilation of nutrients (Voltzow 1994). Histologically, the mantle epidermis composition varies by location (Figures 4.8 and 4.9). On the inner or ventral surface of the mantle, cells are typically heavily ciliated and more generously interspersed with other specialized cells types, including pigmented and secretory cells (Hyman 1967). The dorsal mantle epidermis comprises mainly epidermal cells with fewer specialized cell types and may be thrown into folds resembling glands. The connective tissue supporting the mantle is similar to connective tissue throughout the body. Mantle‐edge glands are responsible for production of the shell (Bevelander and Nakahara 1970; Kapur and Gibson 1967). These typically consist of acini of tall cuboidal epithelial cells and secrete to the surface without a clearly defined duct (Figure 4.10). Potentially to provide added protection where the shell has been lost, opisthobranchs may have defensive glands within the mantle tissue, with a variety of names, locations, compositions, and secretions (Àvila and Durfort 1996; Bezerra et al. 2006; Klussmann‐Kolb 2004; Wa¨gele 1998). In Aplysia, an ink gland may be present, composed of large flask‐shaped secretory cells that contain granular to clear vesicles of secretory material, released through a short duct lined with cuboidal epithelial cells at the secretory cell’s apex (Figure 4.11) (Bezerra et al. 2006; Prince et al. 1998). These are surrounded by muscle and collagen fibers, and other “dispersed” epithelioid cells (Bezerra et al. 2006). Figure 4.8 Ventral (interior) mantel of Lobatus gigas. Epidermal cells are ciliated and interspersed with many mucocytes (arrows) and other secretory cells (arrowhead). Beneath the epidermis is fibrous connective tissue containing many myocytes. 400×. HE. Figure 4.9 Dorsal (exterior) mantel of Lobatus gigas. Epidermal cells are less specialized but more heavily sinuated. 400×. HE. Figure 4.10 Mantle edge of Lobatus gigas. Arrows designate the mantle edge gland. 100×. HE. Inset: Higher magnification revealing detail of the gland, acini of tall cuboidal epithelial cells that empty to the surface without a clearly defined duct. 200×. HE. Although the mantle in Helix does not have folds, the mantle edge can be subdivided into three zones (Figure 4.12). Figure 4.11 Ink gland of mantle edge of Aplysia dactylomela. A large flask‐shaped secretory cell (arrow) contains a vesicle filled with ink secretion. Arrowhead designates the cell nucleus. 100×. HE. Inset shows detail of the duct, lined by cuboidal epithelial cells, and marginated by dispersed epithelioid cells (asterisk). 400×. HE. Source: Luis E. A. Bezerra and Vânia M. M. Melo. Figure 4.12 Overview of the mantle collar with zones of Helix. E, epithelium; GC, gland cells; GOE, general outer epithelium; IGZ, inner glandular zone; MB, muscle bundles; ML, muscle layer; PG, periostracal groove; SG, subepithelial glands. 40×. HE. Figure 4.13 Histologic structure of the mantle collar of Helix. (a) Mantle collar (at the lung) with unicellular gland types. 300×. HE. (b) Ciliated epithelium near the pneumostome. 500×. HE. (c) Mantle collar gland cell types. 900×. HE. (d) General outer epithelium at mantle collar and beneath the shell (inset). 60×. HE, inset 600×. BL, basal lamina; C, cilia; CF, collagen fibers; CG, calcium gland; Ci, cytoplasmic inclusion; E, epidermis; FB, fibrous bundles for attaching to the shell; Hs, hemolymph sinus; M, melanocytes; MB, muscle bundles; Mc, mantle cavity; MC, muscle cell; MG1, mucous gland with granular secretion; MG2, mucous gland with transparent secretion; MV, microvilli; PG, protein gland; RE, respiratory epithelium; SG, subepithelial glands; V, vacuoles; arrows, secretion. The shell of Helix consists of calcium carbonate (CaCO3) in the aragonite phase. Morphologic analysis showed the microstructure of the shell, composed of parallel lamellae and a very different appearance of the inner and outer shell surface. The inner surface is covered by longitudinal structures, whereas the outer surface is covered by a layer of small granules (Ba¨chle et al. 2006). Figure 4.14 shows undecalcified thin ground section of the snail shell. The multilayered construction of the biomaterial can be seen with the crossed lamellar structures of parallel laths. Figure 4.14 Undecalcified thin ground section of the shell of Helix. Ch, conchiolin; EO, epiostracum; O, ostracum. 200×. The ventral aspect of the roof of the mantle may bear one or two hypobranchial glands that run parallel to the gill on its right side, between the gill and rectum. Its role is to secrete mucus to facilitate clearing of debris from the mantel cavity, and in Muricidae it produces a purple pigment that may be incorporated into egg masses. Histologically, the gland consists of modified mantle epidermis where simple to pseudostratified tall columnar epithelial cells are often ciliated and heavily interspersed with mucocytes and several different types of other secretory cells (Figure 4.6) (Westley et al. 2010). Some of the secretory cells secrete acid mucin that stains metachromatically with toluidine blue (Voltzow 1994). As gastropods lack a body cavity, muscle and connective tissue form the interior of the animal and, like other molluscs, gastropods lack a skeletal system. Although muscle fibers are present throughout all parts of the body, the two main muscles of the gastropod are the columellar muscle and the tarsos muscle of the foot. The columellar muscle forms the bulk of the body of the gastropod. It originates at an attachment to the columella (the central axis) of the shell and becomes continuous with the muscle of the foot. Its main function is to draw the animal into its shell. The gastropod uses its muscular foot (tarsos muscles) for locomotion, mating, ovipositioning, defense, and prey capture. In some cases, particularly in marine prosobranchs, the foot muscle inserts on to the operculum. Histologically, muscle cells are fusiform and unicellular and may be smooth or striated (Chantler 1983). They may be organized into fibers that weave in all directions and are heavily intermingled with connective tissue. In some instances, muscle cells may be highly ordered into anatomically consistent bundles or layers. The foot is composed of highly interwoven and branching bundles of myocytes. The supporting connective tissue and epidermis are also similar to that of the mantle; only the latter can be heavily sinuated, particularly on the sole. Epithelium of the sole may be more heavily ciliated, whereas the epithelium of the sides and dorsum is not. In some species, intra‐ or subepidermal glands may supply the foot, for which the locations are highly variable. A pedal or suprapedal gland, formed by subepidermal acini of oval epithelial cells, may be present to supply the ventral foot with a secretion thought to facilitate locomotion (Shirbhate and Cook 1987). The general appearance of the foot of Helix is a reticulum of grooves subdividing the surface into grayish polygonal areas (see Figure 4.2). Microscopically, apart from the grooving, the structure is remarkably reminiscent of the mantle collar and the same kinds of glands are present (Figure 4.13). The main difference is their smaller size, particularly on the dorsal aspect. There are isolated patches of ciliated cells in the epidermis, but the majority of the surface bears no trace of them. Type 1 mucus glands occur commonly in this region. Protein and calcium glands are less abundant than in the mantle, particularly on the dorsal surface. Those cells containing either pigment or lipid occur sparsely as before. The ventral foot of Helix is divided into two layers parallel with the body surface: the superficial layer and deep subepithelial layer (Tonar and Markos 2004; Wondrak 2012). The body wall epithelia include several regions with mixtures of gland cell types, each combination being characteristic of the specific region. Their cell bodies containing the nucleus are situated underneath the epithelium at different depths within connective tissue (unicellular exoepithelial glands), an adaption to terrestrial life to avoid dehydration, and empty their secretions through their necks directly onto the surface of the body wall. Besides such polytypic glandular regions, the foot has two monotypic gland‐cell regions. Figure 4.15 Pedal glands of Helix. (a) Overview of pedal protein gland and suprapedal gland (plane of the upper section is anterior to the lower one). 30×. HE. (b) Cell types of pedal protein gland and suprapedal gland. 300×. HE. (c) Ciliated cells of the duct of suprapedal gland and secretion. 900×. HE. (d) Overview of the suprapedal gland (planes of the sections are posterior to section in panel a, most caudal is bottom one). 30×. HE. A, acidophil cytoplasm; C, cilia; CT, connective tissue; D, duct of the suprapedal gland; E, epithelium of duct; F, foot; FSC, funnel‐shaped cavity; GP, agranular cell cytoplasm; GR, granular cell; Hs, hemolymph sinus; MC, melanocyte; MFA, middle foot artery (branch); N, nerve; NC, neck of gland cell; PPG, pedal protein gland; S, secreted material; SPG, suprapedal gland; VC, visceral cavity. The columellar muscle has a composition similar to the foot, including the predominance of muscle cells, supported by connective tissue and lined by a surface epidermis. The muscle cells are typically arranged into thick bundles to form orthogonal latticework (Voltzow 1994) (Figure 4.16). Figure 4.16 Columellar muscle of Triplofusus papillosus. Myocytes are arranged into intersecting bundles. 400×. HE. The digestive system of gastropods is highly adapted to diet, and thus shows significant variation. It is typically U‐shaped, particularly in torted gastropods (i.e., those that do not detort after the torsion process during the veliger stage). A protrusible radular apparatus lines the buccal mass, consisting of radula (a set of chitinized teeth), radular membranes, and odontophore (or jaw). The esophagus is a thin‐walled organ that courses through the columellar muscle toward the visceral mass where it joins the stomach. Near the buccal mass, it is flanked by salivary glands (SG) and may give rise to a compartment (crop) near the stomach. The stomach often has a cuticularized shield and functions to grind food. It is supplied by a digestive gland (or hepatopancreas, digestive cecum, or midgut gland), and also a style sac in microherbivores. The style sac creates a current to move ingesta and may also contain a crystalline style, a cylindrical gelatinous structure that rotates and facilitates mechanical breakdown of ingesta. The digestive gland is responsible for enzymatic digestion and absorption of nutrients. A gizzard is present in some gastropods. In opisthobranchs, a gizzard is a distal compartment of the esophagus with a thick muscular wall and a heavy cuticle with teeth or plates, whereas in pulmonates, the gizzard refers to a stomach, which has a thick muscular wall. The intestine serves to package feces rather than absorb nutrients. In carnivores, it is often short and straight, whereas in herbivores it is longer and loops or coils near the kidney and heart. The intestine courses through the mantle as the rectum. The anus empties into the mantle cavity close to the gill. A cuticle covers the inner surface of the buccal mass. The radula consists of a thin chitinous band with teeth‐like ridges (Figures 4.17 and 4.18). Odontoblasts, pseudostratified tall columnar epithelial cells at the posterior end of the radula, form new teeth. Two rows of similar columnar epithelium, the supraradular and subradular epithelium, together form the radular sheath that encapsulates the radula. Anteriorly, muscle bundles are attached to the radula for its manipulation, and the radula bends ventrally where it contacts the odontophores, dense plates of connective tissue resembling cartilage, only containing myoglobin and polyhexose sulfate instead of chondroitin sulfate (Lash 1959). Figure 4.17 Radular apparatus of Cerithium sp. Within the buccal cavity (B), the radula (R) is ensheathed by supraradular epithelium (arrows, inset above) and subradular epithelium (arrowheads, inset below). Muscle (M) connects to the radula and associated odontophores (O). 200×. HE. Figure 4.18 Posterior radular apparatus of Nerita peloronta. Odontoblasts are pseudostratified tall columnar epithelial cells that form new radula. 200×. HE. Figure 4.19 Pharynx and radula of Helix. (a) Overview of the cross‐section of pharynx. 20×. HE. (b) Cross‐section of an odontoblast group. 400×. HE. (c) Cross‐section of radula with matured teeth. 350×. HE. (d) Cross‐section of the odontophore. 200×. HE. BL, basal lamina; BP, basal plate; CS, collostyle; CT, connective tissue layer; GR, granulocytes; Hs, hemolymph sinus; IE, inferior epithelium; M, muscle cells (cross‐section); Mc, circular muscle layer; MC, mineralizing cells; MCd, degenerated mineralizing cells; MF, muscle fibers; Ml, longitudinal muscle layer; MO, muscles of the odontophore; MR, muscles of the radula; O, odontophore; OBG, odontoblast cell group; R, radula; RM, radular membrane; RM1, RM2, layers of the radular membrane; SC, supporting cell; SE, superior epithelium; T, tooth; VBA, ventral buccal artery; VC, vesicular connective tissue cell. In Helix the buccal mass is arranged in three concentric and integrated envelopes (Figure 4.19). In pulmonates, the multidenticular radula is generated by numerous groups of a few voluminous cells (situated in odontoblast groups). Pulmonates produce the radula matrix by microvilli, cytoplasmic protrusions, and apocrine secretions (Mackenstedt and Märkel 1987). The posterior part of the radula is located in a blind pouch, the radular sac or radular sheath. It is a U‐shaped groove and the lateral teeth are directed inwards. The collostyle protrudes into the groove from above. The radular sheath consists of two epithelia, the superior and the inferior epithelium, which are linked with the radula. In pulmonates, the few odontoblasts are located at the lower posterior end of the sheath. Odontoblasts are concentrated into groups of a limited number of cells, each secreting the teeth of one longitudinal row. The odontoblasts form the matrix of the radula, whereas the superior and the inferior epithelium contribute to the maturation and the transport of the radula. Cells of the odontoblast group include the following (see Figure 4.19a,b). The superior and inferior epithelium are migrating epithelia. There are mineralizing and supporting cells in the superior epithelium (see Figure 4.19b,c). The mineralizing cells deposit minerals and organic compounds into the newly formed teeth to make them hard. The change in the chemical composition is illustrated by change of the staining properties. The matrix of the newly formed teeth consists of protein and mucopolysaccharides, mainly chitin, but an intussusception of minerals and organic compounds causes hardening of the teeth. After fulfilling their purpose, mineralizing cells die. Supporting cells reach to the basal plate between the teeth. They do not deposit minerals so the areas between the teeth remain flexible. Electron microprobe analysis shows that mainly calcium is incorporated into the teeth of the radula, whereas the radular membrane lacks minerals (Mackenstedt and Märkel 1987). The radular membrane covers the migrating inferior epithelium in the radular sheath. At the boundary between two basal plates, the radular membrane becomes thinner whereas the parts of the membrane just beneath the basal plates remain thick. Functional aspects can explain this – for example, the thicker part of the membrane supports the teeth during feeding and protects the inferior epithelium against tensile forces. The radular membrane reveals two distinct layers in H. pomatia. Salivary glands supplying the anterior esophagus are typically simple tubular glands composed of columnar epithelial cells that form tubules or acini. Acini may be lined by two cell types: a basilar pyramidal vacuolated cell and a columnar ciliated cell (Andrews 1991). The wall of the esophagus is typically very thin, consisting of muscle cells arranged in a circular orientation around the lumen. The mucosa is composed of simple columnar microvillated epithelial cells, some bearing cilia, and an adventitial surface comprises loose fibrous tissue containing many nerve bodies and hemolymphatic vessels. Often the mucosa is arranged into longitudinal folds, and these may contain greater numbers of mucocytes at their tips (Figure 4.20). These folds are typically reduced or absent in the posterior esophagus. There may be discretely vacuolated cells of unknown function just beneath the mucosa (Lobo‐da‐Cunha et al. 2011). Morphologically, the crop and esophagus are similar (Howells 1942). Figure 4.20 Esophagus of Cerithium sp. The esophagus has a very thin wall consisting of a circular layer of myocytes (M) and a mucosa arranged into longitudinal folds (arrows). At its anterior, it is often flanked by salivary glands (asterisks) and ganglia (G). 200×. HE. The Helix esophagus wall is lined with a simple columnar epithelium (Figure 4.21), covered with a thin cuticular layer, the microvilli. The nuclei of the epithelial cells are located at various levels, usually closer to the base. Among the epithelial cells, there are two types of glandular cells (MU1 and MU2), characterized by a bottle‐like shape with distinct “necks.” Their nuclei are located basally, in their widest part. They differ in their secretion: mucin with no staining and acidic mucopolysaccharide with blue staining. Below the epithelium, there is connective tissue and muscle fibers (Koralewska‐Batura 1999). Figure 4.21 Longitudinal section of the esophagus of Helix. BB, brush border; BL, basal lamina; CF, collagen fibers; Cut, cuticular layer; E, epithelium; Hs, hemolymph sinus; MC, muscle cells in longitudinal layer; MLC, muscular layer, circular; MU1, mucus cell type 1; MU2, mucus cell type 2; white arrows, brownish granules in apical domain of supporting cells; black arrows, apical domain of mucus cells. 250×. HE; inset 700×. In Helix, the terminal part of the esophagus widens gradually to form a crop (Figure 4.22) which is often regarded as part of the stomach. The crop wall is lined with columnar epithelium, which also contains glandular cells (see Figure 4.22). Below the layer of epithelial cells there is connective tissue interspersed with muscle fibers that run in various directions. In the crop of Helix, the food is subject to preliminary enzymatic treatment (Koralewska‐Batura 1999). In Helix, the SG consist of numerous acini and the saliva secreted is forwarded from the acini into the intralobular (SDi) and then the interlobular ducts that converge on the paired muscular primary salivary ducts (SD), which finally open into the buccal cavity (see Figure 4.22). In SD, the saliva is driven by the peristaltic contractions of the muscle fibers of the duct toward the buccal mass. The cell types of the SG are as follows. Figure 4.22 Crop and salivary gland in Helix. (a) Longitudinal section of the crop. 300×. HE. (b) Cross‐section of the salivary gland and primary salivary duct. 350×. HE. BL, basal lamina; C, cilia; CC, cystic cell; CD, cell debris; CF, collagen fibers; CT, connective tissue; Cut, cuticular layer; CW, crop wall; E, epithelium; GC, granular cell; Hs, hemolymph sinus; L, lumen of the primary salivary duct; MC, muscle cells; MU, mucocyte; SDi, salivary duct, intralobular; SDp, salivary duct, primary; SG, salivary gland, lobe; V, hemolymph vein; VC, vacuolated cell. Neutral and acid mucins lubricate the food, while the proteins constitute the enzymatic supply in the early phase of digestion (Pirger et al. 2004). The gastric mucosal surface may be covered by a cuticularized chitinous gastric shield along its dorsal and left walls (Figure 4.23). The mucosa is lined by simple columnar epithelial cells with a microvillus border and is variably populated by mucocytes. Ridges and folds in the mucosa corresponding to typhlosoles may be present near the esophagus or small intestine and in these areas the epithelium is often ciliated and may be pseudostratified or bilayered. The submucosa consists of connective tissue seen throughout the body, but perhaps containing greater amounts of hemolymphatic vessels and hemocytes. The muscularis blends with the submucosal connective tissue and is composed of muscle cells arranged in a circular orientation around the longitudinal axis of the lumen. The external surface of the stomach comprises epidermis, similar to the mantle and other body surfaces. In the stomach of Helix there are three large, very characteristic folds. Two of them, one located in the dorsal and the other in the central part of the stomach, are much larger than the third. They form a gutter – the typhlosole running toward the intestine. The third fold is located between the two outlets of the digestive gland lobes. Between them, there are much smaller folds. The stomach wall is lined with a ciliated columnar epithelium with numerous glandular cells. Below the epithelium there is connective tissue, its main component being lipid cells and relatively numerous muscle fibers. The stomach muscular layer is built of circular, longitudinal, and oblique fibers. Two ducts of the digestive gland open to the stomach. Digestion mechanisms in the stomach of pulmonate snails result in only the finest food particles, not exceeding 1 μm, getting into the digestive gland where they are absorbed. Larger particles are moved toward the intestine (Koralewska‐Batura 1999). Figure 4.23 Stomach of Cerithium sp. In areas, the gastric mucosa is covered by a cuticle (arrow). 400×. HE. The style sac mucosa consists of tall cuboidal to columnar epithelial cells with long cilia (Figure 4.24). There are often folds corresponding to a typhlosole with a central intestinal groove. This area is lined by pseudostratified tall columnar ciliated epithelial cells (Mackintosh 1925). If present, the crystalline style is poorly stained and appears as amphiphilic homogenous acellular substance. The mucosa of the small intestine may also be dominated by typhlosoles (Figure 4.25). Similar to the style sac and stomach, where present these bear pseudostratified to bilayered tall columnar ciliated epithelial cells. In the furrows of the ridges, the mucosa is lined by simple columnar epithelial cells. The rectal mucosa is composed of simple columnar epithelial cells but may also contain granular mucocytes. The anal mucosa consists of pseudostratified ciliated columnar epithelial cells, heavily interspersed with mucocytes (Figure 4.26). The submucosa, muscularis, and external surface of all segments of intestine are similar to the stomach. The external surfaces of the rectum and anus are integrated with the mantle and are often coated in mucus derived from the hypobranchial gland. Figure 4.24 Style sac of Lobatus gigas. A distinct fold in the wall is a reflection of a typhlosole. The crystalline style (asterisk) is composed of homogenous acellular substance. 40×. HE. Inset: Higher magnification shows detail of mucosa, consisting of simple cuboidal epithelial cells with long cilia. 400×. HE. Figure 4.25 Intestine of Cittarium pica. Prominences reflect typhlosoles (arrows). The mucosa (M), submucosa (S), and muscularis (Mu) form distinct layers, although in many species the muscularis is not so distinct. The exterior comprises epidermis (E), in this instance bearing many mucocytes that have coated the specimen in mucoid secretion (asterisk). 100×. HE. Inset: Higher magnification of a typhlosole shows bilayered columnar ciliated epithelial cells. 200×. HE. The intestine of Helix consists of the small intestine and rectum. Both these sections are lined with columnar epithelium with numerous glandular cells. In the folds of the glandular part of the small intestine there occur numerous goblet cells (Figure 4.27
4
Mollusca: Gastropoda
4.1 Introduction
4.1.1 Taxonomy
4.1.2 Life History
4.1.2.1 Life Expectancy
4.1.2.2 Reproduction
4.1.2.3 Diet
4.1.3 Relevance
4.2 Gross Anatomy
4.3 Histology
4.3.1 Integument
4.3.2 Connective Tissue
4.3.3 Mantle
4.3.3.1 Hypobranchial Gland
4.3.4 Musculoskeletal
4.3.4.1 Foot
4.3.4.2 Columellar Muscle
4.3.5 Digestive System
4.3.5.1 Alimentary Canal
4.3.5.1.1 Mouth and Radula
4.3.5.1.2 Esophagus
4.3.5.1.3 Stomach and Style Sac
4.3.5.1.4 Small Intestine and Rectum
Stay updated, free articles. Join our Telegram channel

Full access? Get Clinical Tree
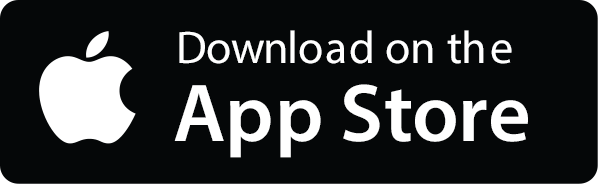
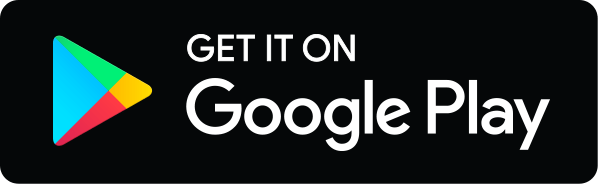