NRG1–EGF+/−
NRG1–TM+/−
NRG1–Ig+/−
NRG1–CRD+/−
NRG1type1-tg
Neonatal injection NRG1β1
Locomotor activity
Open-field test
= (100)
= (102)
= (103)
T-maze
↑ (91)
n.d.
= (99)
n.d.
n.d.
n.d.
Performance
Rotarod
↑ (91)
n.d.
n.d.
↓ (102)
n.d.
Executive function
LI
n.d.
n.d.
n.d.
n.d.
↓ (103)
PPI
↓ (100)
↓ (102)
↓ (103)
Fear conditioning
↓ (93)
n.d.
n.d.
n.d.
n.d.
n.d.
Anxiety
Light–dark test
= (109)
n.d.
n.d.
= (102)
n.d.
Elevated plus maze
= (109)
n.d.
n.d.
= (102)
n.d.
Working memory
T-maze
n.d.
n.d.
n.d.
↓ (100)
n.d.
n.d.
Social interaction
↓ (93)
n.d.
n.d.
n.d.
↓ (103)
Clozapine
n.d.
n.d.
Decreased
locomotor activity, rescued LI (99)
n.d.
n.d.
n.d.
5.1 NRG1–EGF+/− Hypomorphs
NRG1–EGF+/− mice are hyperactive in the open field test (91). The mutant mice would complete the T-maze test faster. They also performed better in rotarod test, a test of forced motor activity that is associated with cerebellum and motor cortex. Ehrlichman (93) studied another line of NRG1–EGF+/− mice (94) where EGF domain was partially deleted. The mice display reduced mismatch negativity, contextual fear conditioning, and social interactions, although their prepulse inhibition and locomotion appear normal.
5.2 NRG1–TM+/− Hypomorphs
NRG1–TM+/− mice are hyperactive in the open-field test (69, 95–97). The aggressive behavior in NRG1–TM+/− mice is increased while the response to social novelty is reduced. The NRG1–TM+/− mice appear normal in spatial learning and working memory (98). Intriguingly, clozapine was able to reverse the hyperactive phenotype (69). Whether NRG1–TM+/− mice are impaired in prepulse inhibition is controversial (96–97). NRG1–TM+/− mice showed anxiety-like phenotype, but normal social interaction.
5.3 NRG1–Ig+/− Hypomorphs
NRG1–Ig+/− mice were normal in open-field and wheel rotation tests. They were impaired in latent inhibition and showed suppression of open-field and running wheel activity by clozapine (99).
5.4 NRG1–CRD+/− Hypomorphs
NRG1–CRD+/− mice appeared normal in open-field and Y-maze tests but were impaired in prepulse inhibition, latent inhibition and working memory (100).
5.5 NRG1type1-tg Hypermorphs
5.6 Neonatal Injection of NRG1 Ectodomain
The extracellular domain of NRG1 could go through the blood–brain barrier after subcutaneous injection in neonatal mice. These mice showed impaired prepulse inhibition, latent inhibition and social interaction at adult stage (103). After being treated with methamphetamine, the mice showed hyperactive in open field test (103).
5.7 ErbB2+/− and ErbB3+/− Hypomorphs
Because null mutations of ErbB2 and ErbB3 are embryonically lethal, only heterozygous mutant mice have been analyzed before NRG1 was identified as a schizophrenia susceptibility gene (91). In a limited set of behavioral paradigms, they appeared to be normal in open-field exploratory activity, spontaneous alternation, and rotarod performance. It was unknown whether they are impaired in prepulse inhibition or social interaction.
5.8 ErbB4 Mutant Mice
Expression of an ErbB4 transgene in the heart (i.e., in ErbB4−/−;ht+ mice) prevents premature death and allows for behavioral analysis. ErbB4−/−;ht+ mice are hyperactive but show no deficits in prepulse inhibition (69) (Table 12.2). When ErbB4 is specifically mutated in neurons by crossing the floxed ErbB4 mice with Nestin Cre mice, nestin–ErbB4−/− mice appeared to be impaired in long-term spatial memory. They were hyperactive during postnatal days 19–20 but became hypoactivity at adult stage (104). Transgenic mice were generated to express dominant negative-ErbB4 (DN-ErbB4), a truncated ErbB4 without the entire intracellular C-terminal region, specifically in oligodendrocytes. DN-ErbB4 was shown to block NRG1 signaling by competing with endogenous ErbB proteins. DN-ErbB4 transgenic mice exhibit hypoactivity, with increased anxiety-like behavior (105). When ErbB2 and ErbB4 are specifically mutated in CNS by crossing the floxed ErbB2, ErbB4 mice with the hGFAP Cre mice, hGFAP–ErbB2−/−/ErbB4−/− mice showed normal in open field test but reduced prepulse inhibition which could be rescued by clozapine (59).
Table 12.2
ErbB4 mutant mice phenotypes
ErbB4−/−;ht+ | Nestin–ErbB4−/− | DN–ErbB4 | hGFAP–ErbB2/4−/− | PV–ErbB4−/− | |
---|---|---|---|---|---|
Locomotor activity | |||||
Open-field test | ↑ (69) | ↑ (104) | ↓ (105) | = (59) | ↑ (63) |
Executive function | |||||
PPI | = (69) | n.d. | n.d. | ↓ (59) | ↓ (63) |
Short term memory | |||||
Working memory | n.d. | n.d. | n.d. | n.d. | ↓ (63) |
Long-term memory | |||||
Water maze test | n.d. | ↓ (104) | n.d. | n.d. | n.d. |
Clozapine | n.d. | n.d. | n.d. | Rescued PPI (59) | n.d. |
5.9 PV–ErbB4−/− Mutant Mice
To study the role of ErbB4 in PV-positive interneurons, we recently ablated ErbB4 specifically in PV-positive interneurons by crossing the floxed ErbB4 mice with the PV Cre mice (named thereafter PV–ErbB4−/− mice) (63). As described above, this mutation prevented NRG1 from promoting activity-dependent GABA release and from suppressing the activity of pyramidal neurons. PV–ErbB4−/− mutant mice do not exhibit differences in weight, whisker number, and rectal temperature in comparison with control littermates, and there were no significant differences in motor coordination. The mutant mice are able to adapt to a novel environment, but they exhibit increased locomotor activity and stereotypical activity.
To examine whether the loss of ErbB4 in PV-positive interneurons resulted in cognitive deficits, PV–ErbB4−/− mice were trained to retrieve food pellets from the end of each arm in an eight-arm radial maze (63). They were scored for the total wrong entries (repeated entries into a previously visited arm or omission of an arm) and the total time to retrieve all pellets. Both mutant and control mice are able to learn to retrieve food pellets by reducing wrong entries and exploration time. However, PV–ErbB4−/− mice showed an increased number of total errors, suggesting possible deficits in working memory. Because the mutant mice are hyperactive, the increase in total errors could be due to random hyperactivity. To exclude this possibility, we monitored correct entries during the first four entries, eliminating effects of total travel distance and time (106). The percentage of correct entries within the first four entries is significantly lower in mutant mice in comparison with controls. Together these observations provide strong evidence for impaired working memory in PV–ErbB4−/− mice. Finally, although mutant mice produced similar startle responses as the control littermates, prepulse inhibition was significantly lower in PV–ErbB4−/− mice. Remarkably, acute treatment with low-dose diazepam, a GABA enhancer, was able to ameliorate disrupted PPI in PV–ErbB4−/− mice, providing evidence for impaired GABAergic transmission.
These observations could be interpreted as evidence that PV-positive interneurons are a major cellular target of abnormal NRG1/ErbB4 signaling in schizophrenia. They are in line with the idea that disrupted NRG1 signaling may cause imbalance in neuronal activity in the brain, providing insight into possible pathogenic mechanisms of schizophrenia. Due to late onset of the PV promoter activity, levels of ErbB4 and function of NRG1 in PV-positive interneurons are not expected to alter until almost 2 weeks after birth. These behavioral deficits are in PV–ErbB4−/− mice likely to result from abnormal neurotransmission or synaptic plasticity. In light of higher expression of NRG1 and ErbB4 in schizophrenic PFC, we believe that these results support a working hypothesis that increased NRG1 activity promotes GABA release and thus suppresses the activity of pyramidal neurons, providing an underpinning mechanism of hypofunction of glutamergic pathways in schizophrenia. Finally, unlike NRG1 and ErbB4 null mutant mice that often die prematurely, PV–ErbB4−/− mice survive into adulthood and thus could serve as a valuable model to study schizophrenia and relevant brain disorders.
6 Conclusions
While recent advances in understanding NRG1 function are exciting, many critical questions remain to be addressed. Due to limited availability of specific antibodies against NRG1 isoforms and different ErbB kinases, little is known when and where they are expressed. NRG1 and ErbB4 are found increased expression in schizophrenic patients. Yet, studies in the past have been carried out mainly in mutant mice where the expression of NRG1 isoforms or ErbB4 was reduced. Moreover, the schizophrenia-like phenotypes in NRG1 and ErbB4 mutant or transgenic mice could result from the defects in neural development as well as in neurotransmission or synaptic plasticity. Therefore, mouse models whose overexpression or mutant of NRG1 and ErbB4 are controlled in space and time could be extremely valuable in understanding how the alternation of NRG1 ErbB4 signaling contributes to schizophrenia pathology. Such models should also be invaluable for the development of therapeutic intervention of this devastating disorder.
6.1 What Is a Good Model for Schizophrenia?
An animal model of schizophrenia needs face validity, constructive validity, and predictive validity. Face validity means that the animal model should resemble the symptoms from schizophrenic patient. Because the delusions and hallucinations could not be measured in animal models, the sensory-motor gating, attention, learning and memory, psychomotor excitement, and social interactions can be evaluated in animal models. Psychomotor excitement and sensory-motor gating are the most wildly used criteria for evaluating NRG1 and ErbB4 mutant mouse models, because it is not usual for a mouse model to match all the phenotypes. What is more, general health should also be observed, including their water and food consumption, body weight, and circadian behavior. It will be more convincing for a mouse model to have behavioral defect only after late adolescence and early adulthood, when the schizophrenic symptoms usually appears in patients.
Constructive validity requests that the animal model should have similar pathophysiological mechanism as the schizophrenic patients. Since many genetic and environmental factors might be involved in the etiology of schizophrenia, it is impossible for one schizophrenic model to mimic all the factors, but the mouse model should mimic the underlying mechanism at different levels, including molecular, cellular and brain circuitry levels. Several hypothesizes for the pathogenesis of schizophrenia were proposed, including abnormal development, hyperdopaminergic, hypoglutamatergic and hypo-GABAergic hypothesis (107). Further studies need to investigate how the NRG1/ErbB4 is involved in the balance of the neurotransmissions among GABA, glutamate and dopamine (108).
Predictive validity means that the animal model should have similar efficacy for the treatment drugs for schizophrenia and can be used to explore new drugs for patients. Atypical antipsychotics such as clozapine are the most commonly used drugs for evaluating the mouse models. Since the downstream pathways of NRG1/ErbB4 signaling are not fully understood, whether any drugs that target these pathways can rescue the schizophrenia-like phenotypes in the NRG1 or ErbB4 mutant mice needs to be further tested.
References
1.
Kessler, R. C., Birnbaum, H., Demler, O., Falloon, I. R., Gagnon, E., Guyer, M., Howes, M. J., Kendler, K. S., Shi, L., Walters, E., and Wu, E. Q. (2005) The prevalence and correlates of nonaffective psychosis in the National Comorbidity Survey Replication (NCS-R), Biol Psychiatry 58, 668–676.PubMed
2.
Wu, E. Q., Shi, L., Birnbaum, H., Hudson, T., and Kessler, R. (2006) Annual prevalence of diagnosed schizophrenia in the USA: a claims data analysis approach, Psychol Med 36, 1535–1540.PubMed
3.
Agid, O., Shapira, B., Zislin, J., Ritsner, M., Hanin, B., Murad, H., Troudart, T., Bloch, M., Heresco-Levy, U., and Lerer, B. (1999) Environment and vulnerability to major psychiatric illness: a case control study of early parental loss in major depression, bipolar disorder and schizophrenia, Mol Psychiatry 4, 163–172.PubMed
4.
Chapman, D. P., Whitfield, C. L., Felitti, V. J., Dube, S. R., Edwards, V. J., and Anda, R. F. (2004) Adverse childhood experiences and the risk of depressive disorders in adulthood, J Affect Disord 82, 217–225.PubMed
5.
McAllister, T. W., and Summerall, L. (2003) Genetic polymorphisms in the expression and treatment of neuropsychiatric disorders, Curr Psychiatry Rep 5, 400–409.PubMed
6.
European Network of Schizophrenia Networks for the Study of Gene-Environment Interactions. (2008) Schizophrenia aetiology: do gene–environment interactions hold the key?, Schizophr Res 102, 21–26.
7.
Weinberger, D. R. (1987) Implications of normal brain development for the pathogenesis of schizophrenia, Arch Gen Psychiatry 44, 660–669.PubMed
8.
Pilowsky, L. S., Kerwin, R. W., and Murray, R. M. (1993) Schizophrenia: a neurodevelopmental perspective, Neuropsychopharmacology 9, 83–91.PubMed
9.
Powell, C. M., and Miyakawa, T. (2006) Schizophrenia-relevant behavioral testing in rodent models: a uniquely human disorder?, Biol Psychiatry 59, 1198–1207.PubMed
10.
Ross, C. A., Margolis, R. L., Reading, S. A., Pletnikov, M., and Coyle, J. T. (2006) Neurobiology of schizophrenia, Neuron 52, 139–153.PubMed
11.
Lewis, D. A., and Moghaddam, B. (2006) Cognitive dysfunction in schizophrenia: convergence of gamma-aminobutyric acid and glutamate alterations, Arch Neurol 63, 1372–1376.PubMed
12.
Tsai, G., and Coyle, J. T. (2002) Glutamatergic mechanisms in schizophrenia, Annu Rev Pharmacol Toxicol 42, 165–179.PubMed
13.
Roberts, E. (1972) Prospects for research on schizophrenia. An hypotheses suggesting that there is a defect in the GABA system in schizophrenia, Neurosci Res Program Bull 10, 468–482.PubMed
14.
Javitt, D. C., and Zukin, S. R. (1991) Recent advances in the phencyclidine model of schizophrenia, Am J Psychiatry 148, 1301–1308.PubMed
15.
Fauman, B., Aldinger, G., Fauman, M., and Rosen, P. (1976) Psychiatric sequelae of phencyclidine abuse, Clin Toxicol 9, 529–538.PubMed
< div class='tao-gold-member'>
Only gold members can continue reading. Log In or Register a > to continue
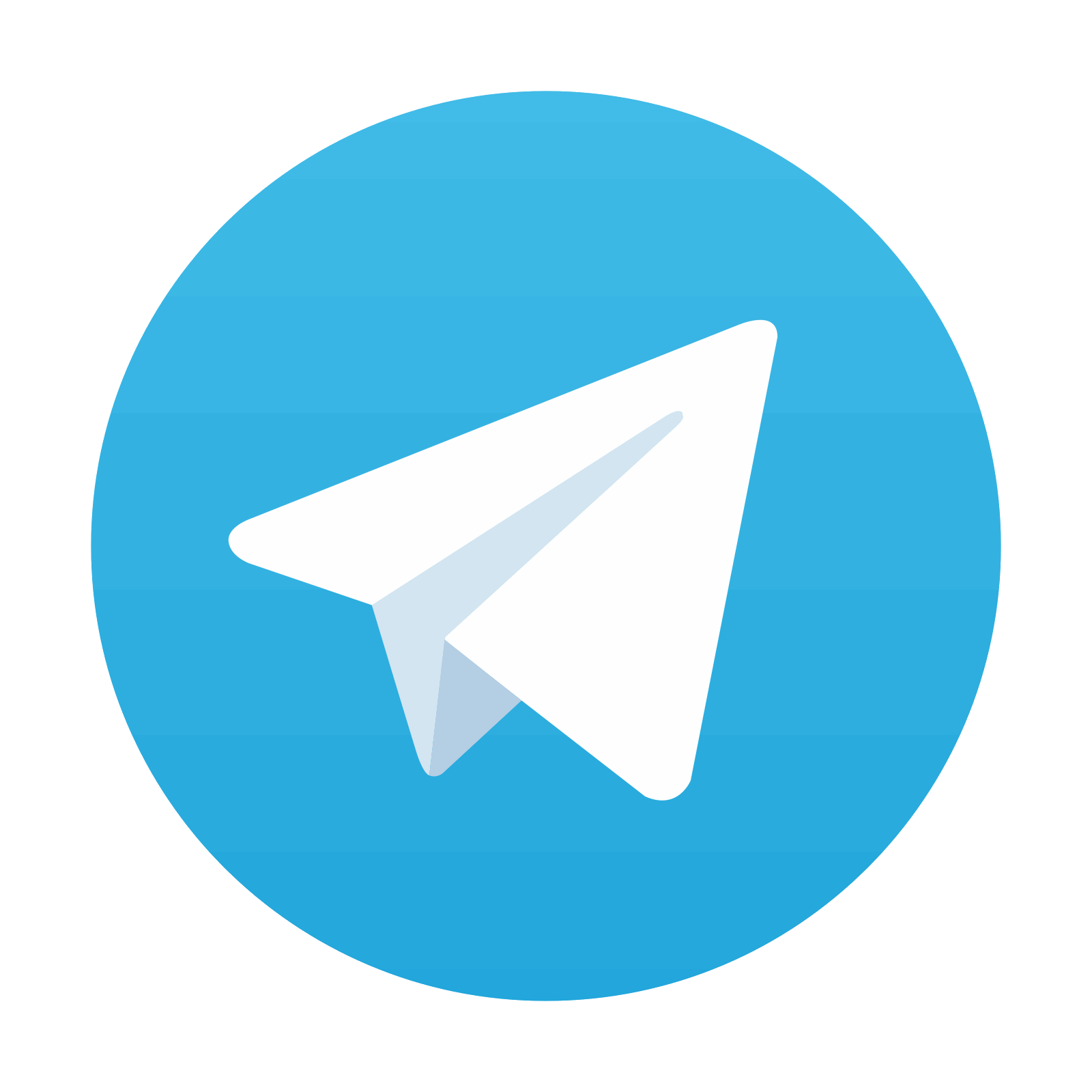
Stay updated, free articles. Join our Telegram channel

Full access? Get Clinical Tree
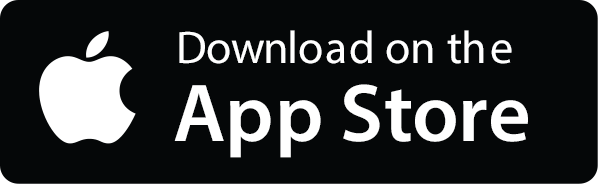
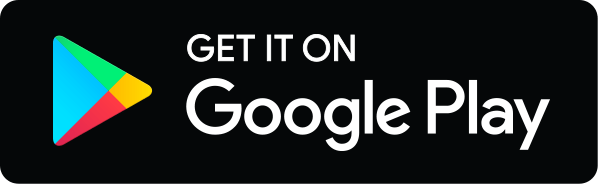