For instance, the Bayesian analysis of the mtDNA data combined partial sequences from three regions: D loop, 12S rRNA, and Cytb (2074 bp total; Figure 19.1a) from Genbank and recently acquired sequences (Steiner & Ryder, 2011). The analysis of the mitochondrial data set resulted in a lack of phylogenetic resolution and poor node support by both bootstrap (BP) and posterior probability (PP) values. The mitochondrial tree is characterized by a polytomy composed of the mountain zebra, a cluster of Burchell’s and Grevy’s zebras, and a third cluster formed by asses and caballines. In the latter, the African wild ass is basal relative to two clades, the caballines and Asiatic asses (kiang and the Asiatic wild ass). Well-supported nodes include the cluster of caballines, Asiatic asses, and the monophyly of Equidae (BP = 100, PP = 1.00).
The Bayesian analysis of the nuclear DNA comprised a large data set consisting of partial sequences of 20 genes (15791 bp) from coding and non-coding areas of the genome (Figure 19.1b; Steiner et al., 2012). The nuclear phylogenetic tree reveals a well-resolved and relatively well-supported topology for most nodes, identifying three groups of equids as proposed before: caballines, zebras, and asses (African and Asiatic). Specifically, the deepest node in the tree corresponds to the split between caballine and non-caballine equids. Within the asses group, the African wild ass appears basal and sister to Asiatic asses, while within zebras, the Burchell’s zebra (E. burchelli) diverged first relative to mountain (E. zebra) and Grevy’s (E. grevyi) zebras.
A more extensive analysis concatenated mitochondrial and nuclear genes into a single dataset (23 genes, 17865 bp; Figure 19.1c). Combining genes makes sense, as analyses of data consisting of a single or small number of genes have a high probability of supporting conflicting phylogenies. In contrast, analyses of concatenated genes have yielded fully resolved species trees with maximum support (Rokas et al., 2003). The combined Bayesian analysis showed similar results relative to the nuclear dataset, separating caballines from asses and zebras. However, in the zebra cluster, mountain zebra appears basal and sister to Burchell’s (E. burchelli) and Grevy’s (E. grevyi) zebras. The monophyly of all species of asses is not well supported by MP and ML approaches, but the relationship between kiang and wild Asiatic asses is resolved.
Lower nodal support in the combined data likely reflects phylogenetic incongruence between the mitochondrial and nuclear datasets. These discrepancies are likely due to differences in gene properties, including base composition and substitutions rate, or even substitution saturation in the case of mitochondrial genes (Brown et al., 1979; Galtier & Gouy, 1995; Perna & Kocher, 1995). In addition, and perhaps more important in the evolution of species, are the biological processes that occurred during speciation, such as incomplete genetic lineage sorting, genetic introgression, or hybridization among species (Rokas & Carroll, 2006; Hallström & Janke, 2008). For example, before closely related species become completely genetically isolated, they can hybridize with each other. This poses a problem for phylogenetic analysis, because the species history can be obscured by introgression or the incorporation of genes from one species into the gene pool of another.
In conclusion, these phylogenetic analyses seem to highlight the value of nuclear data as a complementary source of genetic information for inferring equid relationships relative to mtDNA, supporting (1) the taxonomic division of caballines and non-caballines initially proposed by morphological data (Forsten, 1992) and other molecular studies (Lowenstein & Ryder, 1985; George & Ryder, 1986; Oakenfull & Clegg, 1998; Krüger et al., 2005); and (2) the phylogenetic position of the African wild ass (E. asinus) as sister taxon to Asiatic asses as previously predicted by cranial characters (Bennet, 1980), immunological protein assays (Lowestein & Ryder 1985), Y chromosome sequences, and microsatellite data (Wallner et al., 2003; Krüger et al., 2005). This comprehensive phylogenetic framework provides a new outlook on the evolution of extant equids, allowing other issues related to the evolution of extinct equid species to be addressed, including the history of horse domestication, the origin of horse breeds, and their morphological and physiological adaptations.
Przewalski’s Horse and Horse Domestication
Przewalski’s Horse (Equus przewalskii) is an endangered species that is considered to be the only true “wild” extant horse species. Przewalski’s Horse is the closest living relative to domestic horse (Equus caballus) and can be used to infer the history of horse domestication. Compared with domestic horse (2N = 64), Przewalski’s Horse (2N = 66) has an extra chromosome pair due to one Robertsonian translocation: either fission of domestic horse chromosome 5 or fusion of Przewalski’s Horse chromosomes 23 and 24 (Benirschke et al., 1965; Bowling & Ruvinsky, 2000; Myka et al., 2003; Yang et al., 2003; Ahrens & Stranszinger, 2005). In comparison, the chromosomal differences between domestic horses, asses, and zebras include numerous translocations, fusions, and inversions (Yang et al., 2004, Trifonov et al., 2008). The Przewalski’s horse is known to have the highest diploid chromosome number among horse species (Ryder et al., 1978).
Although this chromosomal difference genetically separates Przewalski’s horse from domestic horse, the two have been known to interbreed to produce fertile offspring (Short et al., 1974). In contrast, hybridizations between domestic horses and other related species such as donkeys usually result in viable but infertile offspring due to multiple chromosomal rearrangements that separate the species (Zong & Fan, 1989; Yang et al., 2004). Skull measurements often overlap between the Przewalski’s and domestic horses (Forsten, 1987; Eisenmann & Baylac 2000), but other skeletal features highlight differences in the morphology between the two horses (Sasaki et al., 1999).
Przewalski’s Horses probably once lived in a broader range throughout Europe and Asia, including the Dzungarian Basin, an area that is now part of Mongolia, Kazakhstan, and the Xinjiang-Uygur Autonomous Region of China (Ryder, 1993). By the mid-1960s, Przewalski’s Horse had become virtually extinct in the wild due to human activity; however, it had subsequently been bred in captivity and reintroduced into its natural habitat in parts of Mongolia and China (Ryder & Wedemeyer, 1982; Ryder, 1993; Bouman & Bouman 1994). The present-day Przewalski’s Horse population, consisting of more than 2,000 animals, originated from a mere 13 animals gathered at the turn of the nineteenth century, one of which was a domestic horse and the other a domestic/Przewalski’s Horse hybrid (Oakenfull & Ryder, 1998). Notably, the founders included only four Przewalski’s Horse females (Volf et al., 1991; Oakenfull & Ryder 1998). Later, additional introgression from domestic horse mares via their hybrid offspring occurred, but was not revealed until genetic studies were performed (Bowling et al., 2003).
The genetic relationship between Przewalski’s and domestic horses has been subject to a heated debate, and several questions remain unanswered: Is Przewalski’s Horse the direct ancestor of domestic horse? Or has Przewalski’s Horse branched out from the ancestral population that included the domestic horse genetic pool?
Previous studies have used different DNA markers to determine the genetic relationship between Przewalski’s and domestic horse and have resulted in contradictory results. Whereas some protein, microsatellite, and Y chromosome analyses have supported phylogenetic separation of the two taxa (Bowling et al., 2003; Wallner et al., 2003), the latest investigations of autosomal (Lau et al., 2009; Wade et al., 2009) and X chromosomal DNA (Lau et al., 2009) have not. For instance, our recent phylogenetic analysis of several autosomal and X chromosomal introns placed Przewalski’s Horses within the domestic horse clade (Lau et al., 2009). Similarly, phylogenetic separation between Przewalski’s versus domestic horses was not observed after typing ∼1,000 autosomal SNPs as part of the analysis of the horse genome (Wade et al., 2009). This led to the speculation that either E. przewalskii is very recently derived from E. caballus, or that the two horses intermixed considerably following divergence from a common ancestor (Wade et al., 2009). Because Przewalski’s Horses are the only truly wild horses existing today, they have alternatively been hypothesized to be the direct ancestors of domestic horses (Ryder et al., 1994).
Possible explanations for these findings are gene flow from domestic horse into the Przewalski’s Horse population, different effective population sizes of males and females, or an unequal ratio of female to male founders (Wallner et al., 2003). Only relatively short genomic regions (or a small number of sites) have been analyzed in the reports mentioned above, inflating statistical fluctuations. Moreover, it is also possible that these discrepancies in results are due to differences in sampling depending on whether the Przewalski’s Horses studied belong to the same mitochondrial lineage.
Studies of mtDNA of Przewalski’s Horse were limited to sequencing the D loop region (Ishida et al., 1995; Oakenfull & Ryder, 1998; Oakenfull et al., 2000). There are four maternal lineages in the current Przewalski’s Horse population (Ryder, 1994); however, sequencing of the mitochondrial D loop region showed only two mtDNA haplotypes in these lineages (Oakenfull & Ryder 1998). Moreover, other studies of mitochondrial DNA indicated that domestic horse and Przewalski’s Horse do not form separate clades (Ishida et al., 1995, Oakenfull & Ryder, 1998).
Recently, using the massively parallel sequencing technology, we determined the sequence of complete mtDNA genomes in all four surviving matrilines of Przewalski’s Horse and compared it to the completely sequenced domestic horse mtDNA genomes that are currently publicly available for six horse breeds (a Thoroughbred, Debao pony, Tibetan breed from Naqu, Tibetan breed from Deqin, Jeju breed, and Tibetan breed from Zhongdian), using the mitochondrial genome of the Somali wild ass as an outgroup (Goto et al., 2011). Three mitochondrial haplotypes were discovered in Przewlaski’s horse – two similar ones, haplotypes I/II, and one substantially divergent from the other two, haplotype III. Haplotypes I/II versus III did not cluster together on a phylogenetic tree, rejecting the monophyly of Przewalski’s Horse maternal lineages, and were estimated to split 0.117–0.186 million years ago, significantly preceding horse domestication, thought to occur only about 6,000 years ago (Goto et al., 2011; Outram et al., 2009).
The divergence of Przewalski’s Horse mtDNA haplotypes I/II and III poses an interesting question. The drastically different haplotypes could represent genetic polymorphism that existed in the ancestral population of Przewalski’s and domestic horses. As horse domestication is a relatively recent event, Przewalski’s Horses could have retained such ancestral polymorphism in their current population. In this case, sequencing mtDNA from ancient horse remains is expected to reveal haplotypes similar to the ones found here for Przewalski’s Horses. Second, one or both divergent mtDNA haplotypes could have been introduced from domestic to Przewalski’s Horses via interbreeding. In particular, two closely related haplotypes are very similar in sequence and cluster together with domestic horse haplotype(s) (Goto et al., 2011), and thus might have been acquired by Przewalski’s Horse through genetic introgression. Additional sequencing of mtDNA from modern domestic horses from Eurasia might identify mtDNA haplotypes similar in sequence to one or several Przewalski’s Horse haplotypes. A combination of these two scenarios (e.g., one divergent haplotype acquired via introgression while another one inherited from the ancestral horse population) is also possible.
Furthermore, as a part of the same study (Goto et al., 2011), we sequenced partial nuclear genomes of the four Przewalski’s Horses and analyzed these data together with the publicly available genomic sequences of the Thoroughbred domestic horse (Wade et al., 2009). In the phylogeny based on X chromosomal sequences, Przewalski’s and domestic horse lineages were intermixed, while in that built from autosomal sequences, Przewalski’s Horses lineages were monophyletic. The results indicate that Przewalski’s Horses have ancient polyphyletic origins and are not the direct progenitors of domestic horses. The data also suggest that Przewalski’s and domestic horse lineages diverged at least 0.117 million years ago, but since then have retained ancestral genetic polymorphism and/or experienced gene flow.
History of Horse Breeds
The different domestic breeds of horse are originally derived from a variety of wild populations distributed from Europe to Middle East. Breeds established prior to 1500 AD exhibit a pattern of geographic distribution and morphological stability that is the result of conservative breeding based on the “native broodmare” (Bennett & Hoffmann, 1999). In the last century, many horse populations have suffered severe decline throughout the world, when horses lost importance as instruments of transportation of mechanical power (Aberle & Distl, 2004).
The study of the genetic structure of different horse breeds has been essential in understanding the development of today’s breeds, and in establishing conservation programs for preserving the genetic variability still present in horse populations. The history of horse breeds and domestication has been investigated largely from mitochondrial and Y chromosome sequences, leading to conclusions about a single patriline (Lindgren et al., 2004) but numerous matrilines (Vila et al., 2001) incorporated into the genetic pool of domestic horses. This is consistent with the breeding plans of most horse breeds, in which one male is bred with many different females (McDonnell, 2005).
The sequencing and genetic analyses of mtDNA sequences, specifically the hypervariable region of the D loop, have been extensively used. Multiple studies have addressed sequence variation among maternal lineages of horse breeds, including the Lipizzan horses (Kavar et al., 2002), Mongolian (Li et al., 2008), German heavy draught (Aberle et al., 2007), Iberian (Royo et al., 2005), Thoroughbred (Hill et al., 2002; Bower et al., 2011), Italian breeds (Cozzi et al., 2004), and New World horses (Luis et al., 2006). In general, these studies document a diversity of hypervariable region haplotypes suggesting mixed origins of horse breeds with high maternal haplotype variation, even within individual breeds. Accordingly, the origin of single breeds is rather obscure, although multiple domestication events are suggested (Vila et al., 2001; Jansen et al., 2002).
New approaches have also been implemented in horse population analyses facilitated by the development of genomic tools for the domestic horse as model organism. Specifically, SNP genotyping has characterized within- and across-breed genetic structure by analyzing patterns of linkage disequilibrium (LD; Wade et al., 2009). These analyses revealed a moderate within-breed LD, with similar values shared among the majority of breeds (see also Chapter 7). Latter implicates that horses do not appear to have undergone a tight domestication bottleneck as observed for example in cows. In addition to that, major haplotypes are frequently shared among diverse populations.
Adaptation in Horse Breeds
Domestic horses extensively vary in their morphological and physiological features. For instance, the warmblood horses are lightweight, quick, and more excitable animals, mostly used for races and leisure sports. The Arabian breed is possibly the best representative of this group. Coldblooded horses, in contrast, are typically tall, heavy animals, such as the Shire, the Clydesdale, or the Friesian breeds. They have been predominantly developed to become very strong and resistant horses, being subsequently used in agriculture and traction-type tasks like goods transportation.
Relatively few molecular studies have addressed the genetic bases of such characteristic phenotypes among horse breeds and most of the studies to date have analyzed mitochondrial genes. Mitochondrial DNA is a candidate genome for this type of adaptive studies because of its vital role in energy metabolism through oxidative phosphorylation in eukaryotic cells (Fonseca et al., 2008). In addition, amino acid changes in mtDNA have shown to improve aerobic capacity and adaptation to thermal environments in some organisms (Mishmar et al., 2003; Jobson et al., 2004; Dalziel et al., 2006). Comparative studies in domestic horses by Xu et al. (2007) and Ning et al. (2010) investigated adaptation to high altitude in Tibetan and Chinese horses using whole mtDNA genomes and the candidate mitochondrial gene ND6, respectively. Both studies produced evidence for adaptive evolution in the ND6 gene in plateau horses, suggesting the potential influence of oxygen deficits and cold temperatures in animals living in high-altitude environments.
The genetic basis of morphological traits in horses has also been studied using genome-wide approaches. Gu et al. (2009) for example analyzed candidate genes involved in functional adaptations contributing to elite athletic phenotypes in Thoroughbred horses. They used microsatellite-based linkage mapping for identifying potential genes under selection. Among the athletic-performance candidate genes identified were mitochondrial proteins such as ACCS1, MTFR1, and PDK4. These results emphasize the importance of energy production genes in horse adaptations, and highlight the relevance of functional co-adaptation studies between mitochondrial and nuclear-encoded proteins for understanding how species maintain optimal metabolic fitness (see Blier et al., 2001; Grossman et al., 2004; Shen et al., 2010).
Future surveys including whole mitochondrial genomes and genome-wide association studies appear as the most appropriate strategies for dissecting the genetic basis of morphological and physiological traits in horse breeds. The precipitous decline in genome sequencing costs and the advances in bioinformatics and comparative genomics, in combination with data on domestic horse pedigrees and phenotypes will make it possible. Genomic approaches will surely lead to the identification of mutations in genes related to morphology, metabolism, and diseases susceptibility in horses.
References
Aberle, K., & Distl, O. (2004). Domestication of the horse: Results based on microsatellite and mitochondrial DNA markers. Archiv Tierzucht, Dummerstorf, 47(6), 517–535.
Aberle, K., Hamann, H., Drogemuller, C., & Distl, O. (2007). Phylogenetic relationships of German heavy draught horse breeds inferred from mitochondrial DNA D-loop variation. Journal of Animal Breeding and Genetics, 124, 94–100.
Ahrens, E., & Stranzinger, G. (2005). Comparative chromosomal studies of E. caballus (ECA) and E. przewalskii (EPR) in a female F1 hybrid. Journal of Animal Breeding and Genetics = Zeitschrift fur Tierzuchtung und Zuchtungsbiologie, 122(Suppl. 1), 97–102.
Benirschke, K., Malouf, N., Low, R. J., & Heck, H. (1965). Chromosome Complement: Differences between Equus Caballus and Equus Przewalskii, Poliakoff. Science, 148, 382–383.
Bennett, D. (1980). Stripes do not a zebra make, Part I: A cladistic analysis of Equus. Systematic Zoology, 29(3), 272–287.
Bennett, D., & Hoffmann, R. (1999). Equus caballus. Mammalian Species, 628, 1–14.
Bernor, R., Armour-Chelu, M., Gilbert, H., Kaiser, T., & Schulz, E. (2010). Equidae. In L. Werdelin & B. Sanders (eds.), Cenozoic mammals of Africa (pp. 685–721). Berkeley: University of California Press.
Blier, P., Dufresne, F., & Burton, R. (2001). Natural selection and the evolution of mtDNA-encoded peptides: Evidence for intergenomic co-adaptation. Trends in Genetics, 17(7), 400–406.
Bouman, I., & Bouman, J. (1994). The history of the Przewalski’s horse. In L. Boyd & K. A. Houpt (eds.), Przewalski’s Horse: The History and Biology of an Endangered Species (pp. 5–38). Albany: State University of New York Press.
Bower, M., Campana, M., Whitten, M., Edwards, C., Jones, H. et al. (2011). The cosmopolitan maternal heritage of the Thoroughbred racehorse breed shows a significant contribution from British and Irish native mares. Biology Letters, 7, 316–320.
Bowling, A., & Ruvinsky, A. (eds.) (2000). Genetic aspects of domestication, breeds, and their origins. Oxon: CABI.
Bowling, A. T., Zimmermann, W., Ryder, O., Penado, C., Peto, S. et al. (2003). Genetic variation in Przewalski’s horses, with special focus on the last wild caught mare, 231 Orlitza III. Cytogenetic and Genome Research 102(1–4), 226–234.
Brown, W., George, M., & Wilson, A. (1979). Rapid evolution of animal mitochondrial DNA. Proceedings of the National Academy of Sciences USA, 76(4), 1967–1971.
Clegg, J. (1974). Horse hemoglobin polymorphism. Annals of the New York Academy of Sciences, 241, 61–69.
Cozzi, M., Strillacci, M. G., Valiati, P., Bighignoli, B., Cancedda, M., & Zanotti, M. (2004). Mitochondrial D-loop sequence variation among Italian horse breeds. Genetics Selection Evolution, 36, 663–672.
Dalziel, A., Moyes, C., Fredriksson, E., & Lougheed, S. (2006). Molecular evolution of cytochrome c oxidase in high-performance fish (teleostei: Scombroidei). Journal of Molecular Evolution, 62(3), 319–331.
Eisenmann, V. (1979). Caracteres evolutifs et phylogeny du genre Equus (Mammalia, Perissodactyla). Comptes Rendus de l’Academie des Sciences, Paris, ser D, 288, 497–500.
Eisenmann, V., & Baylac, M. (2000). Extant and fossil Equus (Mammalia, Perissodactyla) skulls: A morphometric definition of the subgenus Equus. Zoologica Scripta, 29(2), 89–100.
Fonseca, R., Johnson, W., O’Brien, S., Ramos, M., & Antunes, A. (2008). The adaptive evolution of the mammalian mitochondrial genome. BMC Genomics, 9, 119.
Forsten, A. (1987). A comment on equid craniology. Mammalia, 51(3), 455–467.
Forsten, A. (1992). Mitochondrial-DNA time-table and the evolution of Equus: Comparison of molecular and paleontological evidence. Annales Zoologici Fennici, 28, 301–309.
Galtier, N., & Gouy, M. (1995). Inferring phylogenies from DNA sequences of unequal base compositions. Proceedings of the National Academy of Science USA, 92, 11317–11321.
George, J. M., & Ryder, O. (1986). Mitochondrial DNA evolution in the genus Equus. Molecular Biology Evolution, 3(6), 535–546.
Goto, H., Ryder, O. A., Fisher, A. R., Schultz, B., Kosakovsky Pond, S. A. et al. (2011). A massively parallel sequencing approach uncovers ancient origins and high genetic variability of endangered Przewalski’s horses. Genome and Biology of Evolution, 3, 1096–1106.
Grossman, L., Wildman, D., Schmidt, T., & Goodman, M. (2004). Accelerated evolution of the electron transport chain in anthropoid primates. Trends in Genetics, 20(11), 578–585.
Gu, J., Park, S., Katz, L., Sulimova, G., MacHugh, D., & Hill, E. (2009). A genome scan for positive selection in Thoroughbred horses. PloS One, 4(6), e5767.
Hallström, B., & Janke, A. (2008). Resolution among major placental mammal interordinal relationships with genome data imply that speciation influenced their earliest radiations. BMC Evolutionary Biology, 8(162), 162–174.
Harris, A., & Porter, L. (1980). Late Pleistocene horses of Dry Cave, Eddy County, New Mexico. Journal of Mammalogy, 61, 46–65.
Hill, E., Bradley, D., Al-Barody, M., Ertugrul, O., Splan, R. et al. (2002). History and integrity of Thoroughbred dam lines revealed in equine mtDNA variation. Animal Genetics, 33, 287–294.
Hulbert, R. J. (1996). The ancestry of the horse. In S. Olsen (ed.), Horses through time (pp. 11–34). Boulder, CO: Roberts Rinchart.
Ishida, N., Oyunsuren, T., Mashima, S., Mukoyama, H., & Saitou, N. (1995). Mitochondrial DNA sequences of various species of the genus Equus with special reference to the phylogenetic relationship between Przewalskii’s wild horse and domestic horse. Journal of Molecular Evolution, 41, 180–188.
Jansen, T., Forster, P., Levine, M. A., Oelke, H., Hurles, M. et al. (2002). Mitochondrial DNA and the origins of the domestic horse. Proceedings of the National Academy of Science USA, 99(16), 10905–10910.
Jobson, R., Nielsen, R., Laakkonen, L., Wikstrom, M., & Albert, V. (2004). Adaptive evolution of cytochrome c oxidase: Infrastructure for a carnivorous plant radiation. Proceedings of the National Academy of Science USA, 101(52), 18064–18068.
Kaminski, M. (1979). The biochemical evolution of the horse. Comparative Biochemistry and Physiology, 63B, 175–178.
Kavar, T., Brem, G., Habe, F., Solkner, J., & Dovc, P. (2002). History of Lipizzan horse maternal lines as revealed by mtDNA analysis. Genetics Selection Evolution, 34, 635–648.
Krüger, K., Gaillard, C., Stranzinger, G., & Rieder, S. (2005). Phylogenetic analysis and species allocation of individual equids using microsatellite data. Journal of Animal Breeding and Genetics, 122(1), 78–86.
Lau, A. N., Peng, L., Goto, H., Chemnick, L., Ryder, O. A., & Makova, K. D. (2009). Horse domestication and conservation genetics of Przewalski’s horse inferred from sex chromosomal and autosomal sequences. Molecular Biology and Evolution, 26(1), 199–208.
Li, J., Shi, Y., Fan, C., & Manglai, D. (2008). mtDNA diversity and origin of Chinese Mongolian horses. Asian-Australasian Journal of Animal Sciences, 21(12), 1696–1702.
Lindgren, G., Backstrom, N., Swinburne, J., Hellborg, L., Heinarsson, A. et al. (2004). Limited number of patrilines in horse domestication. Nature Genetics, 36, 335–336.
Lindsay, E., Opdyke, N., & Johnson, N. (1980). Pliocene dispersal of the horse Equus and late Cenozoic mammalian dispersal events. Nature, 287, 135–138.
Lowenstein, J., & Ryder, O. (1985). Immunological systematics of the extinct quagga (Equidae). Experientia, 41, 1192–1193.
Luis, C., Bastos-Silveira, C., Cothran, E., & Oom, M. (2006). Iberian origins of new world horse breeds. Journal of Heredity, 97(2), 107–113.
MacFadden, B. (2005). Fossil horses – evidence for evolution. Science, 307, 1728–1730.
McDonnell, S. (2005). Understanding your horse’s behavior. Lexington, KY: Blood-Horse Publications.
Mishmar, D., Ruiz-Pesini, E., Golik, P., Macaulay, V., Clark, A. et al. (2003). Natural selection shaped regional mtDNA variation in humans. Proceedings of the National Academy of Science USA, 100(1), 171–176.
Myka, J. L., Lear, T. L., Houck, M. L., Ryder, O. A., & Bailey, E. (2003). FISH analysis comparing genome organization in the domestic horse (Equus caballus) to that of the Mongolian wild horse (E. przewalskii). Cytogenetic and Genome Research, 102(1–4), 222–225.
Ning, T., Xiao, H., Li, J., Hua, S., & Zhang, Y. (2010). Adaptive evolution of the mitochondrial ND6 gene in the domestic horse. Genetics and Molecular Research, 9(1), 144–150.
Oakenfull, E., & Clegg, J. (1998). Phylogenetic relationships within the Genus Equus and the Evolution of a and b globin genes. Journal of Molecular Evolution, 47, 772–783.
Oakenfull, E., Lim, H., & Ryder, O. (2000). A survey of equid mitochondrial DNA: Implications for the evolution, genetic diversity and conservation of Equus. Conservation Genetics, 1, 341–355.
Oakenfull, E. A., & Ryder, O. A. (1998). Mitochondrial control region and 12S rRNA variation in Przewalski’s horse (Equus przewalskii). Animal Genetics, 29(6), 456–459.
Outram, A. K., Stear, N. A., Bendrey, R., Olsen, S., Kasparov, A. et al. (2009). The earliest horse harnessing and milking. Science, 323(5919), 1332–1335.
Perna, N., & Kocher, T. (1995). Patterns of nucleotide composition at fourfold degenerate sites of animal mitochondrial genomes. Journal of Molecular Evolution, 41, 353–358.
Rokas, A., & Carroll, S. (2006). Bushes in the tree of life. PLoS Biology 4, e352.
Rokas, A., Williams, B., King, N., & Carroll, S. (2003). Genome-scale approaches to resolving incongruence in molecular phylogenies. Nature, 425, 798–804.
Royo, L., Alvarez, I., Beja-Pereira, A., Molina, A., Fernandez, I. et al. (2005). The origins of Iberian horses assessed via mitochondrial DNA. Journal of Heredity, 96(6), 663–669.
Ryder, O. (1994). Genetic studies of Przewalski’s horses and their impact on conservation. In L. Boyd & K. A. Houpt (eds.), Przewalski’s horse: The history and biology of an endangered species (pp. 75–92). Albany: The State University of New York Press.
Ryder, O. A. (1993). Przewalski’s horse: Prospects for reintroduction into the wild. Conservation Biology, 7(1), 13–19.
Ryder, O. A., Epel, N. C., & Benirschke, K. (1978). Chromosome banding studies of the Equidae. Cytogenetics and Cell Genetics, 20(1–6), 332–350.
Ryder, O. A., & Wedemeyer, E. A. (1982). A cooperative breeding programme for the Mongolian wild horse Equus przewalskii in the United States. Biological Conservation, 22(4), 259–271.
Sasaki, M., Endo, H., Yamagiwa, D., Yamamoto, M., Arishima, K., & Hayashi, Y. (1999). Morphological character of the shoulder and leg skeleton in Przewalski’s horse (Equus przewalskii). Annals of Anatomy, 181(4), 403–407.
Shen, Y.-Y., Liang, L., Zhu, Z.-H., Zhou, W.-P., Irwin, D., & Zhang, Y.-P. (2010). Adaptive evolution of energy metaboslim genes and the origin of flight in bats. Proceedings of the National Academy of Science USA, 107(19), 8666–8671.
Short, R. V., Chandley, A. C., Jones, R. C., & Allen, W. R. (1974). Meiosis in interspecific equine hybrids. Cytogenetic and Genome Research, 13(5), 465–478.
Steiner, C. C., Houck, M. L., & Ryder, O. A. (2011). Species identification and chromosome variation of captive two-toed sloths. Zoo Biology 30, 623–635.
Steiner, C. C., Mitelberg, A., Tursi, R., & Ryder, O. A. (2012). Molecular phylogeny of extant equids and effects of ancestral polymorphism in resolving species-level phylogenies. Molecular Phylogenetics and Evolution 65, 573–581.
Trifonov, V. A., Stanyon, R., Nesterenko, A. I., Fu, B., Perelman, P. L. et al. (2008). Multidirectional cross-species painting illuminates the history of karyotypic evolution in Perissodactyla. Chromosome Research, 16, 89–107.
Vila, C., Leonard, J., Gotherstrom, A., Marklund, S., Sandberg, K. et al. (2001). Widespread origins of domestic horse lineages. Science, 291, 474–477.
Volf, J., Kus, E., & Prokopovaá, L. (1991). General studbook of the Przewalski horse. Prague: Zoological Garden.
Wade, C. M., Giulotto, E., Sigurdsson, S., Zoli, M., Gnerre, S. et al. (2009). Genome sequence, comparative analysis, and population genetics of the domestic horse. Science, 326(5954), 865–867.
Wallner, B., Brem, G., Muller, M., & Achmann, R. (2003). Fixed nucleotide differences on the Y chromosome indicate clear divergence between Equus przewalskii and Equus caballus. Animal Genetics, 34, 453–456.
Xu, S., Luosang, J., Hua, S., He, J., Ciren, A. et al. (2007). High altitude adaptation and phylogenetic analysis of Tibetan horse based on the mitochondrial genome. Journal of Genetics and Genomics, 34(8), 720–729.
Yang, F., Fu, B., O’Brien, P. C., Nie, W., Ryder, O. A., & Ferguson-Smith, M. A. (2004). Refined genome-wide comparative map of the domestic horse, donkey and human based on cross-species chromosome painting: insight into the occasional fertility of mules. Chromosome Research, 12(1), 65–76.
Yang, F., Fu, B., O’Brien, P. C., Robinson, T. J., Ryder, O. A., & Ferguson-Smith, M. A. (2003). Karyotypic relationships of horses and zebras: Results of cross-species chromosome painting. Cytogenetic and Genome Research, 102(1–4), 235–243.
Zong, E., & Fan, G. (1989). The variety of sterility and gradual progression to fertility in hybrids of the horse and donkey. Heredity, 62(Pt. 3), 393–406.
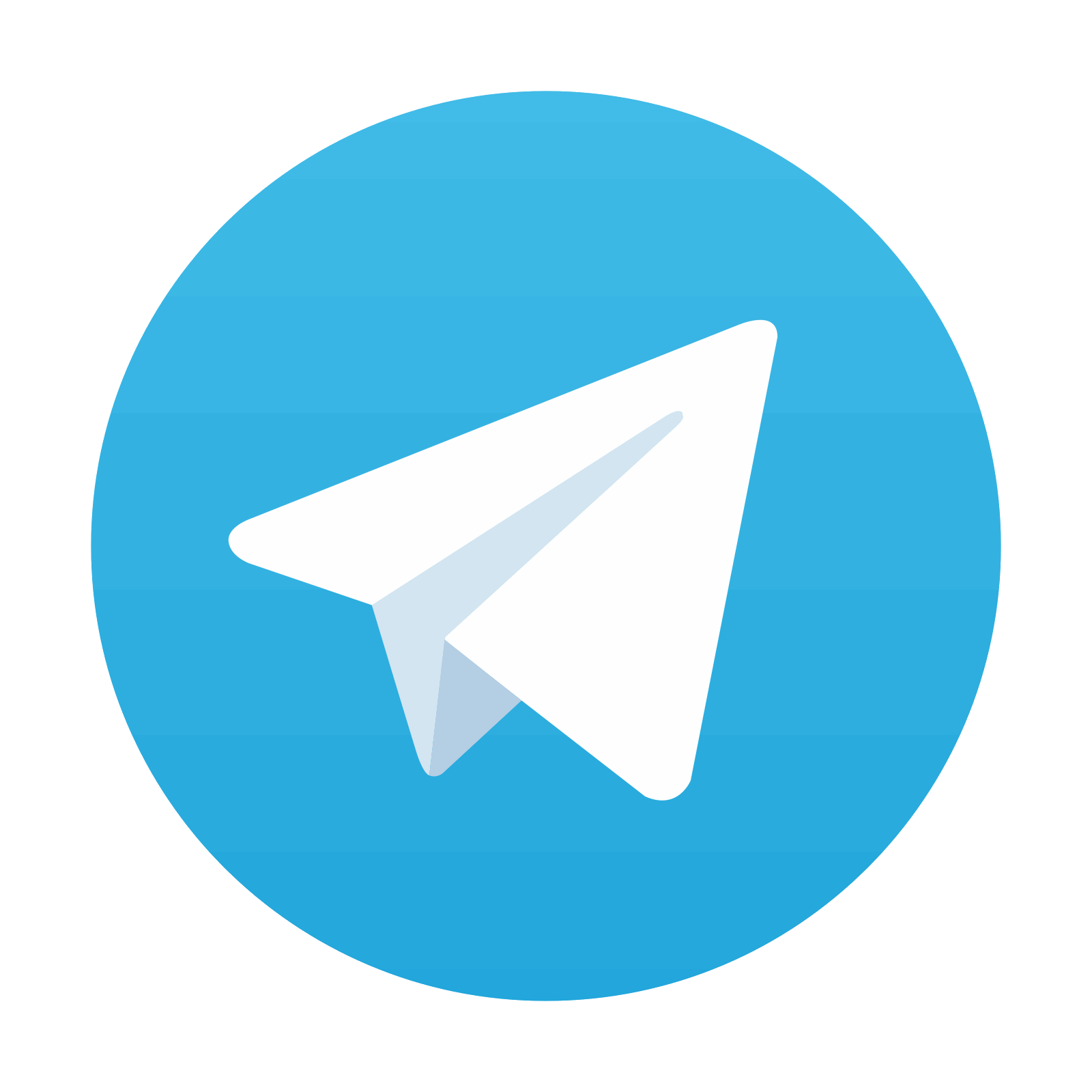
Stay updated, free articles. Join our Telegram channel

Full access? Get Clinical Tree
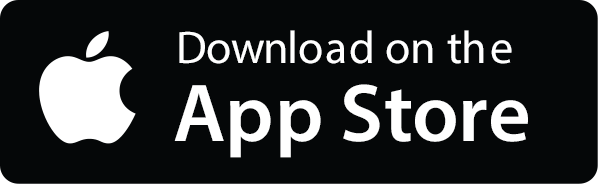
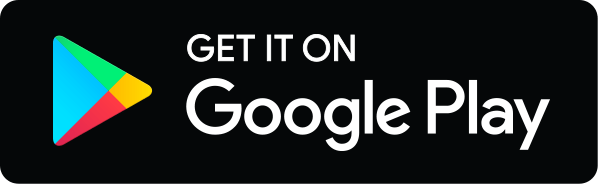