Chapter 15 Miscellaneous Infectious Diseases
CLOSTRIDIAL MYOSITIS
Etiology
C. septicum and C. perfringens are normal inhabitants of the gastrointestinal tract of most warm-blooded animals. Therefore contamination or inoculation of muscle with these organisms is by exogenous routes (Figure 15-1). Soil and feces may contain C. septicum or C. perfringens. C. septicum has been identified specifically as the cause of malignant edema, whereas C. chauvoei infections are referred to as “blackleg.” It probably is easier to refer to all clostridial infections as clostridial myositis because clinical differentiation of the species involved is sometimes difficult, and laboratory assistance is usually required. Malignant edema—implying any clostridial myositis rather than specific C. septicum infections—also has been used as a general term for clostridial myositis.
C. chauvoei has the most confusing pathogenesis. The organism survives in soil, but it is not known whether it survives in both the vegetative and spore forms or only the spore form. Ingestion of C. chauvoei by cattle apparently allows the vegetative form to proliferate in the gut and then gain entrance to the lymphatics, bloodstream, and finally seed muscle and liver. Having reached the muscle and liver, the organism remains innocuously in the spore form unless the surrounding tissue is injured in some way that creates an anaerobic environment suitable for vegetative growth of C. chauvoei. Exogenous infections of muscle also are possible with C. chauvoei if soil contamination or inoculation of damaged tissue occurs. Farms and soils that harbor C. chauvoei create endemic risk of clostridial myositis for cattle grazing this ground or ingesting crops harvested from such soil. Young cattle appear to be at greatest risk for C. chauvoei muscular infections, and most cases occur in well cared for animals 6 to 24 months of age. However, we investigated a herd epidemic of C. chauvoei myositis that involved several first-lactation cows that ranged between 2 and 3 years of age. The cows in this outbreak had grazed pastures the previous summer, but the epidemic occurred during the winter months and was triggered by muscle bruising and trauma as a result of crowding through a narrow passage created by a frozen doorway (Figure 15-2). C. sordelli may have a similar pathogenesis because it has been associated with muscle bruising in rapidly growing beef cattle.
Regardless of the species of Clostridium causing infection, toxemia and severe myositis ensue. Clostridial exotoxins promote spread of the infection, are detrimental to host defense mechanisms, and propagate the anaerobic environment essential for vegetative growth. C. chauvoei, C. septicum, and C. perfringens produce alpha-toxin, which is a hemolytic and necrotizing lecithinase that is leukocidal and increases capillary permeability. In addition, C. chauvoei produces other toxins such as hyaluronidase; C. septicum produces beta-toxin (deoxyribonuclease and leukocidal), gamma-toxin (hyaluronidase), and delta-toxin (hemolysin); C. perfringens also may produce toxins other than the alpha-toxin, depending on the serotype involved. As a general statement, C. chauvoei and C. sordelli are linked with highest mortality, C. perfringens the least, and C. septicum in between. Occasional cases or specific geographic areas may encounter clostridial myositis as a result of C. novyi, C. sordelli, Clostridium fallax, or other species. C. novyi may cause exogenous infection or endogenous infections (“black disease”) as a result of fluke-induced hepatic activation of spores to vegetative forms.
Regardless of the causative species, most clostridial myositis in dairy cattle occurs by exogenous routes. Any procedure that allows feces or dirt to gain entrance to subcutaneous locations constitutes a risk. Minor surgical procedures, tail docking, IM injections, and neglected wounds predispose to clostridial infections. Tail docking is a relatively new management practice in the northeastern United States, and farmers heretofore unfamiliar with the procedure have been performing it. Clostridial myositis, tetanus, and ascending wound infections caused by other organisms have resulted from dirty tail docking techniques. An epidemic of C. perfringens myositis occurred following tail docking of an entire adult herd (Figure 15-3).
Clinical Signs
The signs of clostridial myositis include fever, depression, inappetence, toxemia, and a progressively enlarging region of swollen muscle. Lameness is severe if the myopathy involves limb musculature. Initially the skin over the affected muscle is warm, soft, and may have pitting edema. However, the primary muscle site eventually becomes firm, and the overlying skin is dark, taut, cool, and necrotic. Crepitus caused by gas formation may be palpable in the infected muscle. Soft tissue swelling progresses along fascial planes and ascends or descends, depending on anatomic location. Systemic signs are referable to toxemia induced by the potent clostridial exotoxins. Fever usually is present initially, but some patients may become so ill as a result of toxemia that normal or subnormal temperatures are recorded. Heart and respiratory rates are elevated progressively as the pathology worsens. Signs progress rapidly over a 24- to 48-hour course, and few cows survive after 3 days unless therapy is instituted. The clinical course may be so rapid as to be thought a sudden death. Usually, however, progressive swelling, toxemia, and lameness are observed before death.
Diagnosis
Blood work is not helpful to the diagnosis because neither a complete blood count (CBC) nor serum chemistry panel offers significant data. The leukogram result is extremely variable in clostridial myositis patients and most often is normal, despite the patient’s overwhelming infection and toxemia. Perhaps even more surprising is the fact that serum creatine kinase and aspartate aminotransferase values are sometimes only mildly elevated. In fact, muscle enzymes released from the region of profound myositis cannot gain access to the peripheral blood because of the self-serving vascular thrombosis and destruction created by clostridial exotoxins. Therefore absorption of enzymes and potassium from affected muscle is prevented by diminished blood supply to the lesion. Necropsy confirms the presence of black, deep red, or greenish red necrotic muscle with gas and fluid in C. chauvoei infections (Figure 15-4). Gas also may be present in other types of clostridial myositis, but edema and discolored muscles are the major lesions in C. septicum and C. perfringens. Serosal hemorrhages also can be observed in many tissues. Dr. John King, veterinary pathologist at Cornell University, likens the odor of affected tissue to the sickeningly sweet odor of rancid butter.
Treatment
Treatment seldom is successful unless the disease is diagnosed early in its course. Penicillin is the antibiotic of choice to kill vegetative Clostridium spp., and the drug should be used at very high levels (44,000 U/kg IM or subcutaneously [SQ], twice daily). Intravenous (IV) sodium or potassium penicillin given four to six times daily at the same dose is an excellent choice but may be too expensive for use in cattle. Some clinicians believe it is important to inject some of the penicillin into the region of the infection or proximal to the lesion in an affected limb. Sulfa drugs or tetracyclines also have been used successfully against clostridial myositis infections and were responsible for some of the earliest successful treatment in grade cattle. Systemic antibiotic therapy kills only those organisms that can be reached by viable circulation. Therefore it tends to counteract spread into new tissue but may not be able to attain inhibitory concentrations in the most severely affected muscle because of loss of blood supply in this tissue.
BACILLARY HEMOGLOBINURIA (REDWATER)
Clinical Signs
The disease usually occurs in adult cattle. Peracute illness with high fever (104.0 to 106.0° F/40.0 to 41.11° C), elevated heart rate, gastrointestinal stasis, cessation of milk production, loss of appetite, an arched stance, and evidence of abdominal pain ensue. Intravascular hemolysis causes progressive anemia, eventual dyspnea, and hemoglobinuria that appears after a significant loss of red blood cells has already occurred. Icterus also may be apparent. The course of the disease is rapid, with most patients dying in 12 to 48 hours.
Diagnosis
Gross necropsy findings are somewhat pathognomonic in that a large anemic liver infarct and “blackened” kidney are present (Figure 15-5, A and B and C). novyi can be cultured from the lesion. Despite the fact that C. hemolytica can be cultured from normal livers, the presence of the characteristic infarct associated with the organism usually is sufficient for diagnosis. Clostridial fluorescent antibody (FA) tests also can be helpful but may cross-react with C. novyi types B and C. Toxin identification is conclusive but may not be available.
LEPTOSPIROSIS
Etiology
Leptospira spp. are spirochetes that are considered saprophytic aquatic organisms, and those pathogenic for humans and animals do not appear to multiply outside the host. Infection occurs by penetration of the organism through the mucous membranes of conjunctiva, digestive tract, reproductive tract, skin wounds, or moisture-damaged skin. Hematogenous spread of the organism can result in seeding of multiple organs, including the uterus, and establishment of renal infection. Most Leptospira spp. colonize the renal tubules and are shed in urine for variable periods of time following infection.
Diagnosis
For acute infections in young calves showing hemoglobinuria, water intoxication is the major differential. Adult cattle showing acute septicemic disease and hemoglobinuria require differentiation from many diseases, including postparturient hemoglobinuria, bacillary hemoglobinuria, babesiosis, hemorrhagic cystitis associated with malignant catarrhal fever (MCF), enzootic hematuria, pyelonephritis, and other diseases causing “red urine.” Seroconversion assessed by comparative acute and convalescent titers is the best diagnostic proof of infection. Although several antibody tests are available, the microscopic agglutination test and enzyme-linked immunosorbent assay (ELISA) are used most commonly. FA techniques or dark field examination also can be used to detect leptospira in urine during acute infections with L. interrogans serovar pomona. A fourfold increase in convalescent titer over acute titer is considered significant and is even expected with most serovars. Vaccination of cattle generally causes a relatively low agglutination titer (400 or usually less).
TUBERCULOSIS
Etiology
Few diseases of cattle (other than perhaps brucellosis) generate the emotional, economic, and public health concerns that tuberculosis does. The consequences of a positive tuberculosis reactor cow or cows may entail depopulation of the herd and economic ruin—despite salvage and indemnity or compensation available through regulatory efforts. Few veterinarians in this generation have experience with the disease in dairy cattle and therefore have assumed the disease to be nearly eradicated and of little concern. However, eradication efforts directed toward tuberculosis have been hampered by confirmation of the disease in captive Cervidae, exotic imports and zoo animals, and cattle from Mexico. Additionally, since 1994 Michigan has recognized bovine tuberculosis caused by Mycobacterium bovis in wild white-tailed deer, with the discovery of tuberculosis in cattle populations since 1998. It is highly unusual to have self-sustaining bovine tuberculosis in a wild, free-ranging cervid population in North America, and it appears that high deer densities and the focal concentration caused by baiting (the practice of hunting deer over feed) and feeding may be responsible for this problem. A resurgence of surveillance efforts currently is underway to safeguard dairy cattle in the United States under the cooperative auspices of state and federal regulatory veterinary services. Surveillance programs have been diminished overall because of fiscal cutbacks at both the federal and state levels, but high-risk herds in areas where the disease has been confirmed or where cattle have had contact with infected Cervidae or Mexican cattle are still supported. Some states and some milkshed regions still mandate periodic tuberculin testing of all herds producing milk or supplying milk to the milkshed. Coupled with this concern of increased risk for certain cattle populations, the resurgence of tuberculosis in people has raised great concern.
Clinical Signs
Infected cattle that have clinically detectable lesions represent the minority of infected cattle. When present, clinical signs are extremely variable and often nonspecific. Loss of body condition and failure to thrive with progressive emaciation may occur in patients with more advanced disease. Classic respiratory signs of a chronic moist cough and thoracic abnormalities on auscultation may be the most suspicious signs but do not occur with great frequency. Lymph node enlargement coupled with chronic respiratory disease may result in a higher index of suspicion. Retropharyngeal lymph node involvement may cause either respiratory signs or difficulty in swallowing or eructation. Apparent forestomach or intestinal obstruction may accompany visceral lymph node enlargement. This is usually painless and may be associated with drainage in advanced cases. Udder infections occur in the minority of cases but, when present, have drastic public health ramifications if infected unpasteurized milk is consumed by humans or animals. Fortunately pasteurization destroys M. bovis in milk. Reproductive tract lesions also are rare. Both reproductive and mammary tissue infections usually are accompanied by associated lymph node enlargement.
ULCERATIVE LYMPHANGITIS
Etiology
Similar lesions have been identified in cattle associated with infection by Corynebacterium pseudotuberculosis. In these cases, the lesions are restricted to the lower limbs with or without lymph node enlargement. The ulcerative lesions may discharge a clear, gelatinous exudate. Infection of cattle with C. pseudotuberculosis can also cause granulomatous cutaneous abscesses, typically located on the exposed lateral face, neck, thorax and abdomen, or less commonly mastitis and visceral infections. Because the clinical signs are markedly different than ulcerative lymphangitis, these will be discussed below.
Clinical Signs
Multiple subcutaneous nodules in the metacarpal or metatarsal region are the primary lesions. One or more limbs may be affected. The nodules ulcerate periodically and discharge pus that varies from serous to caseated. Mild lameness may be apparent before ulceration and discharge as the nodules swell and become inflamed. Lameness resolves as drainage occurs. Over time, the nodules may coalesce or form knotted cords of tissue that mainly is subcutaneous but may have a dermal component as well (Figure 15-6). Other than periodic mild lameness and ulceration, systemic signs are absent.
CORYNEBACTERIUM PSEUDOTUBERCULOSIS INFECTION
In cattle, the cutaneous ulcerative lesions exude pus and are typically located on the exposed lateral face, neck, thorax, and abdomen. Affected cattle do not usually show other signs of disease, and the lesions may heal spontaneously in 2 to 4 weeks, although healing may be enhanced by drainage or surgical debridement. The infection often occurs as a herd problem, and up to 10% of cattle in a herd may be affected. The disease occurs more frequently in adult cattle than primiparous or nulliparous heifers. Spread of infection is apparently enhanced where housing and handling facilities cause abrasion to the lateral body surfaces. It has been assumed that skin trauma and contamination of minor skin abrasions by the organism are causative features of the disease. Affected animals are often culled. The disease has most commonly been seen in dairy cows in the arid western United States and Israel and occurs more frequently in the summer months.
BABESIOSIS
Diagnosis
Confirmation of babesiosis requires ancillary tests in addition to the suggestive clinical signs. B. bigemina is more likely to be observed on Giemsa-stained blood smears than B. bovis, but both organisms are more likely to be found in acute infections than in chronic cases. Antibodies against Babesia sp. may appear in the blood of infected cattle within 1 to 3 weeks and are sought by complement fixation (CF) or indirect FA tests. The FA test may be more sensitive and can detect antibodies for a longer period following infection than the CF. Brain biopsies also have been used in the diagnosis of B. bovis infections but are obviously of no value antemortem. Other serologic tests are being evaluated, but all suffer from a lack of availability.
Treatment and Control
Tick control is essential and certainly, based on the U.S. experience, necessary for eradication of babesiosis. Many effective acaricides currently are available (see Chapter 7). In some countries, tick control rather than complete eradication is practiced in the hopes of maintaining a low level of vectors to effectively immunize cattle but not enough to result in severe or widespread disease.
Tick control or eradication is the ideal control method when possible.
ANAPLASMOSIS
Etiology
Natural infection of young cattle results in a carrier state that may persist for the life of the animal. A biologic balance appears necessary to maintain immunity because clearing of infection eventually may allow susceptibility to reinfection. Seroconversion may occur despite the chronic carrier state, although it does not occur in all infected carrier cattle. Protective immunity requires both humoral and cellular immune components, including antibody against the outer cell membrane plus macrophage activation. The immune response can clear the acute rickettsemia but fail to completely clear the infection because of development of antigenic variants of the agent. Seropositive cattle are assumed to be carriers. Seronegative cattle in endemic areas are more difficult to categorize and the subject of much research. Further confusion is added by studies that demonstrate acquired immunity to clinical disease persisting following clearance of infection by chemotherapy. This immunity following chemotherapy with imidocarb or tetracycline persisted regardless of seropositive or seronegative status of the treated cattle. However, in endemic regions harboring anaplasmosis, seronegative cattle within A. marginale-infected herds appear susceptible to infection and illness. Therefore seronegative cows in positive herds have not necessarily developed effective immunity even if they had been seropositive previously and naturally cleared the infection later. Relative exposure rate, concurrent stresses, vector loads, and length of time between clearance of infection and subsequent reinfection all may influence the susceptibility of seronegative cattle that once had been seropositive.
Diagnosis
The CF and rapid card agglutination tests are the most common means of confirmation of infection but may not become positive until 1 week following acute infection. These same serologic tests are very useful to detect chronic carrier cattle that may be free of clinical signs. Diagnosis in acute cases is aided by ancillary tests that verify the severe anemia (low packed cell volume and regenerative) and also rule out liver disease as a cause of jaundice. Microscopic examination of whole blood smears stained by Wright’s, new methylene blue, or Giemsa stains may allow identification of A. marginale in erythrocytes (Figure 15-7). The organisms appear as one or more spherical bodies in the periphery of erythrocytes and must be differentiated from basophilic stippling and Howell-Jolly bodies. PCR and competitive ELISA are newer and more sensitive tests and should be used to help determine infection and clearance of infection following treatment.
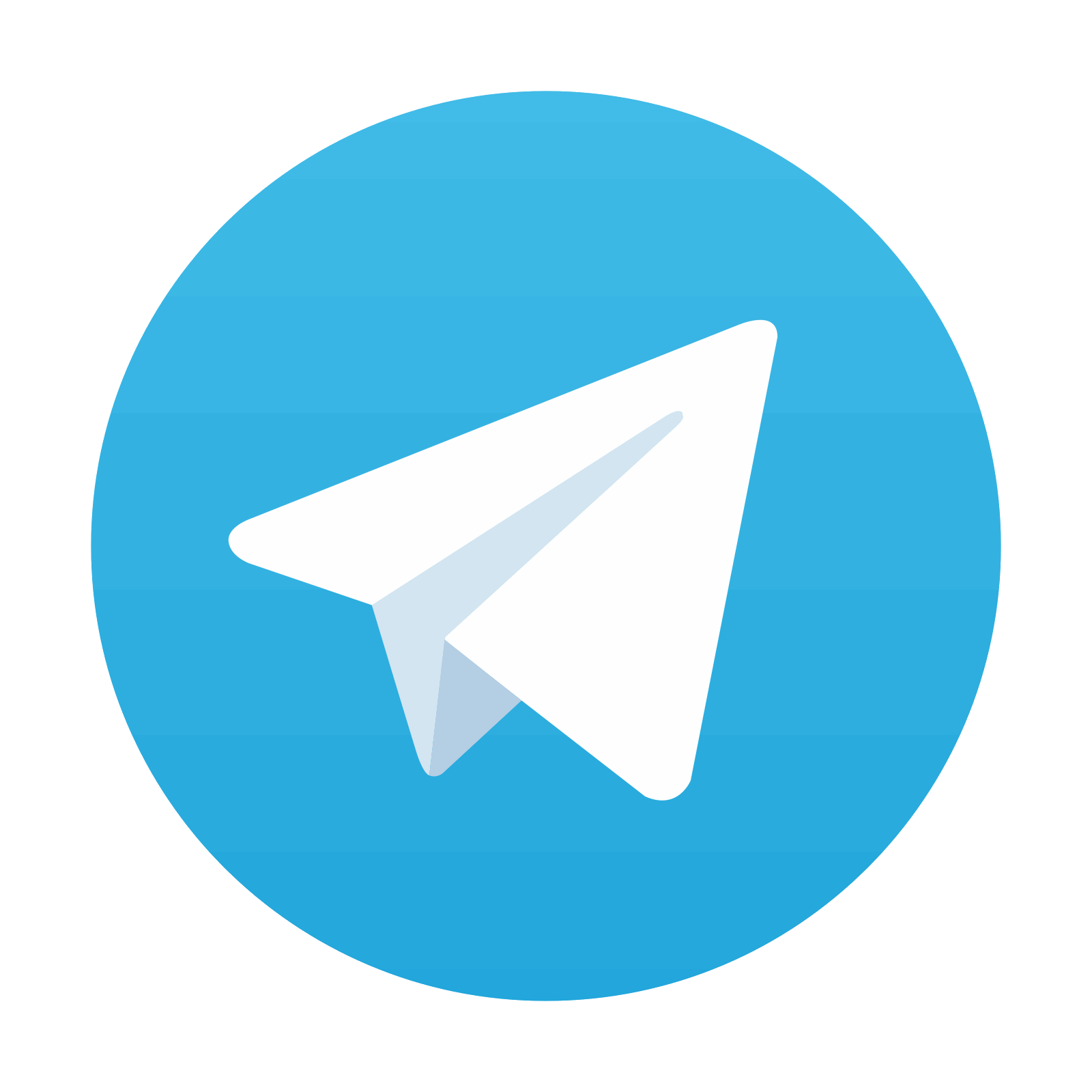
Stay updated, free articles. Join our Telegram channel

Full access? Get Clinical Tree
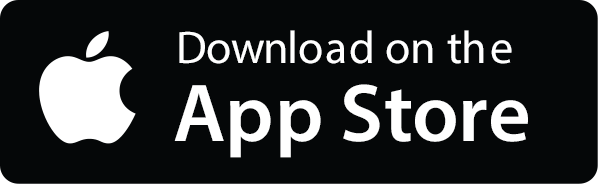
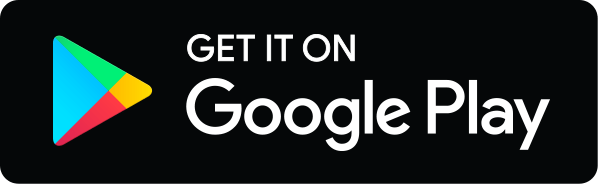