section epub:type=”chapter” role=”doc-chapter”> Philipp Schmierer and Antonio Pozzi Minimally invasive plate osteosynthesis (MIPO) is defined as reduction and fixation of a fracture with a bone plate without direct surgical exposure of the fracture site. Small skin incisions are used to prepare an epiperiosteal, subcutaneous, or submuscular tunnel that allows one to insert and apply the plate to the fracture fragments [1]. The first descriptions of MIPO in human patients dates back to the early 1990s [2], although the most significant progression has occurred in the last decade, largely thanks to the introduction of new implants such as the locking plate. In the last decade, MIPO was also introduced in veterinary medicine and is nowadays an accepted technique in dogs, cats, and horses [3–5]. Reported benefits of MIPO include reduction in operative time, reduced risk for bacterial infection, decreased soft tissue trauma, and preservation of the fracture hematoma [6]. Furthermore several studies in human, as well as in veterinary, medicine showed that MIPO preserves periosteal blood supply compared to open plating, which may accelerate bone healing [7, 8]. Reported disadvantages of MIPO include the technical difficulty and the inability of direct observation of the fracture fragments leading to an increased risk of malalignment [9, 10]. Clinical results after MIPO in dogs have been promising [11–13]. The initial reports of MIPO for tibial fractures in dogs and cats showed early radiographic union and return to full limb function. These positive results were confirmed in a larger study where the average healing time was 45 days and no major complications were reported [12]. The effect of MIPO on fracture healing was evaluated in a cohort of dogs with radius‐ulna fracture using ultrasonography, power Doppler ultrasonography and radiographs. The results of this study showed significantly shorter healing times for dogs that underwent MIPO compared to dogs treated with open reduction and internal fixation (ORIF). [11] However, the effect of MIPO on fracture healing time remains a controversial issue. Baroncelli et al. found no difference in time to clinical union evaluated by radiographs in a retrospective study comparing MIPO and ORIF in 22 dogs with tibial fractures [14]. Other studies concluded that MIPO allowed rapid healing, but the lack of a control make the interpretation of the results difficult [11, 12]. The evolution of MIPO in human orthopedics was accelerated by the development of locking plates. These angular stable implants serve as ideal implants to implement the principles of biological osteosynthesis for several reasons (Figure 5.1). Locking plates act as internal fixators, which eliminate the need for exact contouring. Additionally, the limited contact between bone and implant further helps to preserve the vascular supply to the bone [15]. This chapter aims to give an overview of MIPO principles and techniques, with special focus on the use of locking implants for fracture reduction and fixation. MIPO preserves an optimal environment for fracture healing by limiting surgical dissection and avoiding disruption of the fracture hematoma. It is clearly understood that careful soft‐tissue handling is very important in preserving blood supply to the injured bone. However, it is the combination of not exposing the fracture site and indirectly reducing the fracture that makes MIPO an excellent model for biological osteosynthesis. The fracture hematoma that forms following rupture of endosteal and extraosseal vessels plays an important role in initiating fracture healing [16]. For progressive fracture healing, however, adequate blood supply and oxygen tension at the fractured site is mandatory. The soft tissues surrounding the bone and the periosteum play a critical role in establishing the early blood supply after a fracture [17]. Thus, soft tissue and periosteum preservation using a minimally invasive technique may allow more rapid healing. The effect of MIPO and ORIF on periosteum preservation and early bone healing was studied in two separate experiments. In a cadaveric study of the canine antebrachium, MIPO caused less disruption of periosteal blood supply of the radius when compared to ORIF [8]. In a separate clinical study, earlier vascularization of the fracture callus compared to open plating was demonstrated using ultrasound, confirming the benefit of MIPO in the initial phase of bone healing [11]. Locking plates are ideal implants for preserving periosteal vessels. The periosteal blood supply beneath locking plates is not compromised because compression between the plate and the bone does not occur (Figure 5.1b); this may improve healing and decrease the risk of cortical bone necrosis and infection [18]. A large multicenter clinical study in people reported an infection rate of 1.1% after using the point‐contact fixator (PC‐Fix), one of the earliest locking plate designs [19]. Other studies have shown decreased damage to the periosteum and underlying bone and improvements in bone healing with maintenance of a sound bone structure [20]. It is clear that locking plates should be considered as an excellent implant choice for MIPO. Figure 5.1 Cranio‐caudal radiograph of a three year‐old Domestic Short‐Haired Cat presenting with a comminuted distal diaphyseal tibial fracture. The fracture was reduced manually and a precontoured veterinary cuttable plate (DePuySynthes VET, Oberdorf, BL, Switzerland) was applied to the medial aspect (a). Cranio‐caudal radiograph of a five‐year‐old Domestic Short‐Haired Cat presenting with a mildly comminuted distal diaphyseal tibial fracture. Multiple fissures extend from the fracture site into the proximal fragment. The fracture was reduced manually and Advanced Locking Plate System (KYON, Zurich, ZH, Switzerland) was applied (b). Note that exact contouring is necessary with the nonlocking implant (a) while only approximate contouring is adequate in the locking implant because plate standoff is tolerated (b). The process of bone healing is dependent on numerous interactions between biologic and mechanical factors. If the local circulation is adequate to support fracture healing, the pattern of bone healing is then dependent on the surrounding biomechanical environment. Most fractures repaired with MIPO techniques heal in conditions of relative stability. Relative stability involves placement of implants that provide somewhat flexible fixation, allowing an acceptable degree of fracture segments displacement. Fixation modalities that are commonly employed in MIPO are plates or plate‐rod constructs applied in bridging fashion to span a bone defect, resulting in a relatively stable environment. Understanding the difference between locking and nonlocking plates and the effect of plate type, size, length, position, screw type, and screw placement is important because successful fracture healing depends on appropriate fixation stability. The principal biomechanical differences between conventional and locking plates is the mode of load transfer through a fractured bone. In conventional compression plate constructs or nonlocking bridging plate constructs, fixation stability is limited by the frictional force generated between the plate and the bone. These frictional forces are the result of axial screw force and the friction coefficient between the bone and the plate [21]. If the force exerted on the bone while the dog is ambulating exceeds the frictional limit, relative shear displacement will occur between the plate and the bone, causing a loss of reduction between the bone fragments, or loosening of the screws, or both. Locking plates differ from nonlocking plates because stability is not dependent on the frictional forces generated at the bone–plate interface. These implants consist of a plate and locking head screws, which together act as an internal fixator. Locking the head screw into the plate hole confers axial and angular stability of the screw relative to the plate. Because the stability of the construct does not depend on frictional forces generated between plate and bone, the bone–screw threads are unlikely to strip during insertion. With the screwhead locked in the plate, screw orientation is fixed to the plate resulting in a single beam construct functioning as an internal fixator. With the fixed‐angle and the screw locked in the plate, no movement at the plate screw interface is allowed, resulting in a decreased risk of screw pullout and screw loosening [22–25]. When performing bridging osteosynthesis, the selection of an implant of appropriate length is a crucial step. With longer plates, screw‐working leverage is improved and bending forces are well distributed along the plate, thereby lowering pullout forces on screws [26]. To determine the adequate length of the plate in the preoperative plan for bridging osteosynthesis in MIPO, two values have been used to determine the plate length to be used. The plate span ratio is the quotient of plate length and segmental length of fractured/comminuted bone. The plate screw density is the quotient of number of screws inserted and number of screw holes. Plate span ratio in comminuted fractures, in which MIPO with bridging osteosynthesis is most commonly performed, should be more than two to three. Values for simple fractures range between eight to ten [26]. Plate screw density should be smaller than 0.5 to 0.4 in comminuted fractures. A value of 0.4 to 0.3 is recommended for simple fractures [26]. Besides plate span ratio and plate screw density, the location of the screws along the plate and in relation to the fracture should be considered. The plate working length is defined as the distance between the distal and the proximal screw nearest to the fracture. Its influence on plate strain, construct stiffness, and cyclic fatigue of the plate construct has been evaluated in human and veterinary studies. Recommendations aim toward increased plate working length in order to reduce axial stiffness of the construct and to allow interfragmentary movements [26, 27]; however, conflicting results have been found in several mechanical studies [28–30]. The plate working length in comminuted fractures might not be equal to the distance between the screws closest to the fracture, but rather to the unsupported area of the plate, which correspond to the length of the fracture gap. Also of interest is the location and number of monocortical and bicortical screws in the construct as they are influential on its biomechanical properties. Less torsional stiffness is reached with monocortical screws compared to bicortical screws. Torsional stiffness of the fixation construct is especially relevant in bones undergoing combined axial and torsional loading such as the tibia [31, 32]. A minimum of one screw placed bicortically in each major bone fragment has been shown to significantly increase torsional stability in a biomechanical study using bone models. Interestingly, when the bicortical screw was placed at the innermost position, closest to the fracture, greatest improvement in torsional stability was observed [34]. Before performing MIPO the surgeon should be familiar with the regional and topographic anatomy to avoid harm to neurovascular structures and to minimize postoperative morbidity [34, 35]. The position of the animal on the table is important for access, but also for the use of fluoroscopy without interference with the table or the other limbs. The skin incisions are centered over the expected proximal and distal ends of the plate and an epiperiosteal soft tissue tunnel connecting the two incisions is created with blunt dissection. Long blunt scissors or periosteal elevators are best suited for tunnel creation (Figure 5.2). Figure 5.2 Minimally invasive plate osteosynthesis in a tibial fracture of a cat. Metzenbaum scissors are used for creation of the epiperiosteal soft tissue. Fracture reduction for MIPO is performed using indirect techniques, consisting of repositioning the bone fragments using specific distraction and translation techniques without direct exposure of the fracture site [36–38]. Fracture fragments are manipulated applying forces distant to the fracture [37, 38]. Indirect fracture reduction adapts perfectly to the concept of biological osteosynthesis because the fracture hematoma and the soft tissue envelope around the fracture is preserved [38, 39]
5
Minimally Invasive Plate Osteosynthesis
5.1 Introduction
5.2 Biology and Biomechanics in MIPO
5.3 Surgical Technique
5.3.1 Approach and Dissection
5.3.2 Fracture Reduction
Stay updated, free articles. Join our Telegram channel

Full access? Get Clinical Tree
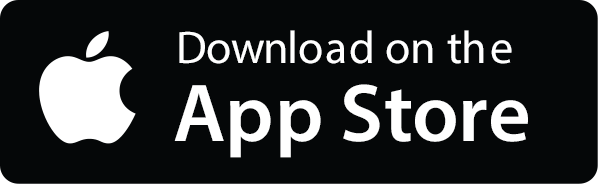
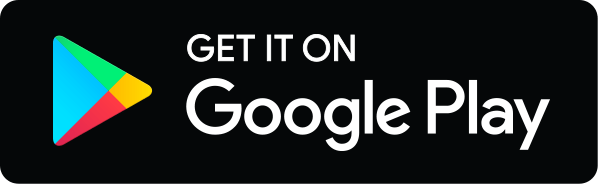