Chapter 25 Caleb O. Lemley1, Leticia E. Camacho2 and Kimberly A. Vonnahme2 1 Department of Animal and Dairy Sciences, Mississippi State University, Starkville, Mississippi, USA 2 Department of Animal Sciences, North Dakota State University, Fargo, North Dakota, USA Maternal recognition of pregnancy encompasses the process whereby a biochemical signal is generated to prevent luteal regression, allowing the corpus luteum to persist and continue to secret adequate progesterone. The requirement of progesterone for the successful maintenance of pregnancy exceeds the length of the bovine estrous cycle and therefore the pregnant female must quickly recognize that a conceptus is present. In cattle, this signal involves conceptus secretion of interferon (IFN)-tau which further stimulates luteotropic and antiluteolytic signals. Although some studies use these words interchangeably, we will further define a luteotropin as a signal that stimulates luteal secretion of progesterone, while antiluteolytic substances act as a signal to block luteolysis by inhibiting or masking endogenous luteolytic signals. This maternal recognition of pregnancy signal is also occurring well in advance of conceptus implantation, which begins during the third week of pregnancy, and is classified as a superficial attachment in cattle. Several biological assays have focused on elucidating substances involved in prolonging the lifespan of the bovine corpus luteum. The majority of these earlier assays focused on examining estrous cycle length in nonpregnant cattle injected with pituitary extracts. In 1965, researchers at Cornell University reported that luteinizing hormone (LH) was the luteotropin for cattle and indeed injections of pituitary extracts or purified bovine LH extended the bovine estrous cycle by 11 or 16 days, respectively.1 This study confirmed the luteotropic actions of LH, which could serve as the endogenous biochemical signal from the conceptus; however, apart from primates and mares, no other mammals appear to possess both genetic subunits for a chorionic gonadotropin. Therefore, a chorionic gonadotropin possessing LH properties is not the signal for maternal recognition of pregnancy in cattle. However, these early studies did contribute to the foundational groundwork examining mammalian species differences in prolonging the lifespan of the corpus luteum, which is an important component of the conceptus–maternal cross-talk during maternal recognition of pregnancy. These early results led researchers to examine other potential substances originating from the conceptus that may act in a luteotropic and/or antiluteolytic nature to prevent luteolysis during pulsatile release of prostaglandin (PG)F2α. Intrauterine infusions of estradiol-17β and PGE2 prolong the lifespan of the bovine corpus luteum as well as progesterone secretion by 5–6 days compared with nonpregnant controls.2 The luteotropic actions of estradiol-17β are more than likely indirect because estrogens have been show to inhibit LH-induced secretion of progesterone by cultured bovine luteal cells.3 The indirect actions of intrauterine estradiol-17β on prolonging luteal lifespan may involve an increase in uterine and/or ovarian blood flow. A local increase in blood flow to the gravid uterine horn occurs coincidental with maternal recognition of pregnancy in the sow, ewe, and cow.4 In addition, this local increase in uterine blood flow may help to redirect prostaglandin transport from the uterine horn to the adjacent ovary via the utero-ovarian plexus. PGE2 directly stimulates progesterone secretion and cyclic AMP production of bovine luteal cells in vitro and in a similar manner to that of LH.5 Moreover, both the bovine endometrium and corpus luteum express the complete prostaglandin biosynthesis pathway and the differential expression of these components during the critical period of maternal recognition of pregnancy (days 15–17 of the estrous cycle) can exert luteotropic or antiluteolytic actions.6 Interferon-tau, first identified as bovine trophoblast protein 1,7 is recognized as the maternal recognition of pregnancy signal in cattle.8 Interferon-tau secretion by embryonic trophoblast cells is highest between days 15 and 17, and is observed up to day 28 of pregnancy; moreover, an inadequate response of the endometrium to IFN-tau is likely to be one of the major reasons for improper production and action of PGF2α and PGE2 leading to pregnancy failure. In vitro cultures of bovine endometrial cells with increasing concentrations of IFN-tau show preferential production of PGE2 compared with PGF2α.9 Moreover, the spatial and temporal relationship of prostaglandin metabolism, transport, and receptor density changes substantially in the uterine endometrium and myometrium following IFN-tau exposure.6 Elevated physiological concentrations of IFN-tau from the conceptus will selectively increase the ratio of PGE2 to PGF2α. This relationship sets up an antiluteolytic effect of IFN-tau combined with a luteotropic effect of PGE2 which eventually leads to prolonged corpus luteum lifespan and proper establishment of pregnancy. In addition, embryonic secretion of PGE2 may produce a synergistic effect with the increased endometrial production of PGE2 that was stimulated by conceptus secretion of IFN-tau. This PGE2 can be competitively transported through the utero-ovarian plexus to the ovary, where it increases production of luteal PGE2, thereby favoring luteal maintenance as a luteotropin.6 In contrast, an elevated ratio of PGF2α to PGE2 will stimulate uterine contractility and competitive transport of PGF2α through the utero-ovarian plexus toward the corpus luteum, thereby initiating luteal regression. Together, uterine contractility and luteal regression can lead to pregnancy failure and/or a return to a new estrous cycle following a normal lifespan of the corpus luteum. Prevention of corpus luteum regression and continual secretion of adequate progesterone is vital for successful maintenance of pregnancy to term. The primary targets for progesterone are the reproductive tract. The amplitude of progesterone secretion can influence the early uterine environment, including the inhibition of mitotic division of the endometrium, the induction of stromal differentiation, and the stimulation of additional glandular secretions of the uterine epithelium or histotroph.10 This increase in glandular uterine secretions of nutrients and growth factors is essential for early conceptus development.11 In 1956, researchers proposed the progesterone block hypothesis, which states that progesterone maintains pregnancy by directly blocking labor and removal of the progesterone source will initiate parturition.12 Later studies revealed that progesterone supplementation prevented the insertion of gap junction proteins between uterine smooth muscle cells by decreasing the expression of connexin-43.13 Progesterone has also been shown to downregulate uterine contractility-associated genes.14 In general cows with less than 0.5 ng/mL of progesterone have elevated contractility in circular smooth muscle cells of the myometrium compared with that in diestrus cows (>4.0 ng/mL progesterone).15 These differences in myometrial excitation–contraction are brought about by the genomic actions of progesterone, which lead to a relaxed and quiescent uterus able to maintain a successful pregnancy until progesterone withdrawal.14 In addition, progesterone synthesis depends on luteal production for the first 200 days of gestation; however, the extragonadal source of progesterone, primarily the bovine placenta, does not secret adequate amounts of progesterone until the third trimester of pregnancy. This placental progesterone secretion is dependent on luteal ovarian input. The luteal placental shift in progesterone secretion, which may contribute to the maintenance of pregnancy, only occurs after PGF2α-induced luteolysis or in ovariectomized animals.16 Moreover, in the cow, the corpus luteum may exert control over placental steroidogenesis, which does not take over progesterone secretion until ovarian luteal deficiencies become apparent during late gestation. The early elongation of the bovine conceptus involves exponential increases in length and width of the trophectoderm and requires differentiation of extraembryonic membranes as well as maternal endometrial influences. This elongation begins on day 14, following the hatching of the ovoid blastocyst at approximately day 9–10, and forms a filamentous conceptus by day 19–20 after insemination.17 On day 14, the majority of ovoid or tubular embryos of approximately 5 mm in length will start to form an epiblast, defining the embryonic disk, which is discernible from the epithelium of the trophoblast. Both of these compartments are internally lined by the continuous hypoblast of which the majority stains positive for α-fetoprotein;18 however, the histological appearance of the hypoblast varies in relation to its spatial orientation to the epiblast versus the trophoblast cell layers. The spatial orientation results in two types of hypoblast cells: squamous cell types if located next to the trophoblast layer, or cuboidal to columnar cell types underneath the epiblast or embryonic disk.18 At this point a distinctive basal lamina is formed between the trophoblast or epiblast and the hypoblast cell layers. In addition, adjacent epiblast cells are connected via tight junctions, as well as peripheral epiblast cells next to the trophoblast cells. Presumptive signs of early embryonic mortality in the tubular conceptus consist of apoptotic epiblast cells or a loosening of the hypoblast from the trophoblast. During this elongation phase the initial folds of the amnion will form as an outgrowth of the trophoblast cells adjacent to the epiblast, while the hypoblast is not included in the formation of this extraembryonic membrane. The most discernible difference during this time-frame is the hypertrophic and hyperplastic trophectoderm growth of the differentiating chorionic sac, which may grow from a few centimeters up to 30 cm or more.19 The early attachment period of days 20–33 post insemination proceeds with sequential stages of pre-contact, apposition, and adhesion between the uterus and the chorion, and ends in a microvillous interdigitation of the uterine epithelium and trophoblast. This time period coincides with several reports of limited success in flushing intact conceptuses for experimental use.20 During the early attachment phase the development of the allantois begins, and on day 21 after insemination a strong positive association between embryo length and width of the allantois can be observed.18 Some researchers have observed abnormalities or underdevelopment of the extraembryonic membranes following in vitro fertilization or somatic cell nuclear transfer; however, the underlying mechanisms for development failure are not clearly understood. Therefore, embryonic mortality during these time points may be caused by a difficulty in nutrient mobilization brought about by the allantois showing deficient vascularization, growth retardation, or its absence.21 In relation to the dramatic elongation of the trophectoderm of the chorionic sac, the allantois undergoes gradual growth from days 21 to 25 post insemination, when it will start to occupy both uterine horns and begin fixation to the chorion to form the chorioallantois placenta; however, this fusion remains incomplete until days 60–70 of pregnancy.21 In addition to the morphological change from a spherical conceptus into a filament-like tube during conceptus elongation, several molecular markers have been characterized in relation to growth and cellular differentiation. The trophoblast mononucleate cells of the trophectoderm will begin to proliferate and differentiate, which may be induced by specific transcription factors such as POU-domain class 5 transcription factor, ERG, and CDX2.22 These transcription factors have been shown to regulate genes such as IFN-tau and trophoblast domain proteins, which are upregulated during maternal recognition of pregnancy. In addition, the differentiation of trophoblast mononucleate cells into trophoblast giant cells coincides with an increase in pregnancy-associated glycoproteins, prolactin-related proteins, and placental lactogen. These proteins are associated with cellular differentiation of the trophectoderm; however, they may not be associated with the proliferation of the trophectoderm during conceptus elongation into the filament-like tube.22 Transcriptome profiling using a bovine-specific microarray has revealed the upregulation of approximately 500 genes and the downregulation of only 26 genes during transition from a blastocyst (day 7) to an ovoid tubular conceptus (day 14). Of these genes, IFN-tau was positively correlated with conceptus size; therefore, it may be a key factor in trophectoderm proliferation during the elongation phase. After further elongation of the bovine conceptus (gestational days 17–19), specific genes involved in trophoblast cell differentiation, migration, and adhesion are upregulated, all of which play a role in fusion of the trophoblast cells to the endometrium of the uterus. Of the 80 genes upregulated during this phase, the CD9 gene, which encodes for cell-surface glycoprotein belonging to the tetraspanin family, of the differentiated trophoblast giant cells more than likely plays a role in cellular migration and adhesion of the conceptus to the maternal endometrium during early attachment (gestational day 20). The growth, development, and welfare of the bovine conceptus depend on uterine histotrophic nutrition derived from the secretions of uterine endometrial glands. The uterine endometrium is a complex tissue composed of a luminal epithelial cell layer, superficial glandular epithelium, deep glandular epithelium, as well as stromal cells with a fibroblast-like morphology. In addition to autonomous regulation of trophectoderm proliferation and differentiation during conceptus elongation, uterine input via endometrial secretions will also drive early conceptus development. As previously mentioned, the bovine conceptus must secrete sufficient quantities of IFN-tau by day 16 of pregnancy in order to inhibit the production of luteolytic pulses of PGF2α by the endometrium to allow for maternal recognition of pregnancy. In addition to conceptus secretions that alter endometrial pathways in a paracrine manner, ovarian hormones have also been shown to stimulate or inhibit endometrial secretions and glandular output. A number of studies have demonstrated that peripheral concentrations of progesterone during the early post-conception period are associated with advanced conceptus elongation and higher pregnancy rates in cattle and sheep. In contrast, lower concentrations of progesterone in dairy cows are associated with decreased conceptus development. Ovarian progesterone can influence the early uterine environment including endometrial sections of nutrients and growth factors that are essential for early conceptus development. Moreover, supplementing exogenous progesterone from day 5 to 9 after insemination resulted in a fourfold increase in trophoblast length and a sixfold increase in uterine concentrations of IFN-tau.23 In addition to the concentrations of progesterone in peripheral circulation, progesterone receptor expression in the endometrium (both luminal epithelium and superficial glandular epithelium) is altered in cows with decreased versus elevated concentrations of progesterone during early post-conception. In cows with low post-conception concentrations of progesterone not only are specific nutrient transporters decreased in the endometrium but also progesterone receptor expression is maintained for a longer period of time in the luminal and superficial glandular epithelium. In contrast, elevated concentrations of progesterone, brought about by exogenous treatment, increase nutrient transporter expression and decrease progesterone receptor expression in the luminal and superficial glandular epithelium.24 Moreover, this downregulation in endometrial progesterone receptors is required to establish uterine and endometrial receptivity to conceptus attachment. The placenta plays a major role in the regulation of fetal growth. The ruminant placenta is morphologically classified as cotyledonary and histologically as syndesmochorial. On the uterine wall there are specialized areas of endometrium called caruncles, with a button-like appearance.25 In nonpregnant ruminants, the caruncles are organized in two dorsal and two ventral rows that run lengthwise along the uterine horns. There are approximately 100 caruncles and they can be seen even in uteri from fetal calves.26 The chorioallantois, which has a flat surface, becomes irregular when it starts to cover the caruncles; this is caused by the growth and expansion of the conceptus on the lumen of the uterus. This process is followed by the recognition of the cotyledons.26 The caruncular–cotyledonary unit is called a placentome and is formed from the growth and interdigitation of fetal villi and caruncular crypts adopting a convex shape.25 Contact surface area is enhanced because the cotyledon finger-like projections enter the crypts formed in the caruncles. Placentomes vary in size, but they are bigger in the horn and decrease in size close to the tip of the horns.26 The placentome is the primary functional area of physiological exchanges between mother and fetus. The efficiency of placental nutrient transport is directly related to uteroplacental blood flow.27 All nutrients and wastes that are exchanged between the maternal and fetal systems are transported via the uteroplacental unit.27,28 Establishment of functional fetal and uteroplacental circulations is one of the earliest events during embryonic/placental development.29,30 In order to support the exponential increase in fetal growth during the last half of gestation,31 proper growth and development of the uteroplacental vascular bed must occur during the first half of pregnancy.27,32 Understanding the factors that impact uteroplacental blood flow will directly impact placental efficiency and thus fetal growth. However, despite intensive research in the area of placental–fetal interactions, the regulators of placental growth and vascularization as well as uteroplacental blood flow are still largely unknown, particularly in cattle. Limited data on development of the bovine placentomal capillary bed are available. In the cow, cotyledonary growth progressively increases throughout gestation.33,34 Histological analysis of capillary bed development in the cow has been performed at mid and late gestation.34 From mid to late gestation, capillary area density, a measure related to blood flow, decreases by about 30% in caruncular tissue, while it increases about 186% in cotyledonary tissue (Figure 25.1). The numbers of capillaries increase by about 150% and 80% in caruncular and cotyledonary tissue, respectively, during this time. Capillary surface density, a measurement related to nutrient exchange, is also increased in both caruncular and cotyledonary tissues (32% and 172%, respectively), while capillary size decreases 67% in caruncular tissue but increases 71% in cotyledonary tissue from mid to late gestation (Figure 25.1). The pattern of capillary development is very different between the maternal and fetal portions of the placentome possibly due to the energetic demands of these independent tissues that share a similar function – delivery of nutrients to the developing calf. Figure 25.1 Capillary number density, area density, surface density, and size in the maternal (caruncular) and fetal (cotyledonary) portion of the bovine placentome from mid (day 125) to late (day 250) of gestation. It is clear that the placenta plays a fundamental role in providing for the metabolic demands of the fetus. Although placental growth slows during the last half of gestation, placental function increases dramatically to support the exponential rate of fetal growth.35,36 For example, in sheep and cattle, uterine blood flow increases approximately threefold to fourfold from mid to late gestation27,37,38 (see below). The relationship between uteroplacental blood flow and conceptus size throughout gestation is further defined in the next section. During pregnancy the physiologic state of the dam is associated with significant but reversible alterations to metabolic demand as well as alterations to the endocrine and cardiovascular systems. As previously mentioned, during early gestation (e.g., maternal recognition of pregnancy and conceptus elongation) endocrine secretions of the ovary and conceptus help establish a healthy pregnancy. Moreover, these secretions serve as biochemical cross-talk between the maternal endometrium and fetal membranes of the placenta during mid to late gestation and help assure proper nutrient and waste exchange during exponential growth of the fetus. Maternal cardiovascular functional capacity changes dramatically during pregnancy, whereby systemic arterial blood pressure and vascular resistance decrease and cardiac output, heart rate, stroke volume, and blood volume increase.39 Although not all these variables have been determined during bovine pregnancy, several mammalian species including the ewe have shown a decrease in mean arterial pressure in early pregnancy that persists throughout gestation. Moreover, the decrease in arterial pressure (estimated as 5–10%) is minor compared with the approximate 20–30% decrease in total peripheral vascular resistance. Maternal cardiac output has been shown to increase as much as 30–40% in pregnant versus nonpregnant ruminants. Therefore, the increase in cardiac output is associated with a dramatic fall in systemic vascular resistance, allowing researchers to characterize pregnancy as a state of systemic vasodilation resulting in profound increases in total systemic flows to all vascular beds. The majority of these studies characterize this relationship by the equation:
Maternal Recognition and Physiology of Pregnancy
Maternal recognition of pregnancy
Conceptus elongation and extraembryonic membranes
Uterine histotroph
Placental vascularization
Maternal cardiovascular function
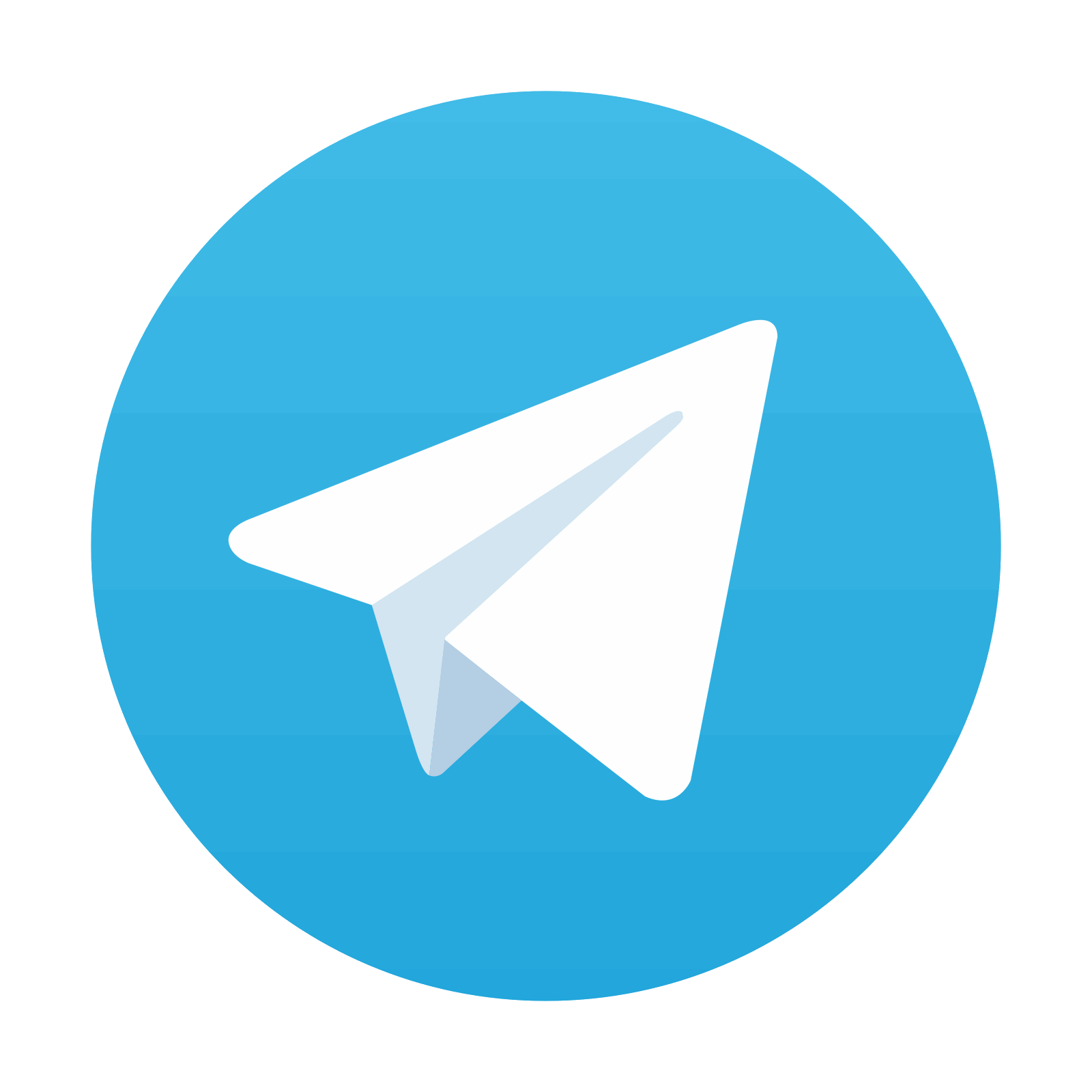
Stay updated, free articles. Join our Telegram channel

Full access? Get Clinical Tree
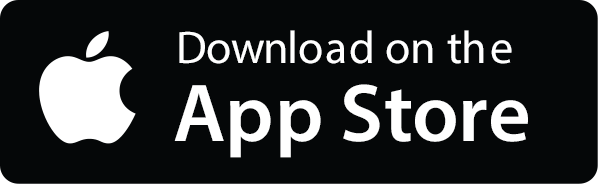
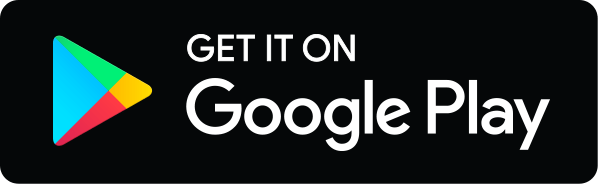