CHAPTER 7 Lower Motor Neuron
General Visceral Efferent System
GENERAL ANATOMY
The GVE system is grouped physiologically and anatomically into two components: the sympathetic and parasympathetic systems. This LMN is a two-neuron system between the central nervous system (CNS) and the effector organ innervated. These two neurons are referred to as the preganglionic and postganglionic neurons. The cell body of the preganglionic neuron is in the gray matter of the CNS, and the cell body of the postganglionic neuron is in a ganglion in the peripheral nervous system. The telodendron of the preganglionic neuron synapses on the dendritic zone of the postganglionic neuron in this ganglion. To be accurate, only the axon of the second neuron is actually postganglionic. The sympathetic system is referred to as the thoracolumbar system based on the location of the cell body of the first neuron, the preganglionic neuron, that is in the lateral gray column from spinal cord segments T1 to about L4 or L5. As a general rule, the sympathetic ganglia are located fairly close to the CNS, and the postganglionic axons are fairly long. With a few exceptions, the neurotransmitter released at the telodendron of the sympathetic postganglionic neuron is norepinephrine. Thus the sympathetic system is referred to as the adrenergic system. The parasympathetic system is referred to as the craniosacral system because the cell bodies of the preganglionic neurons are located either in the brainstem nuclei of cranial nerves III, VII, IX, X, and XI or in the sacral spinal cord segments. As a general rule, the ganglia of this parasympathetic system are located in or fairly close to the effector organ, and the postganglionic axons are short. Acetylcholine is the neurotransmitter released at the telodendron of the postganglionic axon. Thus, this system is known as the cholinergic system. The anatomic components of this GVE-LMN should have been learned in your dissection course on the anatomy of the dog. (Refer to Guide to the Dissection of the Dog by H. E. Evans and A. de Lahunta, ed 6, Philadelphia, 2004, Elsevier). For clinical purposes, the GVE-LMN can be divided into four components, and the veterinary student must understand the functional anatomy of all four: control of the pupils, of micturition and defecation, of the enteric system, and of the cardiorespiratory system.
CONTROL OF THE PUPILS
Parasympathetic Innervation of the Eye
Anatomy
The sensory anatomic pathway stimulated by the light is described in detail in Chapter 14, the visual system. In brief (Fig. 7-1), the light must initially stimulate receptors in the retina. The area centralis of the retina is the most sensitive to light and in carnivores is just lateral to the optic disk. The impulses generated in the retina travel through the optic nerve of that eye and continue in both optic tracts to the most dorsal caudal and lateral aspects of the thalamus, where they pass over the lateral geniculate nuclei and enter the pretectal nuclei in the most rostral part of the midbrain (see Fig. 2-5). Synapse occurs there, and the majority of the axons of the pretectal neurons cross through the caudal commissure to synapse on the GVE parasympathetic LMNs in the most rostral part of the contralateral oculomotor nucleus in the midbrain (see Fig. 2-6). A smaller number of pretectal neurons project directly to the rostral part of the ipsilateral oculomotor nucleus. Thus, light directed into one eye stimulates a series of neurons that terminate in both parasympathetic nuclei of the oculomotor nerve, resulting in a response in both eyes.
For this GVE-LMN pathway, the cell bodies of the preganglionic neurons are located in the parasympathetic nucleus of the oculomotor nerve in the rostral part of the mesencephalon. In humans, this nucleus is known as the nucleus of Edinger-Westphal. It is located in the ventral part of the central gray substance next to the median plane, where it is continuous caudally with the general somatic efferent (GSE) motor nucleus of the oculomotor nerve that is described in Chapter 6. The GVE axons course ventrally and emerge with the GSE axons in the lateral aspect of the interpeduncular fossa on the medial side of the crus cerebri. The GVE axons in the canine oculomotor nerve are located superficially on the medial side of this cranial nerve, where they are at risk for being compressed by extraparenchymal neoplasms and by disorders that cause the mesencephalon to swell or be displaced. The oculomotor nerve, with the GVE preganglionic axons, passes through the orbital fissure into the orbit within the periorbita, where it is adjacent to the lateral side of the optic nerve for a few millimeters. The oculomotor nerve then abruptly branches to the extraocular muscles. Located at this point of branching is a small ganglion, the ciliary ganglion. In this ganglion, the telodendrons of the GVE preganglionic neurons synapse on the dendritic zones of the cell bodies of the postganglionic neurons. The postganglionic axons pass by way of short ciliary nerves along the surface of the optic nerve to the eyeball to innervate the smooth muscle of the ciliary muscle and the sphincter of the pupil.13 The more light that enters the eyes and stimulates the retina, the more impulses will reach this parasympathetic pathway and cause the sphincter muscles to contract and the pupils to constrict. In diminished light there is less stimulus to these sphincter muscles, so the pupils passively dilate. Turn off the lights in the room and watch this happen.
When you direct the light into one eye, you evaluate the direct response in that eye and the indirect or consensual response in the opposite eye. You appreciate this consensual response when you swing the light to the opposite eye. This terminology can be very confusing and it is much better to record what happens in each eye when you direct the light into one eye and repeat it for the other eye. Sometimes, the degree of constriction seen in the eye in which the light is directed (the direct response) is slightly greater than the degree of constriction seen in the opposite eye (indirect, consensual response). This is normal. It occurs because the majority of the axons cross in the optic nerve at the optic chiasm. A similar crossing back of the majority of the axons of the neurons occurs in the pretectal nucleus in the midbrain via the caudal commissure. As a result, the parasympathetic nucleus of the oculomotor nerve on the side ipsilateral to the eye being stimulated receives the greater stimulus. This difference is more obvious in horses and farm animals than in small animals; this correlates with the larger number of axons that cross in the optic chiasm in the former. Be aware that the rate of pupillary constriction varies among species and among individuals of the same species. The rate of constriction is slowest in horses and cattle.
Clinical Signs
Lesions restricted to the visual pathways in the cerebrum can cause blindness but the pupillary light responses are normal. Note that in Fig. 7-1, no cerebral structures are present. Compare this with Fig. 14-5, which depicts the entire pathway of vision. For the examples presented in Table 7-1, imagine the site of the lesion in Fig. 7-1 and consider the clinical signs that would result. For these examples, consider that each lesion causes complete dysfunction of the structure indicated. These clinical signs are summarized in Table 7-1 and the description follows. Remember that the normal menace response requires the entire visual system pathway from the eye to the cerebral cortex to be intact as well as a pathway to the facial nuclei in the medulla. The term oculus sinister (OS) refers to the left eye. The term oculus dextra (OD) refers to the right eye. Oculi uterque (OU) refers to both eyes.
Be aware that it takes a severe retinal or optic nerve lesion to interfere with the pupillary response to light. Clinical blindness and absent menace responses may occur in the presence of retinal and optic nerve lesions before the pupil response to light is lost. Usually animals with bilateral retinal or optic nerve lesions have pupils that are more dilated than normal in room light because of the loss of some of the pupillary light neurons bilaterally. This is commonly seen in acute optic neuritis or sudden acquired retinal degeneration syndrome.2
Remember that a complete oculomotor nerve lesion causes not only a dilated pupil unresponsive to light; that eye also exhibits ptosis and ventrolateral strabismus and will not adduct well on testing normal nystagmus. These three clinical signs are caused by the loss of function of the GSE-LMNs in the oculomotor nerve, as described in Chapter 6.
Fig. 7-2 shows a 9-year-old male malamute that was presented for asymmetry of the pupils, the only clinical complaint. The neurologic examination was normal except for the eyes. Menace responses were normal in both eyes. There was a persistent anisocoria in room light, with the left pupil always being more dilated than the right pupil. Light directed into the patient’s left eye caused the right pupil to constrict but not the left pupil. Light directed into the right eye caused only the right pupil to constrict, not the left pupil. There is a slight ptosis in the left eye but no strabismus. On testing for normal nystagmus, when the head was moved to the dog’s right, the left eye would not adduct as far as the right eye would when the head was moved to the dog’s left side. Your anatomic diagnosis should be as follows: left cranial nerve III, with complete dysfunction of the GVE-LMNs and some loss of the GSE neurons to the medial rectus and levator palpebrae superioris. You must consider a lesion from the origin of this nerve on the ventral aspect of the midbrain to its terminal branching within the periorbita. Remember the superficial location of the GVE preganglionic neuronal axons and their susceptibility to compressive lesions in the cranial cavity. This could be direct compression resulting from an extraparenchymal neoplasm adjacent to this nerve, or it could be indirect compression resulting from a prosencephalic neoplasm that is causing a shift in the position of the midbrain. In the presence of either of these lesions, you would expect to see other neurologic signs, especially with indirect compression. Be aware that what this dog exhibits may be the first indication of an expanding neoplasm in the middle cranial fossa. We have seen this with germ cell neoplasms and meningiomas but not with pituitary neoplasms. The middle cranial fossa is a common site for a germ cell neoplasm, which is also more common in young dogs from 6 months to 3 years of age.47 About 6 months later, this malamute had generalized seizures, and computed tomography (CT) imaging was performed (Fig. 7-3). Figs. 7-4 through 7-6 show magnetic resonance (MR) images and the gross lesions of canine germ cell neoplasms.
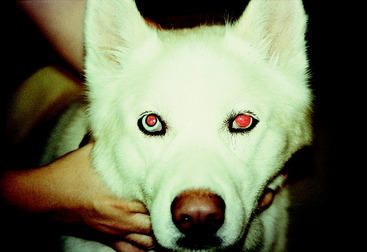
Figure 7-2 A 9-year-old male malamute with a dilated, unresponsive left pupil but normal menace responses bilaterally. Note the slight ptosis in the left eye. There was poor adduction of the left eye when tested for normal nystagmus. The anatomic diagnosis is left oculomotor nerve dysfunction with complete GVE neuronal deficit and partial GSE neuronal deficit. About 6 months later generalized seizures occurred, and computed tomography imaging was performed (see Fig. 7-3).
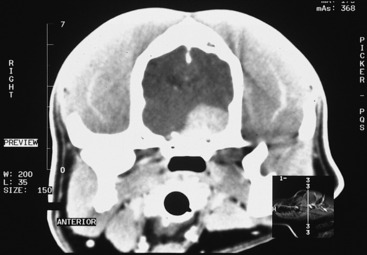
Figure 7-3 Contrast-enhanced CT image of the dog in Fig. 7-2 at the level of the mid-diencephalon and middle cranial fossa. It shows a large contrast-enhancing mass on the left (right side of figure), which is presumed to be a meningioma on the basis of its degree of contrast enhancement and its broad association with the bone of the cranium.
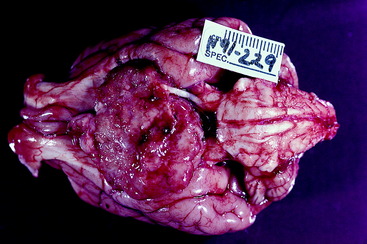
Figure 7-5 Gross appearance of a germ cell neoplasm in a 4-year-old golden retriever with clinical signs similar to those in the dog in Fig. 7-4.
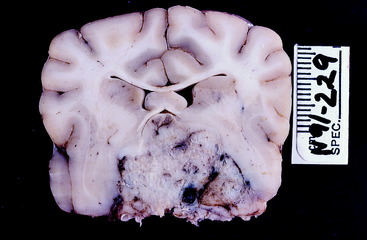
Figure 7-6 Transverse section of the preserved brain of the dog in Fig. 7-5 at the level of the diencephalon. The mass has obliterated the optic chiasm. The white structures in the center that form the shape of an inverted V are the columns of the fornix.
There is a pharmacologic testing procedure that can be used to try to determine whether the GVE-LMN dysfunction is in the preganglionic or postganglionic neuron.4 It involves the denervation hypersensitivity that would be present only if the postganglionic neuron was affected. This hypersensitivity concept is similar to that which is the basis for the denervation potentials (fibrillations and positive sharp waves) seen in electromyograms that occur in skeletal muscle when that muscle has lost its GSE innervation. This pharmacologic testing involves exposing the eye to direct- and indirect-acting parasympathomimetic drugs. The direct-acting drugs elicit a more rapid and more complete response in the hypersensitive denervated muscle (postganglionic lesion), whereas the indirect-acting drugs require the postganglionic neurons to be intact and able to release their endogenous neurotransmitter at the sphincter muscle in the iris. This is a timed response and, in our opinion, is not reliable. Imaging procedures are available, and we strongly recommend their use in locating lesions in this pupillary light reflex pathway.
Sympathetic Innervation of the Eye
Anatomy
The preganglionic neuronal cell bodies are located in the lateral gray column of the first three or four thoracic spinal cord segments (see Fig. 7-1). The axons pass through the ventral gray column and adjacent white matter to join the ventral roots of these segments and the proximal portion of the segmental spinal nerve. Just beyond where the spinal nerve emerges from the intervertebral foramen and before it branches, these preganglionic neuronal axons leave the spinal nerve in the segmental ramus communicans, which joins the thoracic sympathetic trunk. The latter courses longitudinally inside the thorax along the vertebral bodies. The preganglionic neuronal axons destined for the head usually do not synapse in a trunk ganglion but continue cranially in the trunk, pass through the cervicothoracic and middle cervical ganglia, and course cranially in the neck in the cervical sympathetic trunk. This trunk is associated with the vagus nerve forming the vagosympathetic trunk, which is located in the carotid sheath (see Fig. 7-25).
At the head, medial to the origin of the digastricus muscle and ventromedial to the tympanic bulla, the cervical sympathetic trunk separates from the vagus nerve and terminates in the cranial cervical ganglion. Here the preganglionic neuronal telodendria synapse on the dendritic zones of the neuronal cell bodies of the postganglionic neuronal axons. The pathway from this ganglion to the ocular structures is not well defined.1,3,41,46 As a rule, these postganglionic sympathetic neuronal axons follow the blood vessels to the effector organs. A 1932 description of this pathway in the cat showed these axons to pass through the tympanic bulla on the ventral surface of the petrous portion of the temporal bone, but it is not clear whether these are sympathetic postganglionic axons or the parasympathetic preganglionic axons of the glossopharyngeal nerve that form the tympanic plexus known to course in this position.41 It is also possible that the sympathetic postganglionic axons may join this plexus. From this location in the tympanic cavity, these axons enter the cranial cavity ventral to the trigeminal ganglion and join the ophthalmic nerve from the trigeminal nerve, which enters the orbit within the periorbita. Here, these sympathetic axons innervate the orbitalis muscle, which is the smooth muscle in the periorbita and the eyelids, including the third eyelid, the smooth ciliaris muscle, and the smooth dilator muscle of the pupil in the iris (Fig. 7-7). This pathway correlates well with our clinical experience of recognizing a paralysis of these muscles (Horner syndrome) in the presence of otitis media in small animals and trigeminal neuritis in dogs.
In addition to this GVE-LMN, note in Fig. 7-1 the CNS tract that is labeled lateral tectotegmentospinal system. This is an upper motor neuron (UMN) system that is part of the CNS portion of the autonomic nervous system. It actually begins in the hypothalamus and has components that continue caudally in the brainstem; it then continues in the lateral funiculus of the spinal cord. It terminates in the lateral gray column from the T1 to L4, L5 spinal cord segments, where the UMNs synapse on the preganglionic cell bodies of the sympathetic GVE-LMN system. The activity of this UMN system is necessary for the GVE-LMN to function. Noxious stimuli or emotional responses may cause dilation of the pupils. Noxious stimuli to the trunk and limbs utilize spinothalamic and spinotectal pathways to reach the sympathetic centers in the hypothalamus and activate the UMN that projects to the appropriate preganglionic sympathetic neurons in the cranial thoracic spinal cord to cause pupillary dilation. Emotional responses utilize corticohypothalamic pathways to activate this sympathetic pathway. Some texts refer to this sympathetic innervation as a three-order neuron system, with the first-order neuron being the UMN pathway that we just described. The second-order neuron is the preganglionic neuron; the third-order neuron is the postganglionic neuron. This is a confusing concept that is unnecessary for understanding the anatomic diagnosis, so it will not be utilized in this text.
Clinical Signs
The smooth muscle of the eye that is innervated by the sympathetic postganglionic axons includes the orbitalis muscle, and the dilator pupillae (see Fig. 7-7). The orbitalis muscle consists of three sheets. A sheet of circular fibers in the periorbita makes the eyeball protrude. A ventral sheet of longitudinal fibers extends from the sheath of the ventral rectus muscle to the lower eyelid and the third eyelid. A medial sheet of longitudinal fibers extends from the sheath of the medial rectus muscle and the trochlea into the upper eyelid and the third eyelid. The ventral and medial sheets retract the eyelids. Loss of this innervation causes the signs that are often referred to as Horner syndrome, including a smaller pupil (miosis), a smaller palpebral fissure (ptosis is the droop of the upper eyelid), a protruded third eyelid, and enophthalmos that is mild and difficult to appreciate (Figs. 7-8 through 7-11). Be aware that a marked enophthalmos is most commonly associated with atrophy of the muscles of mastication that form a significant portion of the border of the periorbita. With this atrophy, the eyeball sinks into the orbit and as a result, the palpebral fissure is smaller and the third eyelid passively protrudes. These clinical signs can be mistaken for sympathetic paralysis, except that there is no miosis. In some dogs with Horner syndrome, there may be congestion in the bulbar conjunctiva due to lack of vasoconstriction—a “red eye”—and a fundic exam may show congested retinal blood vessels. The miotic pupil, despite loss of sympathetic innervation to the dilator of the iris, can still dilate slightly when the room light is reduced and the iris constrictor muscle relaxes. The anisocoria may be more pronounced if you examine your patient in reduced light. In addition to these clinical signs related to the eyeball and extraocular structures, the sympathetic paralysis in the head causes peripheral vasodilation that may be detected by noting increased warmth of the skin on the affected side as well as pinkness where the skin is not pigmented, in the oral mucosa and external ear canal. Nasal mucosal vasocongestion will decrease the volume of airflow in the ipsilateral nasal cavity. In all domestic animals except the horse, there is decreased sweating on the affected side. This hypohydrosis, or anhydrosis, is difficult to appreciate except in the planum nasolabiale of the ox (see Fig. 7-13).42
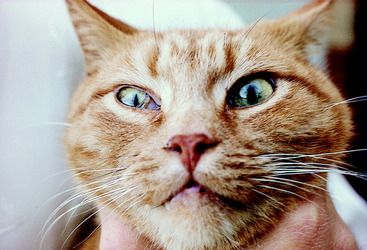
Figure 7-8 Horner syndrome in an adult domestic shorthair with a palpable right thyroid adenocarcinoma.
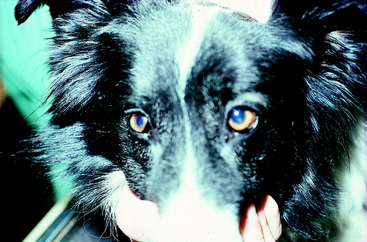
Figure 7-11 Horner syndrome in a 3-year-old border collie with an acute onset of right hemiplegia, with LMN paralysis of the right thoracic limb and UMN paralysis of the right pelvic limb due to fibrocartilaginous embolic myelopathy. Refer to Video 10-43 and Case Example 10-11.
In cattle, sheep, and goats, the most consistent and reliable clinical signs of loss of the GVE-LMN sympathetic innervation to the head are the smaller palpebral fissure and the palpable hyperthermia of the ear. The miosis and protrusion of the third eyelid are often very subtle. In cattle there is less sweating in the planum nasolabiale on the affected side (Figs. 7-12 and 7-13).42 If you warm the muzzle with a heat lamp, this anhydrosis will be more pronounced. The vasodilation in the nasal mucosa may narrow the airway enough on the affected side to be felt on expiration by placing your hand in front of the naris or recognizing the lack of mist on a cold morning (Fig. 7-14). The cow in this photo can be seen in Video 7-1. She is a 12-year-old Holstein cow from a farm in upstate New York. The farmer called for assistance because he astutely noticed that on cold mornings, there was less mist coming from her right nostril, and she also had developed a large swelling on the right side of her neck. About 2 months previously, he had treated this cow for what he assumed to be milk fever by administering calcium gluconate into the external jugular vein. The swelling had been slowly enlarging over the past few weeks. Note the clinical signs of Horner syndrome exhibited by this cow. It was assumed that the swelling in the neck was an infection associated with the treatment the farmer had administered—that at least some of the calcium gluconate had been injected into the perivascular tissues. The infection involved the cervical sympathetic trunk in the carotid sheath, causing the Horner syndrome. This cow also probably had a right laryngeal hemiplegia, which was not detected. It would have been caused by the involvement of the recurrent laryngeal nerve GSE axons contained in the right vagus nerve.
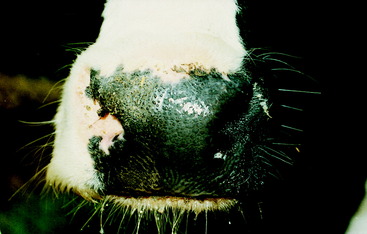
Figure 7-13 The planum nasolabiale of the cow in Fig. 7-12, showing the anhydrosis of the right side.
The horse is unique among the domestic animals in that a sympathetic paralysis causes excessive sweating in the area of skin that is denervated.16,25,38,42 This is often the first clinical sign that is observed, especially if the disorder is acute. An example that most equine practitioners have experienced at some point in their careers and is common in the veterinary college teaching environment relates to the intended intravenous administration of drugs into the external jugular vein. If in this process, some hemorrhage occurs in the adjacent carotid sheath, or if the drug is injected into the sheath, the sudden interference with the sympathetic trunk neurons causes sympathetic paralysis of the head and cranial neck, and almost as soon as the needle is removed from the tissues, the horse starts to sweat from the end of its nose to about the most cranial 15 cm of the neck.
If the paralysis persists, the sweating may decrease with time. In chronic cases it may be present only at the base of the ear. As in ruminants, the smaller palpebral fissure will be obvious, the miosis will be mild, and the protrusion of the third eyelid may be very subtle or not visible (Figs. 7-15 through 7-17). An additional indication of loss of sympathetic innervation to the head is the decrease in the angle of the eyelashes of the upper eyelids, which is associated with the loss of upper eyelid elevation and the loss of innervation to the arrector ciliorum muscles. This difference between the eyelashes of the two eyes can be seen if you elevate the head slightly and observe the eyelashes from the median plane of the head. The loss of sympathetic innervation can be an early sign of equine grass sickness in the countries where that disorder occurs. However, that disease typically affects the eyelash angle bilaterally. To confirm the loss of sympathetic innervation, you can apply a few drops of 0.5% phenylephrine to one eye.19 This is an alpha-1 adrenergic agonist that will rapidly correct the eyelash angle associated with this disorder (Fig. 7-18). Presumably, this eyelash response occurs because of denervation hypersensitivity.
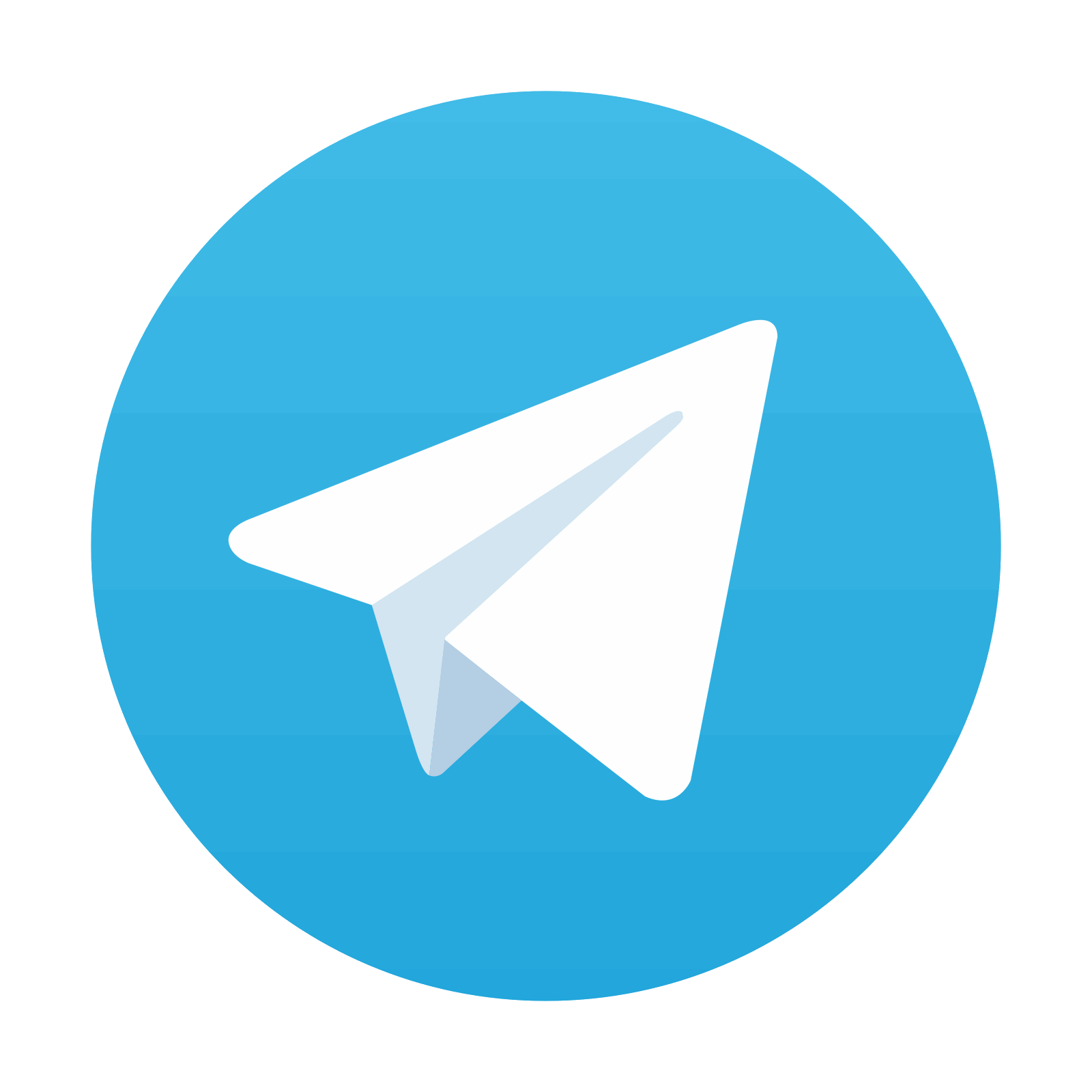
Stay updated, free articles. Join our Telegram channel

Full access? Get Clinical Tree
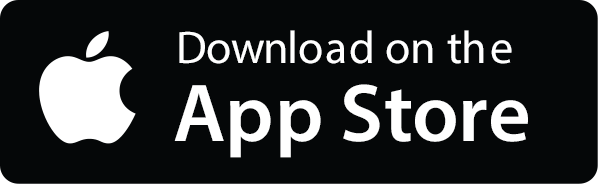
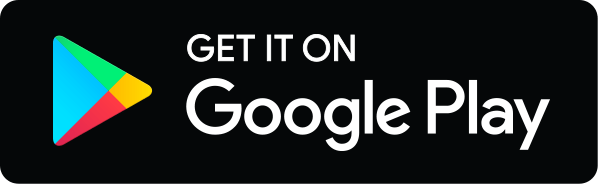