Introduction
Local anesthetics are a group of chemically related compounds that reversibly bind sodium channels and block impulse conduction in nerve fibers. The interruption of neural transmission in sensory afferent nerves or tracts by a local anesthetic drug after local tissue infiltration, regional nerve blocks, or epidural or intrathecal (subarachnoid) injection uniquely and most effectively prevents or reduces pain or nociceptive input during and after surgery. Analgesia in the desensitized area is not only complete by such techniques, but it also removes the immediate secondary (central) sensitization to pain and reduces the central facilitation of the nociceptive pathway.
The use of a local anesthetic is essential if surgery is to be performed in a conscious patient and the pain associated with trauma and inflammation is to be relieved. The use of a local anesthetic technique before surgery may also benefit patients by avoiding general anesthesia or reducing the amount of general anesthetic. Sustained analgesia into the recovery period is a great benefit to patients when a local anesthetic with a longer anesthetic effect is used. Knowledge of the clinical pharmacology of individual local anesthetics enables the achievement of effective and safe neural blockade. Local anesthetic techniques discussed herein have their own particular rate of onset, duration, and risk of complication.
The conduction of impulses in excitable membranes requires a flow of sodium ions through selective sodium channels into the nerve in response to depolarization of the nerve membrane. Mammalian voltage-gated sodium channels consist of one large alpha subunit that contains four homologous domains (D1–D4), each with six putative α-helical transmembrane segments (S1–S6) and one or two smaller auxiliary beta subunits. Under resting conditions, sodium ions are at a higher concentration outside than inside the nerve, and a voltage difference across the axonal membrane, known as resting potential, of 270 mV exists. When the nerve is stimulated, the permeability of the membrane to sodium ions increases transiently, and sodium passes through the membrane by way of sodium-selective ionic channels that exist in various conformations (i.e., resting, open, or inactivated), depolarizing the plasma membrane. During depolarization, the action potential moves in obligatory fashion along the axon, allowing for impulse propagation along the nerve membrane. After a few milliseconds, the membrane repolarizes as a result of inactivation or “closing” of the sodium channels. During repolarization, the membrane is no longer permeable to sodium ions, but potassium channels open, and potassium ions flow down their electrochemical gradient out of the cell.
Figure 10.1. Site of action of amino-ester and amino-amide local anesthetics. The uncharged base form diffuses most readily across the lipid barriers and interacts at the intermembrane portion of the sodium channel. The charged form (BH+) gains access to a specific receptor via the axoplasmic surface of the sodium channel pore. Modified from Carpenter and Mackey, p. 414, with permission.

The precise mode of action of local anesthetic drugs is unknown, but perhaps the combination membrane-expansion and specific-receptor theory is most accepted. In this theory, the quaternary ammonium compounds (amides) and ester local anesthetics first pass through the cell membrane as the uncharged base (B) to reach the intracellular site where the uncharged base is protonated and the charged cation (conjugated acid, BH+) binds to the receptor and “plugs” the channel (Figure 10.1). Perhaps best accepted is the idea that local anesthetics bind to sodium-selective ionic channels in nerves, inhibiting the sodium permeability that underlies action potential and depolarization of the cell membrane. Electrical transmission through a myelinated axon stops when enough concentration of the anesthetic is applied to bathe at least three consecutive nodes of Ranvier.
Frequency-dependent block
Local anesthetics may differ in their ability to bind to sodium channels, depending on the channel status. Open ion-conducting and inactivated sodium channels have a greater local anesthetic affinity than resting, nonconducting sodium channels. Repetitive stimulation of nerve fibers increases the binding affinity of the receptor site for local anesthetics and facilitates the development of neural blockade, a phenomenon called use-dependent or frequency-dependent block, or phasic block.
Other mechanisms of action
Local anesthetics will bind to many different sites that may contain a variety of different sodium channels. The sodium channels in the heart, brain, and axons are not identical. However, if local anesthetics achieve a sufficient tissue concentration, they will affect all excitable membranes, including those that exist in the heart, brain, and neuromuscular junction. The molecular mechanisms by which local anesthetics produce epidural or spinal (subarachnoid) analgesia may include local anesthetic binding to sodium and potassium channels within the dorsal and ventral horns and binding to neural calcium channels, which causes hyperpolarization of cell membranes. Alterations in membrane calcium ion (Ca2+) may be responsible for deformation or expansion of the cell membrane and thus the transmission or conduction of nerve impulses. Local anesthetics may inhibit substance P binding and evoke increases in intracellular calcium (Ca2+), and potentiate γ-aminobutyric acid (GABA)-mediated chloride currents by inhibiting GABA uptake.
Antimicrobial activity
Additional and sometimes controversial benefits of local anesthetics, which are routinely administered before minor skin surgery and for postoperative pain relief, include both a potent antimicrobial effect and improved wound healing. Administration of 1%, 2%, and 4% lidocaine demonstrates a dose-dependent inhibition of growth for all strains of bacteria tested (e.g., Staphylococcus aureus, Escherichia coli, Pseudomonas aeruginosa, and Enterococcus faecalis) and no change in the susceptibility of the bacteria to lidocaine by the addition of epinephrine. Likewise, administration of 2% and 5% lidocaine and 1% prilocaine demonstrates a powerful antimicrobial effect on various bacteria, including E. coli, S. aureus, P. aeruginosa, and Candida albicans. In contrast, 0.25% and 0.5% bupivacaine shows poor antimicrobial effectiveness, and ropivacaine has no antimicrobial effect on such microorganisms.
All local anesthetics contain an aromatic ring at one end of the molecule and an amine at the other, separated by a hydrocarbon chain (Table 10.1). The aromatic end is derived from benzoic acid or aniline and is lipophilic. The amine end is derived from ethyl alcohol or acetic acid and is hydrophilic. Substitution of alkyl groups on the aromatic ring or amine end increases lipid solubility and potency.
Chirality
In general, local anesthetics are supplied commercially as racemic mixtures of both R-(+) and S-(–) optical stereoisomers. Differences in structure result in various pharmacodynamic and pharmacokinetic actions. Ropivacaine is provided as the hydrochloride of the pure S-(–) enantiomer. It is associated with a reduced incidence of both cardiovascular and central nervous system (CNS) toxicity, a concern with use of racemic bupivacaine. In addition, epidural ropivacaine is similar to bupivacaine in onset, depth, duration, and extent of sensory blockade, although motor block is less intense and briefer.
Levobupivacaine is a pure S-(–) enantiomer similar to ropivacaine, and is also less toxic than bupivacaine, which is attributable to a lesser affinity for brain and myocardial tissue than either that of the R-(+) enantiomer or racemic bupivacaine.
Chemical grouping
The clinically useful local anesthetic drugs essentially segregate into amino esters and amino amides, based on the chemical link between the aromatic moiety and the hydrocarbon chain (Table 10.1). Amino esters have an ester link, and the amino amides have an amide link, respectively. The nature of linkage (ester vs. amide) has a notable effect on the chemical stability and the route of metabolism. Ester-linked local anesthetics include cocaine, benzocaine, procaine, chloroprocaine, and tetracaine. Most esters are readily hydrolyzed by plasma cholinesterase and have short half-lives when stored in solution without preservatives. Amide-linked local anesthetics include lidocaine, prilocaine, dibucaine, etidocaine, mepivacaine, bupivacaine, levobupivacaine, ropivacaine, and articaine. The amide agents are very stable, cannot be hydrolyzed by cholinesterase, and rely on enzymatic degradation in the liver. The amide structure of articaine is similar to that of other local anesthetics but contains an additional ester group, which is quickly hydrolyzed by esterases, shortening its duration of action. Ropivacaine and levobupivacaine are synthesized as single S-(–) optical isomers. Other local anesthetics exist as racemates or have no asymmetrical carbons.
The clinical action of local anesthetics may be described by their lipid solubility, anesthetic potency, speed of onset, duration of action, and tendency for differential block. These properties do not sort independently and their relevancy to each other can be compared in Table 10.2.
Controversy still surrounds the differential susceptibility of nerve fibers to local anesthetics and its relation to selective functional deficit. It is apparent that differential block of impulses in nerve fibers exists, varying among anatomical features (different peripheral nerves, fiber diameter, presence or absence of myelination, and surrounding tissue), different local anesthetics, critical duration of drug exposure (absorption, distribution, and elimination of drug from the site of injection), and different animal species (e.g., frogs, rats, cats, and people). Sensory and motor fibers have a characteristic neurophysiological profile, motor and sensory function, and conduction-block susceptibility (Table 10.3).
Table 10.1. Trade names, chemical structure, and main clinical uses of ester-linked and amide-linked local anesthetic agents


Table 10.2. Physical, chemical, and biologic properties of currently available local anesthetic agents

a The potency given is relative to procaine.
ND, not determined.
In situ studies
Local anesthetics block nociceptive fibers (small unmyelinated C fibers and myelinated A-δ fibers) more readily and before other sensory and motor fibers (large myelinated A-γ, A-β, and A-α fibers) (Table 10.3). Local anesthetics block small-diameter myelinated or unmyelinated fibers at a lower concentration than is required to block large fibers of the same type. This is probably attributable to the longer action potentials and the discharge at higher frequencies of smaller fibers.
Differential brachial plexus blockade
Brachial plexus block in dogs produces more rapid onset of motor block when compared with sensory block after the administration of bupivacaine (0.375% with 5 mg/mL epinephrine, 4 mg/kg) (9.7 vs. 26.2 minutes, mean values). This phenomenon is explained by the somatotopical arrangement of nerve fibers such that the motor fibers would be located at the periphery of the nerve trunk (mantle bundles) and the sensory fibers in the center (or core) (Figure 10.2). Consequently, if sufficient analgesic drug is applied to produce motor blockade, the diffusion of the analgesic or its transport into the nerve by the local blood supply will first affect the motor fibers.
Table 10.3. Classification of nerve fibers and order of blockade

Figure 10.2. Somatosensory arrangement of nerve fibers in the trunk of the brachial plexus of the dog. Nerve fibers in the mantle or peripheral bundles innervate primarily motor fibers of the proximal limb, whereas nerve fibers in the core or center bundles innervate for the most part the sensory fibers of the distal foot. The concentration gradient that develops during initial diffusion of local anesthetic into the nerve trunk causes onset of anesthesia to proceed from proximal to distal. Recovery from anesthesia also proceeds from proximal to distal because of absorption of local anesthetic into the circulation surrounding the nerve trunk.

Differential epidural and spinal blockade
In general, progression of epidural and spinal anesthesia is related to the diameter, myelination, and conduction velocity of affected nerve fibers. Exposure of mixed-nerve trunks within the spinal vertebral column to a sufficient concentration of an analgesic drug might cause a loss of sensation in this order: pain, heat and cold, touch, proprioception, and skeletal muscle tone. Recovery of sensation is expected to be in the reverse order.
Autonomic small unmyelinated C fibers and myelinated B fibers seem to be readily desensitized after epidural or spinal administration of local anesthetics. Spinal anesthesia is generally characterized by preganglionic sympathetic nerve blockade (B fibers) that extends further than sensory block (A-δ fibers), and sensory block extends further than somatic motor block (A-α fibers).
Sensory anesthesia sufficient for surgery usually cannot be obtained without motor impairment. Adequate sensory analgesia with little or no motor blockade can be achieved with the epidural administration of low concentrations of bupivacaine or ropivacaine combined with opioids and/or alpha2 agonists. Epidural blockade may be able to differentiate between sympathetic, somatic, and central pain in patients with chronic pain.
A variety of factors can influence the quality of regional anesthesia, including local anesthetic dose, site of administration, additives such as epinephrine or hyaluronidase, pH adjustment and carbonation, baricity, temperature, mixtures of local anesthetics, and altered physiology (such as pregnancy).
Dose of local anesthetic
A greater dose (volume and/or concentration) will facilitate overall efficacy, thereby decreasing the delay of onset of action and increasing both the likelihood of successful anesthesia and its duration. The potential of systemic toxicity by inadvertent intravenous (IV) injection or neurotoxicity precludes the routine administration of large doses of local anesthetic.
All local anesthetics can be neurotoxic, particularly in concentrations and doses larger than those used clinically. Large-scale surveys, using histopathologic, electrophysiological, behavioral, and neuronal cell models, indicate that lidocaine and tetracaine seem to have a greater potential for neurotoxicity than does bupivacaine at clinically relevant concentrations.
Volume of local anesthetic
Generally, a larger volume of local anesthetic will produce a faster and denser block. An exception to this rule is articaine, which readily penetrates tissues and produces anesthesia in approximately 2 minutes, irrespective of the volume injected. The necessity of a larger injected volume of anesthetic solution for a high rate of complete sensory block can be minimized during plexus blocks in dogs if an adequate concentration of a local anesthetic agent (e.g., 1% mepivacaine or 0.375% bupivacaine) is precisely administered at multiple injection sites covering all major nerves of the brachial plexus.
Concentration of local anesthetic
A higher concentration of local anesthetic will also produce a faster and denser block. Increasing the concentration of lidocaine and bupivacaine during phasic (“use dependent”) inhibition of sodium currents increases the rate of binding but has no effect on unbinding sodium channels. In general, the chance for successful desensitization and anesthesia decreases when the concentration is lowered. One study suggests that lumbar epidural anesthesia with 10 mL of 2% lidocaine in humans produces more intense blockade of large-diameter and small-diameter sensory nerve fibers than that with 20 mL of 1% lidocaine. Similarly, administration of 0.75% ropivacaine into the lumbar epidural space of dogs produces a higher rate of complete anesthesia than does 0.5% ropivacaine of similar volume (0.22 mL/kg).
Injection site
In general, the fastest onset (within 3–5 minutes) and shortest duration (1 hour) of anesthesia is usually produced after subcutaneous and intrathecal injections of 2% lidocaine or mepivacaine hydrochloride solution, followed in order of increasing onset time for minor nerve blocks (5–10 minutes), major nerve blocks, and epidural anesthesia (10–20 minutes).
Additives
Vasoconstrictors
As a general rule, the addition of a vasoconstrictor to a local anesthetic agent, such as epinephrine, allows for decreased local perfusion, delayed rate of vascular absorption of local anesthetic, and therefore increased intensity and prolonged anesthetic activity. Lumbar epidural anesthesia, using 10 mL of 1% lidocaine with epinephrine 1:200,000 produces a more intense block of both large-diameter and small-diameter sensory nerve fibers than that achieved with lidocaine alone.
The usual concentration of epinephrine is 5 mcg/mL or 1:200,000 (1 mg/200 mL of saline), which may be obtained by adding 0.1 mL of 1:1000 (0.1 mg) epinephrine to 20 mL of local anesthetic solution. Alternatively, 1:1000 epinephrine may be diluted with preservative-free normal saline. The maximum safe concentration of epinephrine is 1:50,000; concentrations less than 1:200,000 are less effective. Market preparations of local anesthetics that contain epinephrine 1:200,000 have a lower pH (to slow oxidation of the epinephrine) than do plain solutions; for example, 2% lidocaine without and with epinephrine 1:200,000 has a pH of 6.78 and 4.55; and 0.5% bupivacaine without and with epinephrine (1:200,000) has a pH of 6.04 and 3.73, respectively. The low pH of the epinephrine preparations will potentially decrease the amount of free protonated anesthetic base available for diffusion through the axonal membrane, thereby slowing the onset of action.
Epinephrine effects depend on the injection site and the local anesthetic, but, in general, it reduces the potential toxicity of local anesthetics by causing vasoconstriction and thus preventing higher blood concentrations. Acidic epinephrine-containing local anesthetic solutions can decrease the pH at the site of injection, depending on the buffer demand of the injectate and the buffer capacity of the tissue. Epinephrine should not be added to local anesthetics intended for nerve blocks that have an erratic blood supply and for intravenous regional anesthesia (IVRA) with use of a tourniquet because it can cause nerve ischemia and prolonged blockade. Epinephrine often causes tissue necrosis along wound edges. Norepinephrine and phenylephrine appear to have no clinical advantage over epinephrine.
Hyaluronidase
Hyaluronidase depolymerizes hyaluronic acid, the tissue cement or ground substance of the mesenchyme, aiding in the spread of a local anesthetic injection. The increased permeability of tissues may enhance systemic absorption (and toxicity) but shortens the duration of anesthetic effects because more drug is available in base form. The addition of 5 IU of hyaluronidase/mL of 1% lidocaine with 1:200,000 epinephrine solution in a standard dose and technique for ophthalmic surgery (2 mL as retrobulbar injection for intraocular anesthesia, 2 mL for upper-eyelid anesthesia, and 4 mL for extraorbital facial nerve blockade) reportedly does not increase the systemic absorption and cerebrospinal fluid (CSF) concentration of lidocaine in dogs. However, administration of hyaluronidase does not seem to enhance the efficacy of newer local anesthetics with improved spreading power (e.g., articaine and ropivacaine). Administration of 2% articaine or 1% ropivacaine produces a faster onset of anesthesia and less pain on injection than does administration of 1% bupivacaine.
pH adjustment and carbonation
The pH of the local anesthetic solution affects the local distribution of the anesthetic. Extracellular increase of bicarbonate increases the cross-membrane pH gradient, the intracellular concentration of the ionized local anesthetic, and local anesthetic effects. The addition of sodium bicarbonate to procaine, chloroprocaine, mepivacaine, or lidocaine will shorten the onset of nerve block, enhance the density of block, and prolong the duration of block in isolated nerve preparations. This is likely because the amount of nonionized base increases, which enhances diffusion of the local anesthetic through axonal membranes and ion trapping due to the increased cross-membrane pH gradient.
The efficacy of alkalinization depends on the local anesthetic and regional block techniques. The addition of sodium bicarbonate for median nerve block in humans decreases the pain on injection and increases the rate of onset of motor block, but has no effect on duration of sensory anesthesia. Similarly, adjusting the pH of 1% lidocaine or 0.25% bupivacaine with sodium bicarbonate to 7.4 has little effect on duration of anesthesia after injection into the infraorbital foramen or abdominal musculature. Alkalinization produces the best results with 2% lidocaine and 0.5% bupivacaine for epidural block, with 2% lidocaine for axillary brachial plexus block, and with 2% mepivacaine for sciatic and femoral nerve blocks. Bicarbonate has minimal effects when added to ropivacaine.
Local anesthetic solutions may deteriorate over time with the addition of bicarbonate. Solutions of lidocaine and 2-chloroprocaine readily alkalinize to near physiological pH without precipitation. Mepivacaine 1.5% precipitates above neutral pH within 20 minutes. Bupivacaine and etidocaine precipitate after the addition of small amounts of sodium bicarbonate and cannot be alkalinized to physiological pH. Mixtures should be used within 20 minutes of their preparation.
Baricity
This is defined as the calculated ratio of the density of a solution to the density of CSF. One of the most important physical properties affecting the spread of local anesthetic solutions and level of analgesia achieved after intrathecal administration of a local anesthetic is its density relative to the density of CSF at 37°C.
Density is the weight of a unit volume of solution (grams per milliliter) at a specific temperature, whereas the specific gravity (SG) is the calculated ratio of the density of a solution (x) to the known density of water (y), (SG = x/y). The density of a drug in solution cannot be determined from a simple formula because it depends on the physical state of that substance in solution. The density of intrathecal agents is usually compared with the density of the CSF. At room temperature, most glucose-free drugs are isobaric with respect to CSF, but as drugs warm to body temperature they become relatively hypobaric. The densities of 2% lidocaine and 0.5% and 0.75% bupivacaine, for example, are slightly less than that of normal range of CSF in humans and therefore can be considered slightly hypobaric. Continued dilution of 0.75% bupivacaine with water produces increasingly hypobaric solutions. Hypobaric solutions have a baricity less than that of CSF and will migrate to nondependent areas during and immediately after the injection. Glucose-free 0.5% bupivacaine acts as a hypobaric solution, which produces a higher level of analgesia in the nondependent side compared with the dependent side in patients positioned laterally. The unpredictability of extent of spinal block provided by spinal bupivacaine (0.5%) and tetracaine (0.5%) may be related to individual variations in CSF densities. Patients with higher CSF densities demonstrate a higher spinal block after administration of bupivacaine (0.5%, 3 mL).
Dextrose and hypertonic saline-containing local anesthetic solutions (e.g., tetracaine in 10% glucose and dibucaine in 5% hyperbaric saline) have an SG greater than that of CSF. They will migrate from the site of injection to dependent areas. Hyperbaric solutions are created by combining local anesthetics (e.g., 0.5% bupivacaine or 0.5% ropivacaine) with an equal volume of 10% dextrose, producing final drug and dextrose concentrations of 0.25% and 5%, respectively.
Temperature
The cooling of mammalian nerves in vitro slows the conduction velocity and increases the susceptibility to local anesthetic inhibition of transmission. The potency of local anesthetics increases in vitro and in vivo with cooling in some instances but not in others. Inhibition of C fibers (as assessed by galvanic skin potentials) is marginally faster when ice-cold lidocaine (1%) is used compared with room-temperature lidocaine (1%) for median nerve blocks in volunteers. Cooling of lidocaine increases its pKa and the relative amount of the protonated (active) form within lipid, thereby potentiating the anesthetic effect. On the other hand, a decrease in temperature from 37 to 20°C decreases the uptake of lidocaine in mammalian sciatic nerve by 45%. It is unlikely that cooling of local anesthetics (5°C) before injection of small volumes (5 mL) will be of any effect under clinical conditions because of rapid warming of the local anesthetic by the surrounding tissue, preventing the nerve itself from growing cold.
Pregnancy
Pregnant women with lidocaine (1%)-induced median nerve block at the wrist have a greater decrease in sensory nerve action potential than do nonpregnant women, indicating that pregnancy increases median nerve susceptibility to lidocaine desensitization. Similarly, isolated vagus nerves removed from pregnant rabbits are more susceptible to bupivacaine-induced conduction block than are nerve fibers from nonpregnant animals. Progesterone administration to nonpregnant rabbits replicates the increased local anesthetic susceptibility of pregnancy. Distention of the lumbar epidural venous plexus during pregnancy may displace the local anesthetic solution to more cranial regions of the spinal canal. Therefore, to prevent excessive cranial spread of anesthesia, a reduced dose of epidural and spinal anesthetics during pregnancy is recommended.
Local anesthetics, as the name implies, are deposited at or near the desired site of action. In general, local anesthetics are injected near a nerve bundle. Intraneural injection is painful and may cause nerve damage. The factors that determine the distribution of local anesthetic near the injection site are illustrated in Figure 10.3. Most clinically used local anesthetics are weak bases and are supplied as mildly acidic hydrochloride salts to improve solubility and stability. In solution, local anesthetics exist as nonionized base (B) and ionized cation (BH+). The nonionized anesthetic (B) diffuses across the tissue barriers and into the axonal nerve membrane, where membrane stability and neural blockade occur. Nonspecific binding of anesthetic in connective tissue, fat, and muscles and absorption of anesthetic into the vascular and lymph systems reduce the mass (volume × concentration) of the anesthetic available at the neural tissue. Once absorbed into the bloodstream, the local anesthetic is distributed to the lungs, where a significant part (20–30%) is absorbed, depending mainly on the physicochemical properties of the local anesthetic. After back diffusion of the anesthetic from the lung into the blood, the anesthetic is distributed to systemic tissues (e.g., brain, heart, and liver) and is metabolized in the liver to compounds that are primarily excreted by the kidney and bile.
Figure 10.3. Factors that determine the diffusion of local anesthetic near the site of injection and within the body. The nonionized base of the anesthetic (B) diffuses into the axon and nonspecific tissues. Nonspecific tissue binding and absorption into the bloodstream reduce the mass of drug available to diffuse into neural tissue.

Absorption
Systemic absorption of local anesthetics is determined primarily by the drug dose (volume or concentration), duration of effect at the site of action, vascularity of the injection site, and use of a vasoconstrictor. Local anesthetic solutions generally are ineffective when applied to the intact skin.
Topical application of local anesthetics includes transdermal patches, creams, and iontophoretic delivery systems. Proparacaine is the topical local anesthetic most commonly used in veterinary medicine. After application of a lidocaine patch (Lidoderm Patch, Endo Pharmaceuticals, Chadds Ford, PA) a sufficient amount of 5% lidocaine penetrates the human intact skin to produce analgesia in patients with neuropathic pain associated with postherpetic neuralgia and in patients with postthoracotomy and postmastectomy pain, but less than the amount necessary to produce a complete sensory block. A single patch (10 × 14-cm adhesive bandage) contains 700mg of lidocaine, which is used in a 12-hour-on and 12-hour-off period to minimize systemic absorption.
The eutectic mixture of 2.5% lidocaine and 2.5% prilocaine (e.g., EMLA® Cream, AstraZeneca Pharmaceuticals LP, Wilmington, DE) contains 25 mg of lidocaine and 25 mg of prilocaine per each gram or milliliter, which has a sufficiently high concentration of readily available anesthetic base and high water content to penetrate the intact skin and produce reliable cutaneous anesthesia for a wide range of applications in people (e.g., venipuncture in children, radial artery cannulation in adults, and laser treatment) and for venipuncture in dogs, cats, rabbits, and rats. In humans, EMLA preparation is applied as a thick layer under an occlusive dressing, and maximum depth of analgesia of approximately 5 mm is achieved for 30 minutes after a 90-minute application and for a 60-minute period after a 120-minute application of the cream. In general, the depth of cutaneous analgesia in people ranges from 1 to 6 mm and is believed to be time dependent (1–4 hours).
Amethocaine cream 1 g (5% wt/wt) applied for 30 or 60 minutes on the dorsum of the hand produces good analgesia for venous cannulation similar to analgesia produced by 5% EMLA Cream (2.5 g) applied for 30 or 60 minutes. In comparison to the gel preparation, a 30-minute application of the amethocaine-patch system provides profound topical anesthesia of human skin that lasts longer than a 60-minute application of EMLA (3–6 hours vs. 20 minutes).
ELA-Max Cream (PD-Rx Pharmaceuticals Inc., Oklahoma City, OK) is designed to produce analgesia of the skin to reduce venipuncture pain in 20–30 minutes in children without the use of an occlusive dressing. The preparation contains 4% lidocaine, which is liposome encapsulated to enable fast penetration into the stratum corneum. It remains in the epidermis after absorption and minimizes the rapid metabolism of lidocaine. ELA Max Cream lacks the active ingredient of prilocaine, which has been associated with methemoglobinemia (MHG) in infants. Accordingly, ELA-Max Cream may be more suitable for use in cats susceptible to methemoglobin (MHb) formation.
Numby Stuff® patches (Iomed Inc., Salt Lake City, UT), which consist of 2% lidocaine and 1:100,000 epinephrine, are percutaneously administered through iontophoretic drug administration by using a battery generator to deliver a small electrical current (4 mA) through two small electrodes. Each application device delivers 1 mL of the anesthetic to a depth of up to 10 mm in 10 minutes, giving a transient blanching and tingling of the skin
Transdermal local anesthesia achieved by iontophoresis reduces the pain of IV injection of hyperosmolar saline. This technique involves the use 0.5 mL of lidocaine (2–4%) with or without epinephrine 1:50,000 and a current intensity of 0.1–0.2 mA/cm2 at the anode and placement of the cathode on the dorsal surface of the forearm for 10 minutes.
Although veterinary use of Lidoderm patches, ELA-Max Cream, Numby Stuff patches, and transdermal local anesthesia by iontophoresis is minimal to date, potential uses include local analgesia for small wound repair, venipuncture, or catheter placement (IV and epidural).
Distribution
Distribution of local anesthetic at the injection site depends on the volume of local anesthetic injected, inclusion of a vasoconstrictor or hyaluronidase in the local anesthetic solution, and the specific drug employed. The SG (baricity) of the solution relative to the SG of the CSF influences distribution within the CNS.
The distribution of amino-ester local anesthetics (e.g., procaine, chloroprocaine, and tetracaine) in body tissues is limited because of their rapid enzymatic hydrolysis by nonspecific plasma pseudocholinesterases. Amide-type local anesthetics (e.g., lidocaine, mepivacaine, prilocaine, bupivacaine, levobupivacaine, etidocaine, and ropivacaine) are widely distributed after IV bolus injection or a fast rate of vascular absorption. Their pharmacokinetic properties are usually described by a two-or three-compartment model.
In blood, all amide-linked local anesthetics are partially protein bound, primarily to α1-acid glycoprotein (AAG) and, to a lesser extent, to albumin. In general, protein binding of local anesthetics is positively correlated with the degree of ionization in the physiological pH range and the drug’s potency. Plasma protein binding of local anesthetics ranges from 6% for the least potent and short-acting procaine to 95% for the more potent and longer-persisting bupivacaine, etidocaine, and ropivacaine (Table 10.2)
Free drug concentration in plasma, not the protein-bound concentration of drug, governs tissue concentrations. The effect of serum protein binding on lidocaine distribution into the brain and CNS in dogs after IV lidocaine administration indicates that free or unbound fraction of lidocaine is an important determinant of lidocaine entry into the brain and CSF. Protein binding of lidocaine in dogs that receive a loading dose (2 mg/kg) and a maintenance infusion (50 mcg/kg/h) of lidocaine is associated with increased protein binding and only slight increases of free plasma concentrations of lidocaine.
In dogs, the concentration of lidocaine bound to AAG varies considerably, and is higher in dogs with inflammatory disease. Local anesthetic protein binding approaches saturation only at very high drug concentrations, primarily after prolonged infusion of a long-acting local anesthetic (e.g., ropivacaine or levobupivacaine) and local anesthetic–opioid combination to provide prolonged postoperative analgesia. The slow rise in total plasma concentration with increasing duration of infusion of ropivacaine and levobupivacaine appears to be the predominant reason for rare complications related to systemic toxicity produced by these drugs.
Biotransformation and excretion
The liver and lungs are major sites for plasma clearance of local anesthetics. Metabolism converts relatively lipid-soluble local anesthetics into more water-soluble metabolites.
For esters, the primary step is ester hydrolysis, catalyzed by nonspecific plasma cholinesterases. The rate of plasma hydrolysis is rapid, yielding half-lives measured in seconds, and is inversely related to toxicity (chloroprocaine [most rapid] > procaine > tetracaine [least rapid]).
Procaine and benzocaine are metabolized to paraaminobenzoic acid (PABA), a breakdown product responsible for allergic reactions and anaphylaxis in some human patients. The majority of the PABA is excreted unchanged or as conjugated product in the urine. Chloroprocaine and tetracaine are metabolized similarly, but not to PABA. Cocaine is an atypical ester in that it undergoes either ester hydrolysis or N-demethylation to norcocaine and then ester hydrolysis and significant hepatic metabolism and urinary excretion. Cocaine is rarely used in veterinary medicine, although it can be abused for stimulation of horses before a race. Ester metabolism can, theoretically, be slowed by reduced cholinesterase activity during pregnancy and long-term cholinesterase inhibition via poisons, thereby prolonging the clearance of ester anesthetics and increasing the potential for toxicity.
The amino-amide local anesthetics undergo nearly exclusive metabolism by the liver and hepatic degradation, which requires conjugation with glucuronic acid. Cats glucuronidate drugs to a lesser extent than dogs, making cats more prone to develop toxic side effects when given amide local anesthetics.
The order of clearance of amides is prilocaine (most rapid) > etidocaine > lidocaine > mepivacaine or ropivacaine > bupivacaine (least rapid). Lidocaine undergoes oxidative N-dealkylation by cytochrome P4503A4. Mepivacaine, etidocaine, bupivacaine, and ropivacaine also undergo N-dealkylation and hydroxylation. They are further conjugated with glucuronide before they are excreted from the body via the urine or bile. Prilocaine undergoes hydrolysis to o-toluidine, a compound that can oxidize hemoglobin to MHb.
Since all amide local anesthetics are metabolized by the liver, drug clearance is highly dependent on hepatic blood flow, hepatic extraction, and enzyme function. Clearance of amide local anesthetics can be reduced or prolonged by factors that decrease hepatic blood flow, such as β-adrenergic or H2-receptor blockers, by hypotension during regional and general anesthesia, or by heart or liver failure.
When careful technique and appropriate dose are used, local anesthetics are relatively free of harmful side effects. However, local anesthetics may cause severe toxic reactions after unintentional IV administration, vascular absorption of an excessive dose (large volume or high concentration) of the local anesthetic agent, or ingestion of topical local anesthetic preparations.
Doses of local anesthetics, especially those for cats and small dogs, should always be carefully calculated and reduced in sick animals. For example, in healthy dogs and cats, the dose of lidocaine should not exceed 10 and 4 mg/kg, respectively, to prevent toxicity. Repeated applications, the application of higher than the recommended doses, or impaired elimination may all contribute to increasing blood concentration of local anesthetics. Potential damage may also occur from chemical contamination of the local anesthetic solution, allergic reactions, or MHG, or from neural ischemia produced by local pressure or hypotension. The systemic toxicity of local anesthetics involves primarily the CNS and the cardiovascular system.
CNS toxicity
In general, toxic and lethal doses of local anesthetic drugs produce signs of CNS excitation, leading ultimately to convulsive activity followed by CNS depression (unconsciousness and coma) with eventual respiratory arrest and cardiovascular collapse. Acute CNS toxicity occurs at lower doses than those required to produce acute cardiovascular system toxicity.
As the plasma concentration of the drug increases, humans experience a predictable sequence of signs and symptoms, such as numbness of tongue, light-headedness, visual disturbance, muscle twitching, unconsciousness, and convulsions, which may progress to coma, respiratory arrest, cardiovascular depression, and death. In small animals, low concentrations of local anesthetics produce sedation, whereas higher concentrations produce seizures, probably because of selective depression of inhibitory fibers in the subcortical area (amygdala), with subsequent spread, leading to grand mal seizures. Muscle twitching and convulsions are usually the first signs of local anesthetic toxicity observed in dogs and cats. More potent local anesthetics consistently produce seizures at lower blood concentrations and lower doses than do the less potent local anesthetics.
In awake dogs, the mean cumulative dose of serially and rapidly administered IV local anesthetics to produce convulsions is 4.0 mg/kg tetracaine, 5.0 mg/kg bupivacaine, 8.0 mg/kg etidocaine, and 22 mg/kg lidocaine, indicating a relative CNS toxicity of tetracaine, bupivacaine, etidocaine, and lidocaine of about 1:1.2:2:4.
In cats, procaine (the least potent CNS depressant, less lipid soluble and less protein bound) produces seizures at 35 mg/kg IV, whereas bupivacaine (one of the most potent CNS depressants, highly lipid soluble and highly protein bound) induces convulsions at approximately 5 mg/kg IV. IV-administered lidocaine at a dose of 11.7 ± 4.6mg/kg causes seizures in cats. Increased arterial PCO2 (68 to 81 mm Hg) and decreased pH reportedly decrease the convulsive dose of procaine, lidocaine, and bupivacaine by approximately 50% in cats. The toxic effects of local anesthetics within the CNS are enhanced by increased cerebral blood flow, by increased concentration of ionized drug in the brain, or by the direct excitatory effect on subcortical structures.
Cardiovascular toxicity
Since an alarming editorial in 1979 about cardiac arrest in humans following regional anesthesia with etidocaine and bupivacaine, ropivacaine and, recently, levobupivacaine were developed as alternative long-acting amide local anesthetics with less potential for cardiovascular toxicity.
Local anesthetic cardiovascular toxicity may result from direct electrophysiological and mechanical effects on the heart or the peripheral circulation and from local anesthetic actions on the autonomic nervous system. The use of lower concentrations can result in CNS excitation, with increased heart rate, arterial blood pressure, pulmonary artery pressure, and cardiac output. With larger toxic blood concentrations, the systemic effects are characterized by decreased heart rate, arterial blood pressure, pulmonary artery pressure, and cardiac output.
Results from animal studies demonstrate increased systemic toxicity associated with bupivacaine and etidocaine as compared with lidocaine, the most extreme of which include severe CNS and cardiovascular reactions, eventually leading to hemodynamic instability, cardiovascular collapse, and death. Local anesthetics bind and inhibit cardiac sodium channels. Bupivacaine binds more readily and for a longer duration to cardiac sodium channels than does lidocaine. The bupivacaine S-(–) isomer binds cardiac sodium channels less readily than does the R-(+) isomer, forming the basis for the development of levobupivacaine and ropivacaine.
Toxicosis after ingestion or topical application
Topical local anesthetic preparations containing lidocaine, benzocaine, tetracaine, and dibucaine, which are in many prescription and nonprescription products, such as ointments, teething gels, suppositories, and aerosols, can be hazardous to animals if ingested. Ingestion of topical benzocaine preparations or spray before endotracheal intubation has produced varying degrees of vomiting, cyanosis, dyspnea, respiratory depression, prolonged sedation, hypotension, cardiac arrhythmias, tremors, seizures, and even death in dogs and cats. Likewise, digestion of ointments and creams containing 0.5% and 1.0% dibucaine hydrochloride, which may not be considered dangerous by pet owners, has produced salivation, vomiting, hypothermia, bradycardia, hypotension, weakness, seizures, dysrhythmia, and death in dogs and cats. Between 1995 and 1999 the National Animal Poison Control Center (NAPCC) recorded over 70 cases of toxicosis induced by either ingestion or the inappropriate use of lidocaine, benzocaine, or dibucaine in a variety of animals, including dogs, cats, and ferrets. The clinical signs included salivation, vomiting, hypothermia, depression, tremors, weakness, bradycardia, hypotension, and seizures.
Local toxicity
Neurotoxicity
When properly used, local anesthetics rarely produce local neurotoxic effects or localized tissue damage. Neurotoxicity of local anesthetics can be demonstrated in vitro by the collapse of growth cones and neuritis in cultured neurons. Comparison of seven local anesthetics in a study on growing neurons of the freshwater snail demonstrates neurotoxicity in this order: procaine = mepivacaine (least neurotoxic) < ropivacaine = bupivacaine < lidocaine < tetracaine < dibucaine (most neurotoxic).
Myotoxicity
All clinically used local anesthetics are myotoxic, with a drug-specific and dose-dependent rate of toxicity that worsens with serial or continuous administration. Some reports indicate that single and repeated injections of clinical doses of mepivacaine or bupivacaine produce skeletal muscle damage in rats. The administration of bupivacaine and ropivacaine induces Ca2+ release of the sarcoplasmic reticulum and simultaneously inhibits Ca2+ reuptake into the sarcoplasmic reticulum, suggesting an important mechanism in observed myotoxicity. Although skeletal muscle damage from local anesthetics is not a major clinical problem, case reports have been published of local anesthetic-induced myotoxicity in humans after local and regional anesthesia, peripheral nerve blocks, retrobulbar injections, and trigger-point infiltration for treatment of myofascial pain.
MHG
MHG or increased concentration of MHb in the blood is defined as an altered state of hemoglobin whereby the ferrous form of iron (Fe2+) is oxidized to the ferric state (Fe3+), which increases oxygen affinity for hemoglobin (as seen with MHG) and reduces oxygen release at tissues. In addition, oxidative denaturation of hemoglobin can cause Heinz body formation, which can lead to erythrocyte lysis. MHG can cause hypoxia, cyanosis, or even death.
A number of local anesthetic agents, most notably benzocaine and prilocaine, and less often procaine and lidocaine, are implicated as causative agents. Benzocaine induces MHG in several species, whereas lidocaine may increase MHG in cats and people. It appears that these agents do not directly produce MHG, but rather a metabolite, o toluidine, is responsible.
An intense chocolate brown-colored blood and central cyanosis unresponsive to the administration of 100% oxygen suggest the diagnosis of MHG. MHb concentrations of 15% or more cause a brown discoloration to the blood, which is visible on a white paper towel. Laboratory confirmation is by blood co-oximetry, indicating an MHb concentration of greater than 15% (1–2% is normal).
The clinical consequences of MHG are related to the blood concentration of MHb. In humans, dyspnea, nausea, and tachycardia occur at an MHb concentration of >30%, whereas lethargy, stupor, and deteriorating consciousness occur as the MHb concentration approaches 55%. Acquired toxic MHG has been induced with benzocaine topical anesthetics (spray, cream, and ointment). Peak MHb concentrations are directly related to the total dose of benzocaine or prilocaine administered.
MHG has been induced by the nasal, oropharyngeal, and dermal applications of benzocaine in sheep, dogs, and cats. A 2-second spray of benzocaine (estimated dose, 56 mg) to the mucous membranes of the nasopharynx of dogs, cats, monkeys, rabbits, and miniature pigs produces MHb concentrations ranging from 3.5% to 38%, 15–60 minutes after drug administration.
Benzocaine is combined with butamben and tetracaine in a topical anesthetic spray (Cetacaine, Cetylite Industries, Inc., Pennsauken, NJ) that has been commonly used to desensitize the larynx before intubation. Cats are at an increased risk for developing MHG and Heinz body anemia with benzocaine-containing products, including Cetacaine. Because of the susceptibility of cats, ferrets, or other exotic animals to MHG, the topical use of Cetacaine should be avoided.
Allergic reactions
Although allergic reactions to local anesthetics may occur, they are uncommon and often misdiagnosed after accidental IV injection of local anesthetics. True anaphylaxis or life-threatening allergic immune reaction is mediated by immunospecific antibodies (immunoglobulins E or G) that interact with mast cells, basophils, or the complement system to liberate vasoactive mediators and recruit other inflammatory cells. Such reactions have been documented with amino ester local anesthetics (e.g., procaine), particularly those that are metabolized directly to PABA, which is a common allergen. Anaphylaxis to amide local anesthetics (e.g., lidocaine) is much less common. Some reaction may result from hypersensitivity to a preservative (e.g., methylparaben, whose chemical structure is similar to that of PABA). Allergic reactions of dogs and cats treated with amide-linked local anesthetics are very rare.
Anaphylactoid-adverse drug reactions may mimic anaphylaxis, characterized by bronchospasm, upper airway edema, vasodilatation, increased capillary permeability, and cutaneous wheal and flare. Rapid cardiopulmonary intervention with airway maintenance, epinephrine administration, and volume expansion is essential to avoid a fatal outcome.
Treatment of adverse reactions
When local anesthetic-induced convulsions occur, hypoxia, hypercarbia, and acidosis can develop rapidly. Because these metabolic changes greatly increase the toxicity of local anesthetics, prompt therapy with oxygen administration by mask to a dyspneic patient and supporting ventilation (e.g., endotracheal intubation, oxygen supplementation, and positive-pressure ventilation) is indicated.
Anticonvulsant drug therapy is indicated if seizure activity interferes with ventilation or is prolonged. Diazepam or midazolam can be given IV in dogs and cats at 0.5–1 mg/kg in increments of 5–10 mg to effect, with minimal side effects. Thiopental or propofol (1–2 mg/kg IV) acts more rapidly but may produce greater cardiorespiratory depression than that produced with benzodiazepine therapy.
Acute hypotension may be treated with IV fluids (10 mL/kg/h) and vasopressors (phenylephrine, 0.5–5.0mcg/kg/min, or norepinephrine, 0.02–0.2mcg/kg/min). Caution must be exercised to avoid intravascular volume overload, which can produce pulmonary edema, pleural effusion, mucous membrane discoloration, pigmenturia, vomiting, and neurological abnormalities, particularly in small dogs and cats.
An IV bolus of epinephrine (1–15 mcg/kg) may be required in presence of myocardial failure. Guidelines for cardiopulmonary resuscitation should be followed when toxicity progresses to cardiac arrest. Animal studies suggest that lidocaine intoxication causes myocardial depression that can be successfully treated with continued advanced cardiac life support. In contrast, cardiotoxic effects associated with incremental overdosage of bupivacaine, levobupivacaine, or ropivacaine are not as easily treated by resuscitation efforts. The administration of epinephrine may lead to severe arrhythmias, including ventricular fibrillation, with a subsequent mortality rate from bupivacaine, levobupivacaine, ropivacaine, and lidocaine of approximately 50%, 30%, 10%, and 0%, respectively. Early bupivacaine overdose (inadvertent IV injection) and toxicity is best treated with a lipid-containing product (Lyposyn 20% Intravenous Lipid, Hospira, Inc., Lake Forest, IL) capable of absorbing bupivacaine and lowering bupivacaine plasma concentrations rapidly.
Even though the risk of cardiovascular toxicity of ropivacaine has not been completely eliminated, ropivacaine may offer clear advantages over bupivacaine, in that ropivacaine accumulates less sodium-channel blockade at physiological heart rates, dissociates from sodium channels more rapidly, is less cardiotoxic, and is more susceptible to treatment than is bupivacaine.
Ventricular dysrhythmias, including life-threatening ventricular tachycardia associated with local anesthetic toxicity in dogs, have been treated with bretylium tosylate (2–6 mg/kg IV). Procainamide and quinidine may be effective treatments for ventricular arrhythmias in dogs and cats. Lidocaine is generally contraindicated in patients with amide local anesthetic toxicity.
MHG is easily treated and rapidly reduced to hemoglobin by slow (over several minutes) IV administration of a 1% solution of methylene blue (methylthioninium chloride, 4 mg/kg in dogs and 1–2 mg/kg in cats). The dose can be repeated in dogs, but repeated administration of methylene blue in cats is controversial because of the risk of Heinz-body anemia and the aggravation of subsequent hemolysis without further lowering MHb content.
Tachyphylaxis, or the acute tolerance to local anesthetic agents, is defined as decreases in intensity, segmental spread, or duration after repeated administration of equal doses of an anesthetic. Various ester-type local anesthetics (e.g., cocaine, procaine, and tetracaine) and amide-type local anesthetics (e.g., lidocaine, mepivacaine, bupivacaine, etidocaine, and dibucaine) have been used at increasing doses to maintain a similar level of effect during surface anesthesia, nerve blocks, brachial plexus block, and epidural and subarachnoid anesthesia. The underlying mechanisms of tachyphylaxis are not well understood. Local alterations in disposition and absorption of local anesthetics (but not in structure [ester vs. amide] or the pharmacological properties of local anesthetics themselves [e.g., brief vs. long acting]), technique, mode of administration (intermittent vs. continuous), and pharmacodynamic processes (interactions at receptor sites) may all play a role in the development of tachyphylaxis.
Local and regional analgesic techniques: dogs
The popularity of local anesthetic-induced neural blockade in dogs has increased over the past several years. A major driving force behind this increased usage is acceptance of the concept of blocking multimodal pathways to control animals’ pain and suffering. Unlike most general anesthetics, which block the perception of pain by inducing unconsciousness, local anesthesia and regional anesthesia completely block the transmission of noxious impulses in a region of the body of a conscious patient. Local and regional anesthesia also decreases the quantity of opioid and inhalation anesthetic required to obtain the desired plane of anesthesia intraoperatively. Topical anesthesia, infiltration anesthesia, field blocks, selected nerve blocks of the head (anesthesia of the maxilla, upper teeth, eye and orbit, mandible, and lower teeth), anesthesia of the foot and leg (ring block, brachial plexus block, and IVRA), multiple intercostal nerve blocks, lumbosacral epidural anesthesia, and continuous epidural anesthesia provide surgical analgesia and anesthesia in dogs that are considered at risk for inhalant or IV anesthesia. Continuous interpleural analgesia and epidural opioid analgesia can also be used to relieve postoperative pain following general anesthesia.
Topical anesthesia
Many local anesthetics are effective when placed topically on mucous membranes and may be used in the mouth, airway, esophagus, and genitourinary tract. Local anesthetics used topically include lidocaine (2–5%), proparacaine (0.5%), tetracaine (0.5–2.0%), butacaine (2%), and cocaine (4–10%). Preparations that are applied topically come in creams, ointments, jelly, powders, and aerosols. Injectable preparations of lidocaine (0.5–5.0%) with and without epinephrine can also be applied topically to mucous membranes (1–5%). Topical local anesthetic agents can relieve pain during cleaning or dressing of wounds, although their effect is highly variable. The lowest effective dose of topical anesthetic should always be used in order to prevent toxicity from excessive plasma concentrations. Time between application of topical anesthetics and onset of anesthesia is generally longer, and pain relief less, than that achieved with infiltration anesthesia. A 2–4% solution of lidocaine used for topical anesthesia on mucous membranes produces effects in approximately 5 minutes and lasts for 30 minutes.
Local instillation of proparacaine (0.5%), tetracaine (0.5–1.0%), butacaine (2%), piperocaine (2%), oxybuprocaine (0.4%), or cocaine (1–4%) into the conjunctival sac anesthetizes the cornea and conjunctiva for short procedures (e.g., removal of hypertrophied gland of the third eyelid). Proparacaine (0.5%) has been advocated as an excellent topical anesthetic for examination of a painful eye, removal of foreign bodies, sutures, obtaining conjunctival scrapings, and subconjunctival injections. Anesthesia occurs rapidly (1–6 minutes), lasts for 10–15 minutes after single instillation, and may last for up to 2 hours after repeated instillation without untoward effects (e.g., irritation or epithelial damage). A series of three to five instillations of one or two drops of proparacaine at approximately 1-minute intervals may be necessary to produce satisfactory anesthesia of the cornea and conjunctiva. Topical anesthesia is very safe, is simple to apply, and can be repeated, although dogs may resent the application of cold solutions. Data on vascular uptake and maximum blood concentration are not available, large interpatient variability should be expected, and potential for bacterial contamination exists.
Local anesthetic sprays (10% lidocaine or 14–20% benzocaine) anesthetize the mucosa up to a depth of 2 mm within 1–2 minutes after application. Anesthesia lasts for approximately 15–20 minutes. The movable nozzle of the spray can enable easy access to the site of application. Pressure on the nozzle with the forefinger delivers a specific quantity of the anesthetic each second (10 mg of lidocaine from a 10% lidocaine spray can). The average expulsion rate from a benzocaine (Cetacaine) spray is 200 mg/s.
Endotracheal tubes are frequently coated with local anesthetic jells but should not be lubricated with jelly containing 20% benzocaine hydrochloride. Topical sprays and ointments containing 14–20% benzocaine cause dose-dependent MHG. Preparations with over 8% benzocaine include Hurricane Spray (20%) (Beutlich Pharmaceuticals LP, Waukegan, IL), Hurricane Topical Anesthetic Gel (20%) and Liquid (20%) (Beutlich Pharmaceuticals), Camphophenique Sting Relief Formula (20%) (Bayer Health Care LLC. Morristown, NJ), Dermoplast Anesthetic Pain Relief (20%) (PrestigeBrands, Inc., Irvington, NJ), and Cetacaine Spray (14%) (Cetylite Industries, Inc., Pennsauken, NJ). Exposure of the tracheal mucosa to topical benzocaine oxidizes blood hemoglobin in dogs in proportion to the absorbed dose within 10 minutes. MHb cannot bind oxygen or carbon dioxide. Dogs are usually asymptomatic when concentrations of MHb are less than 20%, but show fatigue, weakness, dyspnea, and tachycardia at concentrations between 20% and 50%. Laryngeal sprays containing benzocaine should be used with caution, and if signs of cyanosis and respiratory distress develop, MHG should be considered. In general, benzocaine should be used sparingly and cautiously while continuously monitoring for cyanosis. Dogs at risk of hypoxia after using benzocaine topical anesthesia should receive oxygen and IV methylene blue (1.5 mg/kg) therapy.
One of the oldest forms of topical anesthesia is superficial cooling. Ethyl chloride can be used to freeze a small local area of skin for punctures, skin biopsy, or incision of small abscesses. Ethyl chloride is sprayed on the skin for 3–7 seconds from an inverted bottle and a distance of 10–20 cm, with the jet stream aimed so that it meets the skin at an acute angle to lessen the shock of impact. Surface anesthesia results from cooling (<4°C), which occurs during the evaporation process. Attempts to freeze large skin areas by using ethyl chloride are contraindicated because of the potential for frostbite. Ethyl chloride’s brief action (<2 minutes), ability to produce a freezing sensation, and flammability when exposed to open flames and electric sparks (electrocauterization) limit its use. Inhalation of ethyl chloride should be avoided because it may produce narcotic and general anesthetic effects, or fatal coma with respiratory and cardiac arrest.
Pontocaine cream and a liposomal tetracaine preparation (0.5% tetracaine encapsulated into phospholipid vehicles) effectively penetrate human skin within 30–60 minutes of application, producing long-lasting (>4 hours) analgesia. The most clinically usable cream contains a 5% eutectic mixture of 2.5% lidocaine and 2.5% prilocaine (EMLA cream), which overcomes the human stratum corneum barrier within 1 hour of topical application without adverse effects. Lidoderm patches have been used to reduce pain associated with surgical incision in dogs.
Infiltration anesthesia
Infiltration of local anesthetics requires their extravascular placement by direct injection and may be the most reliable and safest of all the local anesthetic techniques. Lidocaine (0.5–2.0%) is the local anesthetic most often used for infiltration. Only sharp and sterile needles should be used. Local anesthesia can be produced by multiple intradermal or subcutaneous injections of 0.3–0.5 mL of local anesthetic solution by using a 2.5-cm, 22–25-gauge needle or by using a longer needle (3.75–5.0 cm) and slowly injecting local anesthetic while advancing the needle along the line of proposed incision (linear infiltration). Pain is minimal if the needle is advanced slowly into the first desensitized wheal and successive injections are made at the periphery of the advancing wheal. This technique assures that the dog senses only the initial needle insertion. Intradermal deposition of local anesthetic over a superficial abscess, cyst, or hematoma is a routine procedure. Infection along the filtration site will not occur if the needle has not entered the abscess. The amount of local anesthetic used for infiltration anesthesia depends on the size of the area to be anesthetized. Approximately 2–5 mg/kg of lidocaine or mepivacaine and 4–6 mg/kg of procaine without epinephrine may be used to diffuse into surrounding tissue from the site of injection and anesthetize the nerve fibers and endings. Large amounts of relatively dilute solutions are often infiltrated into operative sites. The lowest possible concentration of local anesthetic that will produce the desired effect should be administered. For example, an average dog (20 kg) will tolerate approximately 50 mL of 0.5% lidocaine without demonstrating signs of toxicity, whereas only 20–30 mL of 1% lidocaine or 10–15 mL of 2% lidocaine can be injected. The local anesthetic may be diluted in 0.9% sodium chloride solution (not with sterile water) to a 0.25% solution if a large volume of local anesthetic is needed for infiltration of a large operative area. The total dose of drug administered should be reduced by 30–40% in old dogs (>8 years) and sick or cachectic dogs in poor condition.
Continuous-infiltration anesthesia
This can be accomplished by the use of a continuous catheter-insertion system and a disposable infusion pump. A sterile multipore catheter is placed within the surgical incision (e.g., total ear canal ablation with lateral bulla osteotomy, forelimb amputation, or median and lateral thoracotomies) at the end of the surgical procedure. The catheter is connected to an elastomeric reservoir infusion pump, which is filled with local anesthetic (i.e., lidocaine, mepivacaine, or ropivacaine) to its full capacity (65, 100, 270, or 335 mL) to deliver the local anesthetic at a constant rate (0.5, 2.0, 4.0, or 5.0 mL/h) for several days. This technique is generally well tolerated, producing good postoperative analgesia for up to 50 hours, with no acute local anesthetic toxicity, hemodynamic instability, or breakthrough pain. Early signs of toxicity, such as nystagmus, restlessness, apprehension, and vomiting, are readily treated by removing the pump.
Field block
This technique can be used for anesthetizing large areas. First, intradermal or subcutaneous linear infiltration is produced around the lesion as previously described. Local anesthetic is then deposited in the deeper tissues by passing the needle through the desensitized skin far enough to infiltrate the deep nerves supplying the area (Figure 10.4).
Regional nerve blocks
Injection of local anesthetic solution into the connective tissue surrounding a nerve produces loss of sensation (sensory nerve block) and/or paralysis (motor nerve block) in the region supplied by the nerves (regional anesthesia). Smaller volumes (1–2 mL) of local anesthetic are needed to produce nerve blocks when compared with a field block, thereby reducing the danger of toxicity.
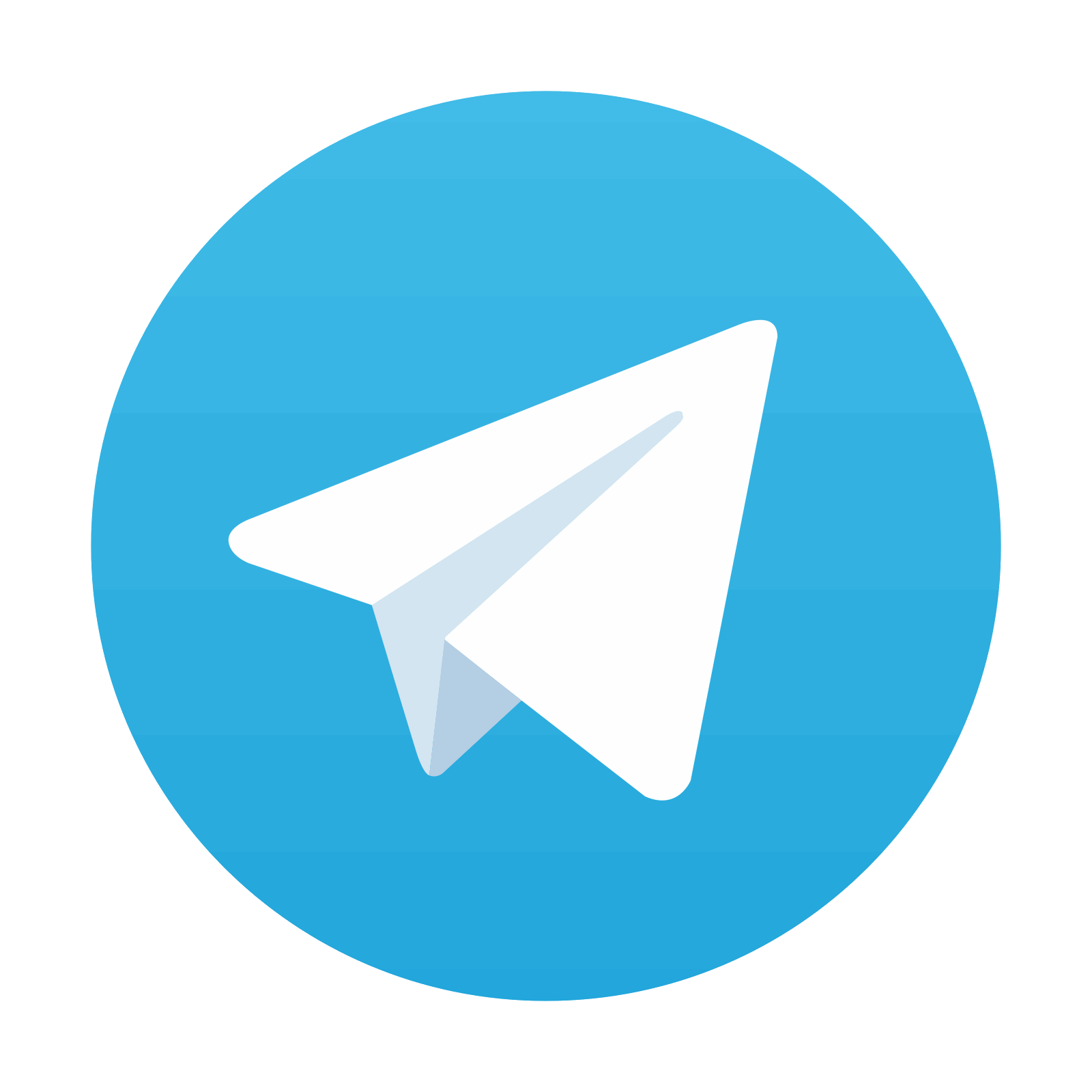
Stay updated, free articles. Join our Telegram channel

Full access? Get Clinical Tree
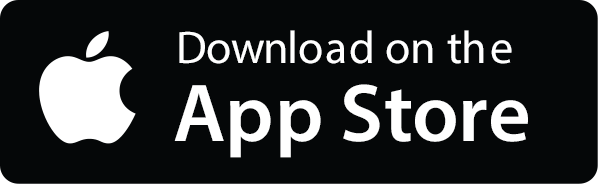
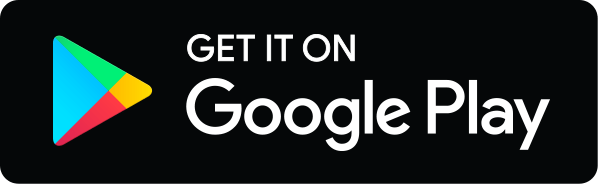