Raquel M. Walton IDEXX Laboratories, Inc., Langhorne, PA, U Acronyms and abbreviations that appear in this chapter include: BCS, body condition score; BM, body mass; DM, diabetes mellitus; GIT, gastrointestinal tract; GLUT, glucose transporter; HDL, high‐density lipoprotein; ID, insulin dysregulation; LPL, lipoprotein lipase; LCFA, long chain fatty acids; LDL, low‐density lipoprotein; NaF, sodium fluoride; NEFA, nonesterified fatty acids; PPID, pituitary pars intermedia dysfunction; PSSM, polysaccharide storage myopathy; VLDL, very low‐density lipoprotein. Lipids perform a variety of roles in the body, ranging from energy storage to serving as major cell membrane components and polar solvents for fat‐soluble vitamin and hormone transport. Of the many lipids present in mammals, only a very few are of clinical and diagnostic relevance. This chapter will discuss the primary lipid abnormalities encountered in equids and the methods for their diagnosis. A preliminary discussion of the types of lipids and their role in metabolism is necessary to adequately understand equine lipid disorders. Hyperlipidemias in horses are largely due to hypertriglyceridemia, so triglyceride metabolism will be emphasized. Triglycerides are synthesized in intestinal enterocytes from three long chain fatty acids (LCFA) and one glycerol molecule that are absorbed following the digestion of dietary fats by pancreatic lipase (Figure 9.1). Within enterocytes, triglycerides are incorporated into chylomicron particles that are made water soluble by a protein and phospholipid coating. Chylomicrons are released first into lymphatics and then into blood via the thoracic duct. Lipoprotein lipase (LPL), produced by adipocytes and myocytes, translocates to the luminal surface of endothelium where it breaks down plasma triglycerides into LCFA and glycerol, which are extracted into fat and muscle. In nonruminants, glucose is the primary building block for LCFA used in triglyceride synthesis; acetate is used for LCFA synthesis in ruminants [1]. Insulin is involved in lipid metabolism by facilitating glucose uptake into adipocytes and myocytes and increasing LPL activity in endothelial tissue. Glucagon, corticosteroids, adrenocorticotropic hormone (ACTH), and epinephrine stimulate hormone‐sensitive lipase in adipocytes to catalyze the hydrolysis of stored triglycerides and release nonesterified fatty acids (NEFA). Hyperinsulinemia promotes triglyceride production and increases adipose tissue, whereas glucagon and corticosteroids counteract these effects. When there is increased release of fatty acids from adipocytes, the NEFA not utilized by peripheral tissues are taken up by hepatocytes to be oxidized for energy, converted into ketones or used to synthesize triglycerides that are either stored or released as very low‐density lipoprotein (VLDL). Thus, increases in circulating fatty acids contribute to hepatic triglyceride storage and hypertriglyceridemia via hepatic VLDL synthesis (Figure 9.2). Lipids are a class of compounds that are soluble in organic solvents and insoluble in water. Lipid hydrolysis produces fatty acids or complex alcohols that can combine with fatty acids to form esters [2]. Hyperlipidemia and hyperlipemia are used synonymously to denote increases in serum or plasma lipid concentrations. By convention, lipemia refers to the gross appearance of serum or plasma when hypertriglyceridemia is present. Figure 9.1 Overview of triglyceride (TG) metabolism. TGs are synthesized in enterocytes or in the liver. TGs of dietary origin are synthesized in the intestine from monoglycerides (MG), long chain fatty acids (FA), and glycerol broken down from dietary fats by pancreatic lipase. Within enterocytes, triglycerides are incorporated into chylomicron (CM) particles that are released first into lymphatics and then into blood via the thoracic duct. In the liver, TGs are synthesized and released as very low‐density lipoproteins (VLDLs). Lipoprotein lipase (LPL) on the luminal surface of endothelium breaks down plasma triglycerides (in the form of chylomicrons or VLDLs) into FA and glycerol, which are taken up by muscle and fat. In nonruminants, glucose is the primary building block for FAs used in triglyceride synthesis. The two principal lipids measured in animals are triglycerides and cholesterol. All triglycerides and the majority of cholesterol are carried within lipoproteins. Lipoproteins are classified according to their relative density, which is dependent upon their composition. The classes of lipoproteins include VLDL, low‐density (LDL), and high‐density (HDL) lipoproteins. The lipoprotein core is hydrophobic and consists of triglyceride and cholesterol ester molecules surrounded by a hydrophilic membrane composed of proteins (apoproteins), phospholipids, and unesterified cholesterol molecules. Lipoproteins are measured after they are separated using electrophoresis or ultracentrifugation. The predominant lipoprotein in horses is HDL, comprising 61% of the total plasma lipoprotein mass, with VLDL and LDL comprising 24% and 15%, respectively. Similar to humans, VLDL is triglyceride rich, whereas LDL is cholesterol rich and HDL is protein rich. Normal concentrations of VLDL and total triglyceride are reported to be higher in Shetland ponies than Thoroughbred horses [3]. Although lipoprotein fractions are not typically measured in veterinary medicine, they may be of diagnostic utility in metabolic syndrome (discussed below) [4]. Glucose is the most important precursor of LCFA in nonruminants, whereas acetate is used in ruminants. As hindgut fermenters, horses absorb short chain fatty acids (acetate, propionate, and butyrate) from insoluble carbohydrates. Horses can readily shift from using glucose‐based LCFA synthesis in diets high in soluble carbohydrates to acetate‐based synthesis when fed high‐roughage diets [5, 6]. Following triglyceride hydrolysis in adipocytes, LCFA are released into circulation as NEFA bound to albumin. Typically, NEFA are produced in response to energy demand, thus increased concentrations are considered an indicator of negative energy balance. Concentrations of NEFA increase with stress, exercise, and low blood glucose as a result of hormonal stimulation of adipocyte lipase (Figure 9.2). Accordingly, care should be taken to minimize excitement and exercise should be restricted in the period immediately before sampling. Figure 9.2 Hormone effects on triglyceride (TG) metabolism. Insulin inhibits the breakdown of TGs into nonesterified fatty acids (NEFA) and promotes triglyceride production and increases adipose tissue. Glucocorticoids, ACTH, glucagon, and catecholamines are insulin antagonists that stimulate hormone‐sensitive lipase to break down TG into NEFA and glycerol. The increased concentrations of NEFA are taken up by hepatocytes to be oxidized for energy, converted into ketones, or used to synthesize triglycerides that are either stored in the liver or released as VLDL. Serum or plasma can be used for measurement but heparin anticoagulant should be avoided since it can increase NEFA concentrations during storage. NEFA concentrations are stable in whole blood for 24 hours and in separated serum or plasma for 72 hours as long as the samples are kept at 4 °C; storage at room temperature results in increased NEFA concentrations. Because of the instability of NEFA concentrations, NEFAs are best measured in situations in which the sample can be kept cool and submitted to a diagnostic laboratory relatively quickly. Circulating triglycerides are present within lipoproteins produced by the liver (VLDL) or intestinal enterocytes (chylomicrons). Triglyceride concentration is preferentially measured in serum and is stable for up to a week at 4 °C, for three months at −20 °C, and for years at −70 °C. Triglycerides are made of three LCFA and a glycerol molecule. Most assays for serum triglycerides use a reaction with a lipase to release glycerol from triglycerides; it is the glycerol that is measured following a coupling reaction with a substance that can be detected spectrophotometrically. Triglycerides should be measured on fasting samples (minimum of 12 hours). Triglycerides are usually reported in mg/dL. To convert into SI units (mmol/L), the concentration in mg/dL is multiplied by a factor of 0.01129. The gross appearance of lipid in plasma or serum, termed lipemia, is only caused by increased concentrations of triglyceride‐rich lipoproteins (chylomicrons and VLDL). Thus, an estimate of triglyceride concentration can be determined visually (Figure 9.3). Serum or plasma that is clear indicates that the triglyceride concentration is <200 mg/dL (2.26 mmol/L); serum with triglyceride concentrations around 300 mg/d: (3.39 mmol/L) appears hazy‐turbid, whereas triglycerides >600 mg/dL (6.77 mmol/L) impart an opaque, milky appearance [2]. It is of interest to note that serum triglyceride concentrations exceeding 500 mg/dL (5.65 mmol/L) have been associated with no visible evidence of lipemia in horses [7]. However, the authors may have ignored a hazy‐turbid appearance as not consistent with the classic milky appearance associated with lipemia. Figure 9.3 Lipemic serum is opaque and white to pink. The pink tinge is commonly due to mild hemolysis that often occurs with lipemic serum. The presence of chylomicrons is indicated when a creamy, homogeneous layer floating on the surface of plasma or serum appears after refrigeration for several hours. If only chylomicrons are present, the serum below the floating lipid layer is clear. In contrast, lipemia attributable to increases in VLDL produces turbid or milky serum that persists after refrigeration. Fasting lipemia is due to the presence of VLDL, whereas postprandial lipemia is associated with chylomicronemia. Measurement of cholesterol includes total cholesterol derived mainly from cholesterol‐rich lipoproteins LDL and HDL. Like triglycerides, cholesterol can be synthesized in the liver or absorbed from dietary sources in the intestine. Cholesterol concentration is stable for a week at 4 °C, for three months at −20 °C, and for years at −70 °C. Measurement involves hydrolysis of cholesterol esters to produce cholesterol. Added cholesterol oxidase produces hydrogen peroxide, which reacts with an indicator dye for spectrophotometric analysis. Cholesterol can be measured in fasted or nonfasted samples. The laboratory definition of hyperlipidemia is an increase in measurable serum lipids, which in horses are usually triglycerides. Diagnosis of hyperlipidemia is dependent upon measurement of serum triglyceride concentration. By convention, equine hyperlipidemias are clinically classified in the following manner: hyperlipidemia is characterized by serum triglyceride concentrations from 100 to 500 mg/dL (1.13–5.65 mmol/L) without gross lipemia or clinical signs; hyperlipemia is defined as a serum triglyceride concentration more than 500 mg/dL (5.65 mmol/L) with visible lipemia and fatty infiltration of the liver and/or other organs [7, 8]. Hyperlipidemia is usually associated with insufficient food intake and is often unnoticed because it is subclinical. These categorizations use 100 mg/dL as the cut‐off for the lower reference value. Different laboratories and different species under varying conditions will have different cut‐off values for hyperlipidemia and hyperlipemia. For example, normal triglyceride concentrations in horses and nonpregnant ponies are often reported as <100 mg/dL, but reference values for ponies during the last trimester of pregnancy concentrations can reach 250 mg/dL (2.83 mmol/L). In one study, concentrations of up to 390 mg/dL (4.4 mmol/L) are reported for healthy donkeys [9]. Thus, hyperlipidemia and hyperlipemia should be defined using the lower end of the reference interval established by the laboratory producing the results. Hyperlipidemia is typically associated with periods of negative energy balance (e.g., hypophagia, pregnancy, lactation), but is also linked to obesity, stress, sex, and breed. The pathogenesis of equine hyperlipemia lies in disturbances in energy metabolism compounded with insulin resistance. Insulin resistance is the cell’s diminished capacity to transport glucose from the blood into peripheral tissues in response to insulin (discussed further in the next section). In the context of insulin resistance, development of a negative energy balance results in excessive and inappropriate mobilization of fatty acids that accumulate in the liver as triglycerides and in the blood as VLDL. The increase in plasma triglyceride and NEFA concentrations can interfere with insulin’s inhibition of hormone‐sensitive lipase, contributing to increased lipolysis. Catecholamines, cortisol, and ACTH, all of which are increased in illness, also stimulate LPL. The hyperlipemia results in accumulation of fatty acid metabolites in myocytes that can interfere with insulin‐mediated glucose transport into cells, enhancing insulin resistance [10]. Concomitant azotemia may inhibit LPL activity, further exacerbating the lipidemia [11]. The ensuing self‐perpetuating hyperlipemia results in tissue lipidosis and ultimately organ dysfunction and failure. Many of the biochemical abnormalities present in hyperlipemia (other than the hypertriglyceridemia itself) can be attributed to either a primary disease process that precipitated hyperlipemia and/or to organ dysfunction/failure caused by lipidosis. There is a high prevalence of hyperlipemia in pony breeds, especially Shetland ponies, miniature horses, and donkeys. Female ponies show a higher prevalence than males, representing 75% or more of cases [8]. In ponies, hyperlipemia is more prevalent in reproductively active mares, with increased incidence in late pregnancy and early lactation. The increased prevalence of hyperlipemia in female miniature breeds and donkeys applies to both reproductively active and inactive mares [8, 9]. Prevalence reports range from 3–5% up to 11% in the general population and are even higher in hospitalized patients (up to 18%) [9, 12]. Hyperlipemia frequently occurs secondary to primary disease processes such as enterocolitis/colitis and colic that produce a negative energy balance and affect insulin sensitivity (see section on insulin resistance below). In miniature horses, hyperlipemia develops consequent to a primary disease in the vast majority of cases. In contrast, ponies and donkeys can present with primary hyperlipemia; stress and obesity are predisposing factors. Both stress and obesity induce decreases in insulin sensitivity, which is considered the primary predisposing factor. There appears to be a genetic predisposition in the case of Shetland ponies. Hyperlipidemia (triglyceride concentrations above the reference value limit but without gross lipemia or associated illness) is reported in horses, usually as a result of inadequate food intake. Hyperlipidemia/hypertriglyceridemia due to fasting in horses is reported to range from 100 to 300 mg/dL [7]. In contrast, hyperlipemia, defined as marked increases in serum triglyceride concentrations (e.g., >500 mg/dL) with gross lipemia and clinical illness, is uncommon in large‐breed horses compared to ponies, small breeds, and donkeys [13, 14]. A third hyperlipidemic category of marked hypertriglyceridemia (>500 mg/dL) associated with clinical signs but without visual evidence of plasma lipemia, has been described [7]. The absence of plasma lipemia distinguishes this disorder from hyperlipemia. It is uncertain why this magnitude of hypertriglyceridemia does not cause visible plasma turbidity or opacity given that triglyceride concentrations >300 gm/dL should cause turbidity and concentrations >600 mg/dL should impart a milky appearance. Cases of severe hypertriglyceridemia without visible lipemia were associated with systemic inflammatory response syndrome (SIRS), which is defined as presence of two or more of the following abnormalities: fever or hypothermia, tachycardia, tachypnea or hypocapnia, leukocytosis, leukopenia or greater than 10% immature granulocytes (left shift). All horses had one or more primary disease processes and were off feed, and many were azotemic. Thus, most of the factors contributing to the pathogenesis of hyperlipemia were present. A prevalence of 0.6% over the course of a two‐year period was reported [7]. Metabolic syndrome in horses is primarily a disorder of insulin dysregulation (ID) and is discussed in this context in the next section. Equine metabolic syndrome (EMS) is not a disease but rather a combination of factors that imply increased susceptibility to laminitis. The primary components defining the syndrome include obesity (generalized or localized), hyperinsulinemia, and insulin resistance with a predisposition to the development of laminitis. Another main component of EMS is mild hypertriglyceridemia (including increased VLDL concentrations). Hypertriglyceridemia is more commonly seen in ponies than horses with EMS [15]. In humans, hypertriglyceridemia with decreased HDL cholesterol concentrations is used as a criterion in the diagnosis of metabolic syndrome. Interestingly, horses with EMS have hypertriglyceridemia (VLDL and VLDL triglycerides) with increased HDL cholesterol [4]. A positive correlation between plasma HDL and triglyceride concentrations has also been detected in Shetland ponies [16]. It is postulated that this is a result of the near absence of cholesterol ester transfer protein activity in equine blood. Cholesterol ester transfer protein catalyzes transfer of cholesterol from HDL to VLDL in humans. Thus, in humans, triglyceride carried by VLDLs is exchanged for cholesterol when VLDLs interact with HDL in the blood and, as VLDL concentrations increase, interactions with HDL increase and HDL cholesterol concentrations decrease. Hypertriglyceridemia has been found to be a significant predictor of laminitis in ponies with cut‐off values from 0.64 to 1.06 mmol/L (57–94 mg/dL) [17, 18]. However, triglycerides are correlated with body condition score (BCS); not all obese horses have EMS and cases of EMS in lean horses are reported. Consequently, triglycerides may not be a very specific marker of ID and therefore may not be as valuable in the prediction of laminitis [19]. Obese horses with insulin resistance also have increased blood NEFA concentrations [4]. The NEFA concentration increases with obesity because adipose tissues reach their maximum capacity for fat storage and insulin’s inhibitory effects on hormone‐sensitive lipase are reduced. As a result, the influx of fatty acids into tissues increases, which causes an accumulation of fatty acid metabolites that interfere with insulin receptor signaling, thereby enhancing insulin resistance. Increased uptake of fatty acids by the liver increases the availability of triglyceride for VLDL synthesis, which is part of the mechanism of hypertriglyceridemia associated with insulin resistance. Increased VLDL production has been associated with feed deprivation and hyperlipemia in horses, which are conditions that develop in response to increased mobilization of NEFA from adipose stores. In addition to lipids, the other principal component in energy metabolism in mammals is glucose. Glucose is produced in the liver and, to a lesser extent, in the kidney or is absorbed from dietary sources. Production of glucose is accomplished primarily in the liver through gluconeogenesis and glycogenolysis. In nonruminant species, glucose is manufactured using mainly amino acids. The volatile fatty acid propionate, absorbed in the rumen or hindgut, provides the major building block for de novo glucose synthesis in ruminants. As a hindgut fermenter, the horse can use either amino acids or propionate in gluconeogenesis [20]. Equids rely heavily on gluconeogenesis to maintain blood glucose concentrations due to limited stores of glycogen. During negative energy balance, protein catabolism increases to provide amino acids for gluconeogenesis. There are multiple hormones whose actions result in increases in plasma glucose concentration and one principal hormone that decreases blood glucose. Normoglycemia is the result of the interactions of multiple hormones, but the two primary processes responsible for glucose homeostasis are insulin secretion by pancreatic beta‐cells in response to blood glucose concentration and the response of skeletal muscle and adipose tissue to insulin concentrations. Insulin decreases blood glucose concentrations by promoting cellular uptake, utilization, or storage and by inhibiting hepatic gluconeogenesis (Figure 9.4). Glucagon and catecholamines increase blood glucose by stimulating glycogenolysis; glucagon and corticosteroids stimulate gluconeogenesis; and glucagon and corticosteroids inhibit insulin activity at the receptor and postreceptor level. Catecholamines inhibit insulin secretion, stimulate glucagon release, and inhibit insulin activity at the postreceptor level. The effects of these hormones on fat metabolism were described previously (Figure 9.2). After a meal, insulin is released from pancreatic beta‐cells to facilitate glucose uptake by fat and muscle tissue via the GLUT‐4 transporter. Insulin is not required for glucose uptake by the hepatic GLUT‐2 transporter, but affects hepatic glucose metabolism by stimulating hepatic glycolysis, inhibiting gluconeogenesis, and facilitating glycogen synthesis. When there is a negative energy balance, normoglycemia is maintained in multiple ways. The metabolic rate slows to limit glucose consumption. Glucagon secretion increases and insulin secretion decreases, resulting in gluconeogenesis, glycogenolysis, and peripheral lipolysis. The metabolism shifts from glucose to fatty acids as a primary energy source. Because the liver uses few fatty acids for energy, and the pathway for ketone body formation is not well developed in horses, if the negative energy balance persists, increasing amounts of triglycerides are produced (Figure 9.2) [6]. With time, the hepatocellular triglyceride concentration overwhelms the liver’s capacity for synthesizing and exporting VLDL, resulting in increased triglyceride storage and hepatic lipidosis. Figure 9.4 The effects of hormones on glucose metabolism. Insulin promotes normoglycemia by increasing glucose uptake, utilization, and storage and inhibiting gluconeogenesis. Glucagon, corticosteroids, and epinephrine cause hyperglycemia through various mechanisms including inhibition of insulin activity and stimulation of gluconeogenesis and glycogenolysis. Insulin resistance (IR) is defined as insensitivity to insulin at the cell surface, where glucose entry into the cell is facilitated, or insulin ineffectiveness as a result of the disruption of intracellular glucose metabolism [21]. Insulin dysregulation (ID) comprises states of tissue insulin resistance as well as persistent or intermittent hyperinsulinemia [22]. Hyperinsulinemia was previously thought to occur solely as a response to tissue insulin resistance, but in ponies, transient hyperinsulinemia has also been shown to result from an inappropriate response to ingested carbohydrates in the absence of tissue insulin resistance [23]. Hyperresponsiveness to oral nonstructural carbohydrates without concurrent evidence of insulin resistance is proposed to occur via a functional enteroinsular axis, where alterations in glucose absorption and the secretion of intestinal incretin hormones may account for the difference between healthy and dysregulated ponies. Glucose and lipid metabolism are intricately linked via insulin, glucagon, and other hormones. Abnormalities in lipid metabolism are therefore associated with dysregulation of glucose metabolism. Increased plasma fatty acid concentrations are associated with several insulin‐resistant states in humans through various mechanisms, including inhibition of glucose transport or phosphorylation activity [10
9
Laboratory Assessment of Lipid and Glucose Metabolism
9.1 Lipids
9.1.1 Triglyceride Metabolism
9.1.2 Laboratory Characterization of Lipid Metabolism
9.1.2.1 Lipoproteins
9.1.2.2 Nonesterified Fatty Acids
9.1.2.3 Triglycerides
9.1.2.4 Cholesterol
9.1.3 Equine Hyperlipidemias
9.1.3.1 Hyperlipemia in Ponies, Miniature Horses, and Donkeys
9.1.3.2 Horse Hyperlipidemias
9.1.3.3 Equine Metabolic Syndrome
9.2 Glucose
9.2.1 Glucose Metabolism
9.2.2 Insulin Resistance and Dysregulation
Stay updated, free articles. Join our Telegram channel

Full access? Get Clinical Tree
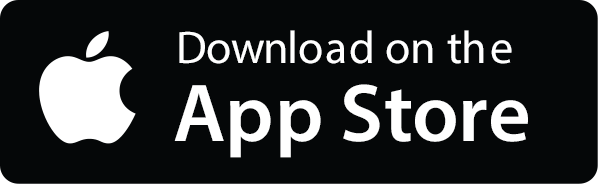
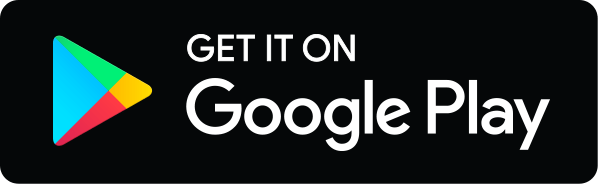