CHAPTER 3 INVERTEBRATES
Invertebrates represent the largest group of animals on Earth, with approximately 1 million species characterized to date. Additional species are being discovered all of the time, and it has been estimated that as many as 30 million invertebrates may reside on planet Earth. The diversity among invertebrates is enormous, with over 30 different phyla and myriads of subsequent taxa. Differences between the groups are so great that the only common feature among them is that, as their name implies, they do not possess a true vertebral column.
COMMON SPECIES KEPT IN CAPTIVITY
Cnidarians
There are approximately 10,000 different species of cnidarians, and the majority of these animals are found in the marine environment. Cnidarians are classified either as polyps or medusae. The polyps include the corals (Figure 3-1), hydrae, and anemones (Figure 3-2). The medusae include the true jellyfish and the box jellyfish. Cnidarians can be found as individuals or colonies, depending on the group. Most are carnivorous, subduing and killing their prey with specialized cells called cnidocytes (see Anatomy and Physiology). Jellyfish are one species with cnidocytes, which also are venomous to humans.
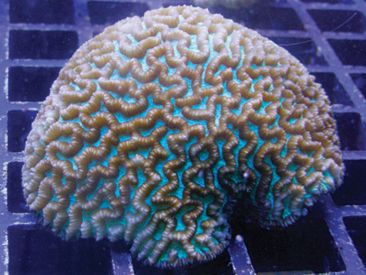
Figure 3-1 Maze brain coral (Platygyra sp.). These stony corals are popular in the aquarium hobby.
(Photo by Trevor Zachariah.)
Gastropods
There are approximately 60,000 different species of gastropods, and these animals are primarily aquatic, although terrestrial forms exist. Among the aquatic gastropods, the major-ity of animals are found in benthic habitats. Gastropods are the most numerous and diverse class within the phylum Mollusca and are the only group to have members that have evolved a terrestrial lifestyle (the Pulmonata). The gastro-pods are primarily represented by the snails (Figure 3-3) and slugs.
Arachnids
There are approximately 70,000 different species of arachnids, and more than 80% of the animals in this group are spiders and mites. The majority of arachnids (Figure 3-4) are terrestrial and carnivorous. The arachnids, like the cnidarians, may possess specialized tools (e.g., venom) to capture and kill prey. In some species (e.g., spiders and scorpions), the venom can also be harmful to humans. Certain species of arachnids, such as the mites and ticks, have evolved to live as parasites on vertebrate hosts.
Myriapods
There are approximately 13,000 different species of myriapods, all of which are terrestrial. Centipedes (≈3000 species) are from the order Chilopoda, and most of these animals are nocturnal predators. These invertebrates possess fangs, which they use to envenomate their prey or potential predators. Millipedes (≈10,000 species) are from the order Diplopoda, and all of these animals are nocturnal detritivores (Figure 3-5). Diplopods possess more legs than any other animal. Unlike the chilopods, diplopods are secretive and prefer to hide from predators rather than challenge them. Diplopods do not pose any real danger to humans.
Insects
The insects represent the largest group of invertebrates, with over 900,000 described species. It is estimated that more than 75% of the animal species on Earth are insects. The greatest diversity among any group of living animals is seen with the insects. Most are terrestrial, but some have also developed the ability to fly. Some species of insects have developed parasitic life cycles, many of which include human involvement. Some species of insects can pose a danger to animals and humans through their defensive mechanisms (e.g., bees). Numerous species are of economic importance to humans as pests.
Echinoderms
There are approximately 6,000 different species of echinoderms. Many of these animals are common to the commercial aquarium trade, including the sea stars, brittle stars, sea urchins, sand dollars, sea cucumbers, and sea lilies (Figure 3-6). All of these species are marine and benthic. The echinoderms share a five-part radial body plan, also known as pentamerous symmetry, and have the ability to voluntarily move connective tissue, known as catch connective tissue (see Anatomy and Physiology).
ANATOMY AND PHYSIOLOGY
Cnidarians
Whether in the form of a polyp or medusa, all cnidarians have a basic body plan that is radially symmetric. There are two tissue layers: the epidermis, which lines the outside of the animal, and the gastrodermis, which lines the inside of the animal. These layers are separated by a nonliving layer of elastic, gelatinous material known as the mesoglea, which provides structure and buoyancy without metabolic cost. Tentacles ring the mouth, the single opening to the digestive system (Figure 3-7). All surface tissues perform direct gas exchange.
In the tentacles, and sometimes in the living tissue layers of the body, cnidocytes can be found. Cnidocytes contain a specialized, secreted organelle called a cnida. When stimulated by chemical or tactile cues, the cnidae use osmotic pressure to propel a hollow tube with great force out of the cell toward prey or predators. The tube can be used to physically subdue or inject venom into prey. The venom used in cnidae can be quite potent and may also be used for defense. Each cnida can be used only once, after which the cnidocyte must secrete a new one.
Arachnids
The body plan of the arachnids has two major components: the prosoma and the opisthosoma (Figure 3-8). The prosoma is comprised of a fused head and thorax that is covered dorsally by a carapace and ventrally by a sternal plate. The pleurae join the two and are flexible, which allows them to move relative to each other. The opisthosoma, or abdomen, contains the majority of the internal organs. In spiders, the prosoma and opisthosoma are joined by a narrow bridge called the pedicel. In scorpions, the two body segments are fused, and the opisthosoma is segmented and divided into two parts: the anterior mesosoma and the posterior metasoma, or tail. The metasoma is comprised of five to seven segments and, at its terminus, has a telson with a stinger.
Most of the appendages originate on the prosoma. The most cranial pair of appendages are called the chelicerae. The chelicerae help to grasp and tear prey and bear the fangs in those species that have them (Figure 3-9). After the chelicerae, the next pair of appendages are called the pedipalps. The pedipalps come in a variety of forms and can serve different functions. In spiders, the pedipalps are similar to the legs but lack the metatarsal segment, whereas in scorpions, they terminate with pincers. In both groups of arachnids, the pedipalps are used to grasp prey and assist with copulation. The remaining prosomal appendages are the walking legs, of which there are four pairs. Each leg has seven segments, including (proximal to distal) the coxa, trochanter, femur, patella, tibia, metatarsus, and tarsus. The distal end of each leg bears a tarsal claw and, in many species, scopulae, dense tufts of hair that allow the arachnid to climb. The last set of spider appendages originate on the posterior end of the opisthosoma and include the three pairs of spinnerets. The spinnerets are variously modified to meet the needs of each species. Any of the appendages of arachnids may be autotomized, or voluntarily detached, and regenerated after several molts.
The prosoma contains the anterior portion of the digestive tract, including the diverticula of the midgut, which extend through the pedicel; the sucking stomach; and the mouth, which is just posterior and ventral to the chelicerae. A pair of venom glands are also found in the prosoma of spiders. These glands are under voluntary control and are connected directly to the fangs. The prosoma is highly muscular and contains pseudoskeletal, cartilage-like structures called endosternites, which serve to anchor the muscles. The prosomal muscles maintain hemolymph pressure by contracting and relaxing the carapace and sternal plate, which in turn allows for extension of the appendages.
Sensory perception in arachnids is achieved through a number of specialized organs. Spiders are covered in different types of hairs that allow for sensing of tactile, seismic, and chemical stimuli. Scorpions possess paired pectines, which are paired comb-like organs used to detect chemical and seismic stimuli. The pectines are located caudal to the last pair of legs.1 Both spiders and scorpions possess eyes, although the number (up to 12) varies. The visual acuity of arachnids can also vary among species. It is generally believed that giant spiders have poor vision, whereas jumping spiders (family Salticidae) can make well-developed images.
In many of the New World giant spiders, hairs are used as a defense mechanism. Urticating hairs, located on the opisthosoma, are small, barbed structures that can be discharged to ward off a threat. Giant spiders use their caudal pair of legs to rapidly kick these hairs into the air. When the urticating hairs settle on the body surfaces of a potential predator, they cause severe irritation. Individual spiders may have more than 1 million urticating hairs on their abdomen, at a density of approximately 10,000 hairs per square millimeter.2 The urticating hairs are replaced after each successive molt.
The life span of some scorpion species may reach 25 years.3 The most common species kept in captivity, the African emperor scorpion (Pandinus imperator), has a life span of 3 to 8 years.1 Male giant spiders live only a short time after reaching sexual maturity and have a life span of 6 to 18 months, on average.4 Female giant spiders, however, live significantly longer, with anecdotal reports of individuals surpassing 30 years of age. With proper captive care, it would not be uncommon for female giant spiders to live in excess of 20 years.
Myriapods
The myriapod body plan is elongated and composed of numerous segments (Figure 3-10). Each segment, except for the head and anal segments, bears either one (centipedes) or two (millipedes) pairs of legs, although the first few segments in millipedes bear only one pair of legs. Despite their names, centipedes and millipedes do not have 100 and 1000 legs, respectively. In actuality, these animals generally have 40-60 and 150-200 legs, respectively. Most of the body mass of the myriapod consists of the trunk. Millipedes are cylindrical in shape, with a hard, calcified exoskeleton. Centipedes are dorsoventrally flattened, with no waxy outer cuticle layer. Centipedes are built for speed when catching prey, whereas millipedes are slow and built for powerful digging.
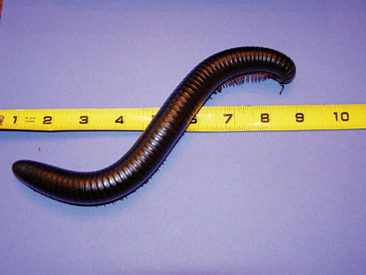
Figure 3-10 Giant African black millipede (Archispirostreptus gigas). Note the large size and long, tubular body form.
(Photo by Trevor Zachariah.)
Besides the legs, myriapods have other appendages that are important for their survival. A pair of antennae are located on the head and the jaws, providing sensory input. Centipedes also possess a pair of forcipules on the first body segment, which are essentially venomous fangs used for acquiring prey. On the anal segment of centipedes is a pair of anal legs. These structures can have various functions, including tactile, defense, and aggression, depending on the species.3
The digestive system in all myriapods is long and tubular. Centipedes have a pharynx and esophagus that represent the majority of the gut length, whereas the millipede gut consists primarily of midgut. Millipedes have salivary glands associated with the oral cavity, whereas centipedes have a variety of glands associated with the pharynx and esophagus.3 The paired Malpighian tubules serve as the primary excretory organs. Millipedes have a layer of tissue that surrounds the midgut that has both energy storage (e.g., glycogen) and detoxification properties.3
The heart is a tubular organ that lies dorsally along the length of the trunk. Ostia act to move blood in and out of the heart. The dorsal pericardial sinus is formed by a horizontal membrane, as is the ventral perineural sinus. The perivisceral sinus is located in the middle of these other sinuses.3 Myriapods have an open circulatory system.
In myriapods, gas exchange occurs via tubular trachea that delivers oxygen directly to the tissues. The trachae open to the environment through spiracles in the exoskeleton. In most species, the spiracles cannot be closed, which greatly hampers water conservation and results in the necessity of maintaining a humid, moist environment. The spiracles are located ventrally in millipedes and laterally in centipedes.
Sensory structures in myriapods consist mainly of the eyespots and antennae. The eyespots consist of a varying number of ommatidia (individual sensory units), depending on the species. In most myriapod taxa, the ommatidia are not clustered in densities high enough to form a true compound eye, such as is found in insects.3 Myriapods are not believed to be capable of forming images. Instead, it has been suggested that these animals are limited to sensing light and movement.3 The antennae are able to sense both tactile and chemical stimuli.
Myriapods are gonochoric and practice internal fertilization. In general, female centipedes are protective of their egg masses until the young hatch and disperse.3 Centipedes generally have a life span of 4 to 6 years; millipedes live for 1 to 10 years.3
Insects
The insect body is divided into three body sections: the head, thorax, and abdomen. The head bears a single pair of dorsal antennae, a variable number of ocelli, a single pair of compound eyes, and the ventral mouthparts. The mouthparts are modified to reflect the feeding strategy of the taxa. For example, sucking mouthparts are found on moths and butterflies (order Lepidoptera); piercing and sucking mouthparts on aphids, cicadas, and assassin bugs (order Hemiptera); cutting and sponging mouthparts on flies (order Diptera); and chewing and sucking mouthparts on bees and wasps (order Hymenoptera).3
The abdomen of insects is segmented and relatively devoid of appendages. A terminal pair of cerci are usually present as are, in some species, external genitalia.3 The abdomen is often the largest of the three basic body segments and houses the majority of the viscera.
The digestive system of insects is divided into three regions: the foregut, midgut, and hindgut. The foregut consists of the mouth, pharynx, esophagus, crop, and proventriculus. The midgut is the primary site for food digestion. Attached to the anterior end of the midgut are two to six ceca.3 The hindgut is comprised of the intestine, rectum, and anus. A variable number of Malpighian tubules (e.g., 2 to 250, depending on the taxon) are attached to the anterior end of the hindgut.3 The hindgut serves as the major excretory center in the insect body and resorbs most of the water from the digestive system.
Oxygen is delivered directly to the tissues by tubular trachae, in a fashion that is similar to that of myriapods and some arachnids. Spiracles are found on the thorax and abdomen, but not on the head.3 Unlike the spiracles of myriapods, those of most insects can be closed to prevent loss of moisture or water.
In insects, the perception of multiple types of sensory stimuli is performed by sensilla, or hair-like receptors. These structures are found all over the body, although the majority are found on the appendages.3 Chemical, tactile, temperature, and humidity receptors are found on the antennae and tarsi. The abdominal cerci contain tactile and seismic receptors. The ocelli are used to detect changes in light intensity and aid in orientation.3 Visual stimuli are detected by the compound eye, and each of the ommatidia has its own lens. Thus, contrary to popular misconception, the insect brain, similar to the brain of vertebrates, integrates the information from each ommatidium to form a mosaic image.3 Many insects also have tympanic organs for the detection of sound.
Insects are gonochoric and practice internal fertilization. There are three types of development among the insects. Hemimetabolous development involves juveniles called nymphs, which are dissimilar from adults, are aquatic, and grow and molt until reaching a final molt into the adult form. Paurometabolous development involves juveniles that are also called nymphs; however, these are similar to adults and grow and molt into the adult form. Holometabolous development (e.g., metamorphosis) involves juveniles called larvae, which grow and molt until forming a pupa, from which the adult form emerges. Holometabolous development is a successful life strategy, as approximately 80% of insects (e.g., approximately 740,000 species) utilize it.3
Crustaceans
The crustaceans, like the insects, represent a diverse group of animals, with variable body forms. One of the most commonly recognized forms, the order Decapoda (e.g., crabs, lobsters, crayfish, and shrimps), will be described here (Figure 3-11).
The anterior section of the trunk bears eight pairs of appendages. The anterior three appendages, or maxillipeds, assist with feeding, while the posterior five, pereopods, are used for walking. There are seven segments of the pereopods, including the coxae, basis, ischium, merus, carpus, propodus, and dactyl. In many species (e.g., crabs, lobsters, and crayfish), the first pereopod is modified into an enlarged cheliped or pincer that is used for defense, food acquisition, and courtship. Limb autotomy is a common occurrence in decapods and most often occurs as a result of combat with conspecifics or in defense against predators. The limbs regenerate with later molts.
The posterior of the trunk is comprised of a variable number of segments, depending on the taxon. The terminal end of the trunk bears a telson and paired uropods, and together, these form a tail fan. The tail fan helps to create the backward thrust of shrimp, crayfish, and lobsters. The five pairs of appendages arising from the posterior trunk are the pleopods. The pleopods are biramous and may be modified to serve a variety of functions, including swimming, burrowing, creating ventilating or feeding currents, brooding eggs, gas exchange, and copulating.3 In crabs, the posterior abdomen and pleopods are reduced and found ventral to the carapace.
Decapods have a compact, ostiate heart. This organ is located dorsally under the carapace. The heart is connected to a system of arteries (seven main arteries leave the heart), capillaries, and venous sinuses.3 Hemolymph is transferred through the circulatory system to the gills for oxygenation. The gills, of which they may have up to 24 pairs, are also responsible for excreting nitrogenous wastes.3 Ion balance in crustaceans is maintained primarily by the antennal glands (e.g., green glands), which are located in the cranial aspect of the head. Urine is created and stored in a bladder that opens near the base of the ventral pair of antennae.
Sensory perception in crustaceans is accomplished by the eyes, antennae, and appendages. The stalked eyes are some-what mobile, and some crustaceans may be able to detect color.3 Setae (hair-like receptors) are capable of detecting chemical stimuli and are primarily found on the antennae and appendages. Aesthetascs, or collections of setae, are located on the dorsal pair of antennae. Statocysts are found at the base of the dorsal antennae and assist with orientation. In some crustaceans, statocysts may also be found on other appendages.3
Echinoderms
The body plan of echinoderms follows pentamerous symmetry. Though a type of radial symmetry, the echinoderms are not closely related to the cnidarians. Pentamerous symmetry is based on a central axis around which five body regions aggregate. The body surfaces are described as oral and aboral (Figure 3-12). Sea cucumbers (class Holothuroidea) maintain pentamerous symmetry in an elongated body form, with the ends of the animals being described as oral and aboral.
Besides their recognizable body forms, another unique feature of echinoderms is the water-vascular system (WVS). The basic anatomy of this system starts with a madreporite, an eccentric, porous opening that is found on the aboral surface of sea stars and urchins and on the oral surface of brittle stars. The madreporite opens into a stone canal, which then leads to a circumoral ring canal. Leading perpendicularly from the ring canal are the radial canals and blind sacs known as polian vesicles. The radial canals reach into the arms of sea and brittle stars and along the inside to the aboral surface in sea urchins and cucumbers.5 Multiple lateral canals direct water from the radial canals to ampullae, each of which is attached to a tube foot. The ampullae are used in a manner that is similar to the bulb of a turkey baster, increasing pressure to extend the tube feet and decreasing pressure to contract them. The function of the polian vesicles has not been fully determined, but it may serve in aiding maintenance of fluid pressures within the WVS.
The madreporite and stone canal function to maintain fluid pressure within the WVS. The stone canal is supported by calcareous ossicles and is lined with cilia, which beat to create water flow. The fluid within the WVS is essentially seawater, with increased cellular, protein, and potassium concentrations.3,5
Another unique feature of echinoderm anatomy is catch connective tissue. This tissue is mutable, which allows these animals to vary the rigidity of their bodies at will. The stiffness or softness of the dermis is due to the extracellular matrix, in which nerves have been found to terminate.3 Research has found that calcium ion concentrations vary proportionally to the rigidity of the tissues.3
HUSBANDRY
Because of the enormity of invertebrate species, it is not possible to cover all of the husbandry needs of these animals in a single chapter. Instead, we will give a short review of the husbandry needs of the most common species; for a more detailed review, see Lewbart.6 In considering the captive care of an invertebrate, it is best to have some background knowledge of the particular species’ natural history. Even though there is relatively little known about the needs of the myriad species of invertebrates, it is best to try to mimic the natural environment and diet as much as possible. Many times, it is best to maintain a simplistic approach, as more advanced attempts at maintaining these animals may have negative results.
Cnidarians
As with most aquatic species, water quality is the most important factor associated with the successful management of cnidarians in captivity. Stoskopf7 recommends the following guidelines: ammonia levels less than 0.1 ppm, nitrite levels less than 1 ppm, nitrate levels less than 10 ppm, dissolved organic matter levels between 0.5 and 3.0 ppm, undetectable phosphate levels, calcium levels between 400 and 450 ppm for corals, pH between 8.2 and 8.4, alkalinity between 3.2 and 4.5 mEq/L, and a specific gravity (as a surrogate measure for salinity) around 1.025 to 1.027. Trace elements are another important consideration, and these animals depend on the water to provide these essential nutrients. Unfortunately, little is known regarding the specific needs of these animals, so attempts should be made to mimic natural levels of trace elements based on the natural body of water from which these animals are derived.
Water motion is particularly important for cnidarian species (Figure 3-13). Cnidarians are generally classified as being either mobile or sessile. Water motion is essential for both groups because it facilitates nutrient gathering and oxygenation. For mobile species, water motion is important also for transporting the organisms.7 Because sessile species cannot move away from their wastes or accumulated organics, water motion serves to disperse potential toxicants. Cnidarians exposed to excessive water motion can be injured. The force of the water movement can push cnidarians into the walls of the aquarium or other fixed objects within the aquarium.7 Even sessile cnidarians can be injured by excessive water movement, as the fixed organisms are battered by the substrate. Water motion can be provided by way of a power head, airstone, or wave maker. Water motion is generally measured in gallons of water moved per hour. Determining the most appropriate flow rate depends on aquarium size and volume. The authors generally look at the movement of the cnidarians in the aquarium to deter-mine what is best. If the cnidarians appear to be moving too quickly or are being battered against the aquarium or substrate, then the flow rate should be reduced.
Due to their diversity, the cnidarians as a group are well adapted to a variety of temperature ranges; however, individual species may have a relatively narrow tolerance for temperature changes.7 As a general guideline, tropical anemones and corals should be kept between 20° and 31° C (68°-87.8° F), with an optimal temperature being around 24° C (75.2° F).7 For temperate species, a temperature range of 20° to 24° C (68°-75.2° F) is considered more appropriate.7 Thermostatically controlled aquarium heaters are the best method to provide an appropriate temperature range within an enclosure. In larger aquaria, multiple heaters may be required to establish an appropriate temperature range. Thermometers should be placed in different areas of the aquarium to monitor temperature.
Many cnidarians derive a significant amount of their nutrients, sometimes up to 90%, from symbiotic algae (e.g., zoochlorellae or zooxanthellae) that are embedded in their tissues.3 The loss of these organisms, due to improper lighting or water quality conditions, can be devastating for a cnidarian. Full spectral lighting that mimics the sun is considered ideal. The light should provide ultraviolet and visible light. Stoskopf7 recommends photosynthetically active radiation (e.g., 400-700nm) with more flux density between 400 and 550nm than between 650 and 700nm. The lighting should be maintained close to the water surface (<6 cm) to maximize its value.
Gastropods
Terrestrial species are easily kept in terraria of different sizes; however, vertical enclosures are preferable for arboreal species and horizontal cages for terrestrial species. A moist substrate, such as damp sphagnum moss, should be used to provide moisture and will help to maintain a relatively high humidity level in the enclosure. The substrate should be changed periodically to reduce the likelihood of opportunistic pathogens. Gastropods left on “dirty” substrate are more prone to dermatitis and shell lesions. The frequency of substrate changes will depend on the number of animals, size of the vivarium, and frequency and types of food offered. Cork bark can be placed in the enclosure to provide hiding places for the animals. Because of gastropods’ ability to climb perpendicular surfaces, a tight-fitting, solid lid is recommended (e.g., glass top). This will also help to maintain the high humidity level. Although humidity is important, it is just as important that the air in the enclosure does not become stagnant. Stagnant air can lead to the overgrowth of certain pathogens that can affect gastropods. Gastropods are most comfortable and active with low-intensity lighting. Temperature requirements will vary depending on species; for some species, temperatures exceeding 23° C (73.4° F) should be avoided.8 Again, knowledge of where an animal originates can help a veterinarian determine the most appropriate temperature range.
Arachnids
The size of an enclosure for a giant spider does not need to be expansive, as most species are not large in size or extremely active. Also, they should not be kept communally, because cannibalism is possible. A 35.6 cm × 25.4 cm × 25.4 cm (14″ × 10″ × 10″) glass container can be used as a basic enclosure and provides ample space for all but the adults of the largest species of giant spiders (e.g., Theraphosa blondi, Lasiodora parahybana, Pseudotheraphosa apophysis). In addition to glass tanks, plastic storage containers, large plastic or glass bottles, and glass or plastic fish bowls can be used. A distinction can be made between arboreal and terrestrial species of giant spiders. With terrestrial species, care must be taken to keep the height of the enclosure to a minimum. All spiders are capable of climbing vertical glass and plastic surfaces and can suffer life-threatening injuries from short falls (see Common Disease Presentations). Arboreal species, however, should be provided an enclosure with a high vertical-to-horizontal ratio (e.g., 3 : 1) (Figure 3-14).
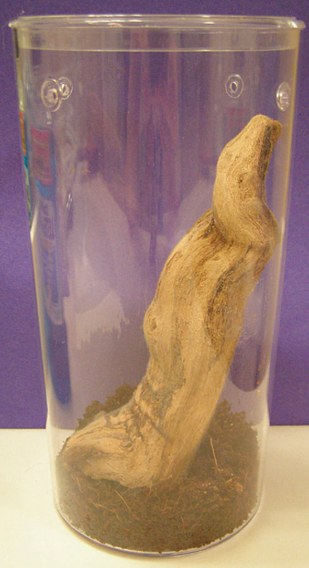
Figure 3-14 Basic arboreal spider habitat. Note the large vertical-to-horizontal ratio.
(Photo by Trevor Zachariah.)
For giant spiders, humidity must be closely regulated, even more so than temperature. Excessive humidity can lead to potentially harmful fungal and pest infestations within an enclosure. Insufficient humidity can lead to desiccation and dehydration. There are several ways to regulate humidity: change (1) the moisture in the substrate (e.g., higher water-to-substrate ratio), (2) the size of the water dish, or (3) the amount of ventilation of the enclosure. To increase humidity, the substrate moisture and the water dish size should be increased, while the amount of ventilation is decreased. To reduce the humidity within an enclosure, the opposite should be done. Lightly misting the enclosure with lukewarm water (24.4°-26.7° C, 76°–80° F) can also be done to increase humidity. For large vivaria, live plants can be used to increase humidity. For giant spiders, a general rule is that tropical species require 70% to 100% humidity, desert species 40% to 60% humidity, and temperate species 50% to 70% humidity.9,10
Giant spiders can vary in their daily periods of activity, and no broad generalizations can be made for this group. For most species of giant spider it is not known whether the animal is diurnal, nocturnal, or crepuscular; however, it is known that all species appear to be extremely averse to bright light.10 For this reason, giant spiders should not be kept under a direct light source. Also, as stated earlier, direct light sources (e.g., incandescent bulbs) can desiccate an enclosure and its resident. Direct sunlight has the same effect and should be avoided. Low or ambient lighting works well for most species, and a 10- to 12-hour photoperiod is recommended.
There are a variety of substrates available, either commercially or naturally, for use in giant spider enclosures. Organic materials are usually preferred. Examples include topsoil, potting soil, peat moss, sphagnum mass, bark or mulch (except pine or cedar), ground coconut hull, and leaf litter. All of these substrates are good at enhancing humidity levels in an enclosure, but they are also prone to supporting fungal and pest infestations.9–11 To reduce the likelihood of opportunistic infestations, the material should be dry or only slightly damp.
Nonorganic materials are also popular substrates for giant spiders. Examples include vermiculite, aquarium gravel, artificial turf, and sand. Compared to organic substrates, these materials are not as efficient at maintaining humidity. Sand and aquarium gravel are not recommended, as they can abrade the exoskeleton of a giant spider and provide unstable footing. Many of these nonorganic substrates can be mixed in various ratios to provide an appropriate environment. For burrowing species, the substrate should be packed well and be coarse enough that the risk of burrow collapse is minimized. For giant spiders, Marshall9 recommends a substrate depth of 8 to 20 cm for terrestrial species and 2.5 cm for arboreal species. Substrates that are strongly contraindicated include cat litter (too dusty), carpet (potential injury from snagging), newspaper (pest and fungal problems), and wood chips (pest and fungal problems, pine and cedar toxicity).10,11
Live plants are not essential components to making a vivarium. Although they add a decorative touch, they are not needed to properly maintain most species. Plastic plants can be used and are easier than live plants to disinfect. Live plants used in a giant spider vivarium must be relatively small in size and able to tolerate low light levels. Cacti, or plants with spines or sharp edges, should be avoided because they can be injurious to the spider.9 Marshall11 recommends snake plants (Sansevieria spp.), bromeliads (Cryptanthus spp.), peperomias (Peperomia spp.), and climbing plants (e.g., philodendrons (Philodendron spp.) or pothos (Epipremnum spp.) for giant spider enclosures.
Crickets are the most common prey item offered to giant spiders.11 Although crickets do not provide a balanced diet for vertebrates (e.g., inadequate calcium), they appear adequate for invertebrates. However, a varied diet comprised of different invertebrate and vertebrate prey species is still considered more appropriate.9 Cockroaches, grasshoppers, mealworms, superworms, kingworms, wax moth larvae, earthworms, and neonatal mice may be used to vary the diet.8,12 Small lizards, crayfish, goldfish, and raw meat have also been given to giant spiders but are less common and generally not recommended.10 Captive-reared prey is preferred over wild-caught prey. Wild-caught prey can serve as a source of toxin exposure or infectious disease.13 All prey items should be offered a high-quality diet before being offered to the spider. If the prey items are not promptly fed to the spider, they will be of limited nutritional value.
Many giant spider species are stimulated by movement of their prey, so it is usually best to feed live prey items when appropriate. Exceptions to this include vertebrate prey that may injure the spiders. When live prey items are offered, they should be monitored carefully to ensure that they do not harm the spider. This is especially true during the molting process, when the exoskeleton is more fragile; a giant spider should never be fed during a molt. Prey of appropriate size should be offered. A good rule of thumb to follow for spiders is that the prey should be no larger that the spider’s opisthosoma,10 or one third to one fourth the length of its body.12 If any prey is left uneaten after 24 hours, it should be removed. The quantity of food offered to a giant spider should be based on the dietary habits of the particular species, its life stage, and the environmental conditions. For example, adult giant spiders should be fed on a weekly9,12 or, at a minimum, monthly basis,10 whereas spiderlings should be fed every 2 days.12
Water should always be made available to giant spiders, as they will actively drink water. Open water dishes should be provided, though the depth should be limited to prevent drowning. Some sources recommend placing a soaked sponge or cotton in the water dish; however, this is not considered hygienic and can serve as a source of opportunistic bacterial infections for the spider.10,13 For giant spiders, the water dish must be positioned so that the animal can tilt its mouthparts down to the surface (Figure 3-15).8,10 The water dish should be cleaned and refilled regularly, or as often as it is soiled.
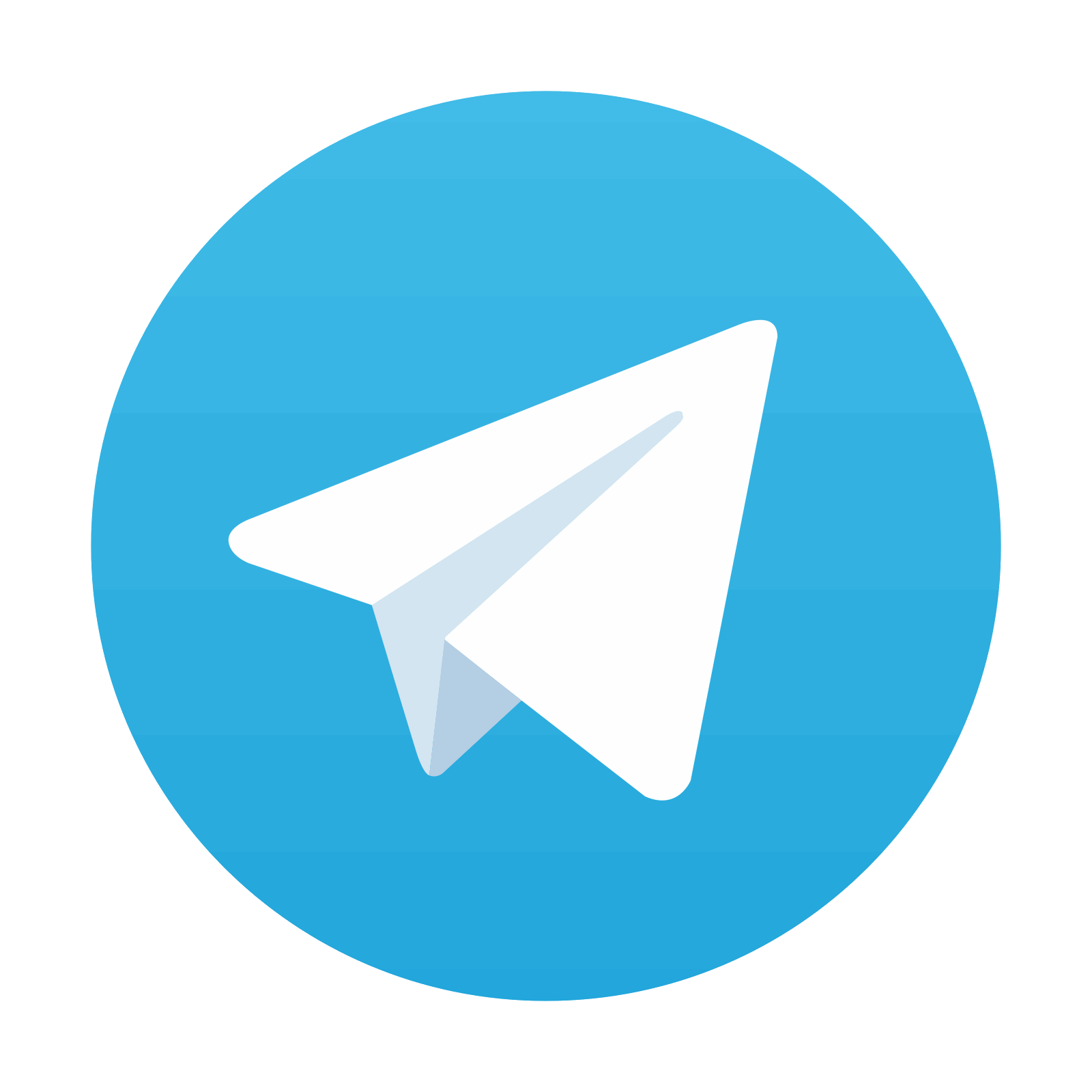
Stay updated, free articles. Join our Telegram channel

Full access? Get Clinical Tree
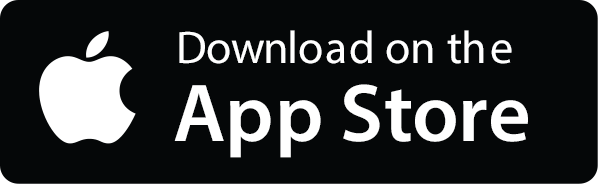
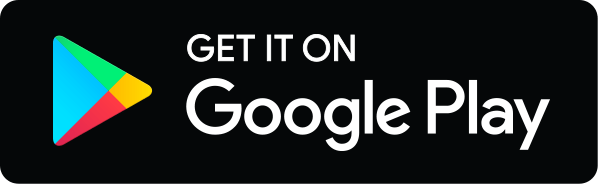