Chapter 30 Butch Cargile1 and Dan Tracy2 1 Progressive Dairy Solutions, Twin Falls, Idaho, USA 2 Multimin USA, Auburn, Kentucky, USA Successful reproductive management of the modern dairy cow results from the implementation of best management practices. In the absence of widespread infectious disease, the largest effector of reproductive performance is prior and current nutritional status. Nutritional status of the dairy cow involves a complex interaction between macronutrients and micronutrients and herd-level management. In this chapter we discuss several of these interactions and their effects on reproductive performance. From a veterinary perspective, the primary tenet of animal nutrition is preventative medicine. Cows are programmed to succeed or fail, reproductively, based on the degree of metabolic stress experienced during the transition period from the nonlactating late-gestation stage to the lactating postpartum stage. The goal of any commercial dairy’s reproductive program is the establishment and maintenance of a subsequent pregnancy as soon as physiologically possible following parturition. This pregnancy leads to another parturition and new lactation cycle. Unfortunately, parturition is a biological event associated with considerable risk. The risks associated with several metabolic–immunological disorders are correlated with the transition period of a dairy cow, in addition to the possibility of dystocia which itself has a complex interaction with each of the metabolic disorders. Given the array of possible complications and negative outcomes associated with parturition, profitability is the only impetus strong enough to cause dairy farmers to expose their cattle to this degree of risk. Figure 30.1 displays the relative periods of the lactation cycle with regard to profitability. Over a cow’s productive life, the more cumulative time she spends under the area of the curve associated with profitability, the more profitable she is for the operation. The only way to gain access to this phase of the lactation cycle is through additional parturitions. Figure 30.1 Periods of the lactation cycle with regard to profitability. The transition period is widely accepted as the 3 weeks immediately before parturition through 3 weeks immediately following parturition. Disorders associated with this period of the reproductive cycle of the dairy cow include, but are not limited to, periparturient paresis (milk fever), retained fetal membranes (retained placenta), metritis, hepatic lipidosis (fatty liver syndrome), ketosis, and displaced abomasum. These disorders exert both direct and indirect effects on reproductive performance via follicular memory, immune function, and endometrial status. Table 30.1 displays incidence rates of the different metabolic disorders. Table 30.1 Incidence of the different metabolic disorders. Placental detachment is an immunologically driven event. In a study by Kimura et al.1 neutrophils isolated from the blood of cows with retained placenta had significantly lower function before and after parturition, extending up to 2 weeks after calving. Further evaluation in this experiment revealed lower plasma interleukin (IL)-8 concentrations in cows ultimately developing retained placenta. IL-8 functions as a chemoattractant for neutrophils and induces phagocytosis. Addition of anti-IL-8 antibodies to cotyledon preparations led to a 41% reduction in neutrophil chemotaxis in vitro. Metritis is the commonly accepted vernacular for an infection contained within the uterus of the postparturient cow. These are typically Gram-negative anaerobic bacterial infections caused by invasion (growth) of microbes from the vagina or delivered to the uterus iatrogenically. Immunosuppression during the periparturient period has been shown to predispose the cow to metritis. This immune suppression has been described as starting 1–2 weeks prior to parturition.2–5 Cows experiencing dystocia have been identified as being 2.58 and 4.32 times more likely to develop metritis than cows classified as having a normal parturition.6,7 One method being studied in an attempt to ameliorate this prepartum immunosuppression is alteration of the ratio of omega-6 (n-6) to omega-3 (n-3) fatty acids in the duodenum.8 It appears that by increasing the n-6 : n-3 ratio in multiparous cows before calving, functional properties of mononuclear cells may be improved. It has been suggested that a dietary ratio of n-6 to n-3 of 15 : 1 or more could be beneficial to reproductive performance during the lactation to follow. Negative energy balance (NEB) is defined here as insufficient consumption of the calories necessary to meet metabolic requirements. This is the rule rather than the exception for late prepartum and early postpartum dairy cows. NEB begins in the prepartum period and progresses as dry matter intake (DMI) declines sharply several days before calving. This sharp decline in DMI is now thought to be the result, rather than cause, of excessive lipolysis.9 Ketosis is clinically classified as elevated plasma level of the ketone β-hydroxybutyrate (BHB). This occurs when the body’s tissues, primarily muscular and neural, utilize less ketone than is being produced by hepatocytes. Increased production of BHB is a result of excessive mobilization of nonesterified fatty acids (NEFA). Ketosis, by itself, is a mammalian survival mechanism. However, animals of economic importance should not be approaching the survival threshold as this is well outside the zone of profitability and efficient production. Cows first testing positive for subclinical ketosis (BHB concentration 1.2–2.9 mmol/L) from 3 to 7 days in milk were 0.7 times as likely to conceive to first service compared with cows first testing positive at 8 days in milk or later,10 intimating effect(s) based on temporal occurrence as well as incidence. Hepatocyte uptake of NEFA depends on the circulating NEFA plasma concentration. Once internalized, NEFA can be utilized, through esterification, back into triglyceride (TG) for internal storage. TG can be repackaged into very low density lipoproteins (VLDL) and exported from the liver for use by the mammary gland and other tissues. Internalized NEFA can also be oxidized partially, which results in ketone production, or oxidized completely for adenosine triphosphate (ATP) production. This process is termed β-oxidation. Intrahepatic repackaging of TG involves the inclusion of TG into VLDL. The bovine liver has a limited capacity to produce VLDL. Therefore, during periods of excessive lipolysis, the cellular machinery of the bovine hepatocyte becomes overwhelmed with TG and is physically impaired by the amount of TG being stored intracellularly. The upper end of the spectrum of this condition is termed hepatic lipidosis and typically occurs when TG constitutes greater than 5% of liver tissue on a wet basis. The B vitamins niacin and choline are effectively used to alter the adipose–circulatory–hepatic axis. Niacin effectively limits lipolysis through binding and stimulation of the G-protein-coupled receptor GPR109A in humans.11 Similar effects have been seen in dairy cattle fed 12 g/day per head of rumen-protected niacin.12 While effective at reducing lipolysis, this method of control leads to a significant reduction in energy-corrected milk production and is unacceptable in commercial settings. Lipolysis and the subsequent metabolism of NEFA by the bovine liver is an evolutionary adaptation to conserve lactation through provision of VLDL as an alternate energy substrate and provide the substrate necessary for milk fat production in colostrum and early lactation milk. When this process is limited, production is limited. Although potentially appealing from a reproductive standpoint, ultimately this control strategy is financially infeasible from a commercial perspective. Supplementation with rumen-protected choline, on the other hand, addresses the rate-limiting step involving the inclusion of TG into VLDL, thus facilitating a net increase in hepatic energy production (translation) without increasing hepatic charge. Hepatic charge has been theorized to be a satiety initiator in the bovine.9 Choline is integral in the production of phosphatidylcholine and critical for lipid transport and lipoprotein synthesis.13 Feeding of rumen-protected choline in a study by Lima et al.14 led to a reduction in mastitis approaching statistical significance. The link between postpartum mastitis and early reproductive performance is discussed later in this chapter. Both choline and the amino acid methionine are methyl donors and can spare each other in this process (Figure 30.2). Figure 30.2 Methylation pathways (DNA, proteins, lipids). Another effective nutritional strategy addressing clinical and subclinical ketosis is the use of the only ionophore currently approved for use in lactating dairy cattle, monensin sodium. In Canada and other countries, controlled-release indwelling capsules that reside in the rumen and provide continuous release of monensin are approved for use. This delivery system is not currently approved in the United States but feeding of monensin is approved. Monensin has been shown in a meta-analysis to reduce the incidence of clinical ketosis and severity of subclinical ketosis.15 Monensin exerts its effects by increasing ruminal propionic acid production as a result of bacterial selection. This effect seems counter to the hepatic oxidation theory proposed by Allen16 as the production of propionate is involved in satiety signaling in ruminants. However, this can be reconciled because the additional production of propionate is spread over a longer time-frame than the spikes from the rapid fermentation of starch following meals.17 In its embryonic state in dairy cattle immunology, and infancy in human immunology, immune modulation by β-glucans is addressed crudely from a nutritional standpoint presently. The source and, more importantly, form of the specific β-glucan appears to have significant impact on its functionality with regard to gene expression within target immune system cells. Extensive studies are needed to elucidate the exact proinflammatory and pro-resolving effects of β-glucans and their subsequent effect(s) on reproduction. Milk urea nitrogen (MUN) is closely associated with blood urea nitrogen (BUN). BUN is an indirect measure of “excess” dietary protein or, more specifically, ammonia. Within the normal range of commercial ration formulation, BUN concentration increases as the ratio of soluble protein to nonfiber carbohydrates increases. Absolute amounts of the different protein fractions must be matched with their corresponding carbohydrate fractions when considering their respective ruminal degradation rates. When matched, efficient use of dietary protein and carbohydrate results in efficient production of microbial protein with minimal ammonia needing to be detoxified by inclusion into urea by the liver. The biochemical process of converting ammonia to urea exerts an energy cost on the cow. A study by Rajala-Schultz et al.18 showed that cows with MUN levels between 10.0 and 12.7 mg/dL were 1.4 times more likely to be confirmed pregnant than cows with MUN levels above 15.4 mg/dL. In this same study, cows with MUN levels below 10.0 mg/dL were 2.4 times more likely to be confirmed pregnant than cows with MUN levels above 15.4 mg/dL. Studies suggest multifactorial negative fertility effects as a result of elevated BUN, including altered oocyte development,19,20 embryonic maturation, and intrauterine environment.21,22 The use of metabolizable protein and amino acid balancing nutritional models has allowed crude protein and average MUN levels to be reduced. Rumen-protected forms of lysine and methionine are commercially available and appear to be economically viable. Use of these ingredients allows balancing of rations at considerably lower crude protein levels while still maintaining predicted production levels on a metabolizable protein basis since lysine and methionine are the first and second limiting amino acids in normal dairy rations. The functional micro-unit of reproduction from the female perspective is the ovum. Follicular memory is a carry-over effect that delays the influence of metabolic status on reproductive performance by as much as 60 days.23–25 As the investigation and understanding of the influence that individual fatty acids exert on reproductive performance increases, NEB becomes a more complex proposition as it relates to fertility in the dairy cow. The negative effects of oleic acid, and a combination of oleic, palmitic and stearic fatty acids, have been studied with regard to their direct effect(s) on granulosa cells and cumulus–oocyte complexes26 and theca cells.27 In vivo, these negatively correlated effects are secondary to elevated circulating plasma levels of these individual and/or combinations of NEFA. Fatty acid composition of adipose tissue is influenced greatly by diet and degree of ruminal biohydrogenation of unsaturated fatty acids. Since NEFA result from the liberation of TG from adipose tissue, nutritional composition of absorbed fatty acids has a direct influence on the relative and absolute concentrations of NEFA.
Interaction of Nutrition and Reproduction in the Dairy Cow
Introduction
Reproductive goals
Transition period
Disorder
Incidence (%)
Milk fever
5–10
Retained placenta
5–10
Clinical ketosis
5–10
Metritis
8 to >40
Clinical endometritis (4–6 weeks after calving)
15–20
Retained placenta, metritis and endometritis
Ketosis and hepatic lipidosis
β-Glucans
Milk urea nitrogen
NEFA concentration and oocyte formation
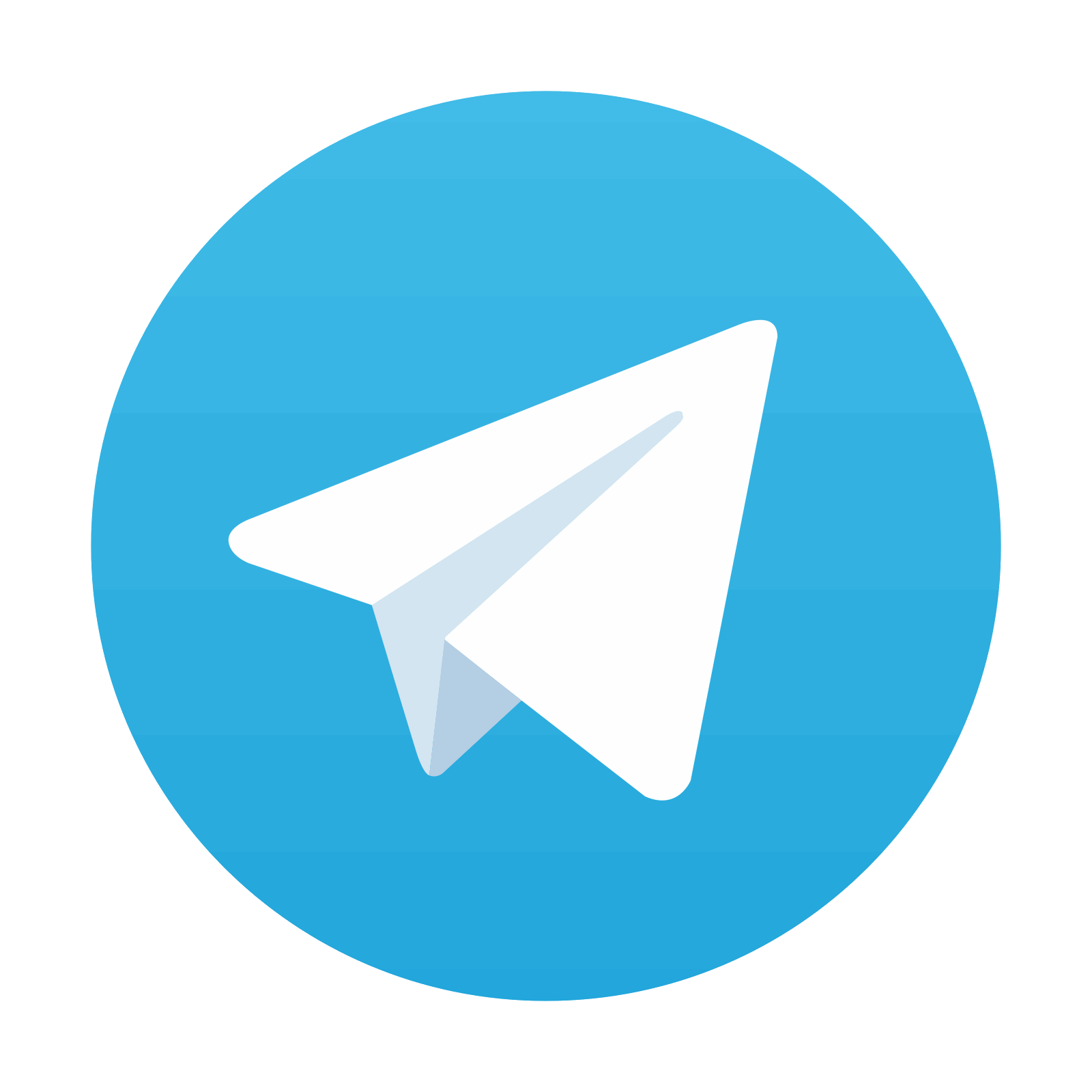
Stay updated, free articles. Join our Telegram channel

Full access? Get Clinical Tree
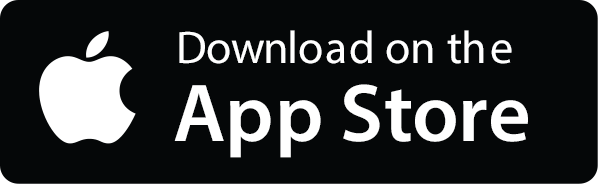
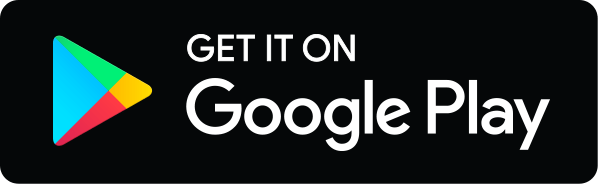