20 Infectious Hematopoietic Necrosis Virus 1Bro Biotech, Glenelg, Maryland, USA; 2Phycal Inc., Highland Heights, USA; Aquaculture is a major industry with an average annual growth rate of approximately 7% and globally is the fastest growing sector in animal-based food production. However, the biggest threat to sustainable growth of fish aquaculture is viral disease. One of the most important of these fish viral diseases is infectious haematopoietic necrosis (IHN), which is a disease of salmonid fish caused by the infectious haematopoietic necrosis virus (IHNV) (Walker and Winton, 2010). IHN has widespread geographic distribution and seriously impacts both farmed and native salmonid stocks. IHN was first described in the 1950s in sockeye (Oncorhynchus nerka) and Chinook (O. tshawytscha) salmon, and then in rainbow trout (O. mykiss) in 1967 (Amend and Smith, 1975). Acute clinical infections are most prevalent in fry and juvenile fish. IHNV is one of the three aquatic Rhabdo-viruses listed as reportable by the World Organization for Animal Health (OIE) and is considered to be a constraint to the expansion of salmonid aquaculture in the USA (Bootland and Leong, 2011). The major impact of IHNV is on farms that rear young rainbow trout or salmon where cumulative mortality can reach 90–100% (Bergmann et al., 2003; Purcell et al., 2012a). In addition to direct losses due to mortality, the disease has a negative impact on the breeding of endangered salmon stocks, causes restrictions on the movement of IHNV-infected fish or survivors, and necessitates the destruction of infected stocks as a containment strategy. It was estimated that IHN caused a minimum of US$3 million loss to the Idaho (USA) trout industry alone (Congleton, 1988). This is due to direct mortality, decreased fish production levels and deformities that occur in the survivors. In Canada, between 2001 and 2003, IHN epizootics caused CAN$250 million loss to the Atlantic salmon industry (MacKinnon et al., 2008). As recently as May 2012, an IHN outbreak occurred in a salmon farm off Bainbridge Island, Washington, resulting in the removal of all fish and a significant loss to the USA salmon industry (http://tinyurl.com/c2oalmr). IHNV infects a broad range of salmonids including rainbow trout/steelhead (O. mykiss), brook trout (Salvelinus fontinalis), lake trout (S. namaycush), cutthroat trout (O. clarkii), brown trout (Salmo trutta), Atlantic salmon (Salmo salar), chinook salmon (O. tshawytscha), sockeye/kokanee salmon (O. nerka), chum salmon (O. keta), cherry salmon/masou salmon/Yamame trout (O. masou), biwa salmon (O. masou rhoduris), amago salmon (O. rhodurus), coho salmon (O. kisutch), Japanese charr (S. leucomaenis), Arctic charr (S. alpinus), Arctic grayling (Thymallus arcticus) and mountain whitefish (Prosopium williamsoni). The susceptibility to IHNV can vary greatly from species to species and between strains within a species. IHNV has also been isolated from European eel (Anguilla anguilla) (Bergmann et al., 2003). Through laboratory studies, the virus was also shown to infect other related salmonids, such as gilt-head bream (Sparus aurata), turbot (Scophthalmus maximus), sea bass (Dicentrarchus labrax), northern pike (Esox lucius) and pile surfperch (Rhacochilus vacca). Research is ongoing to determine if other hosts could be carriers of this virus to help explain viral distribution and pinpoint the potential cause of epizootics. For example, a recent study examined the ability of the Pacific lamprey to act as a host and found that it did not develop clinical signs or accumulate virus higher than the amount originally injected (Kurath et al., 2012). The first sign of an acute IHN outbreak is a sudden increase in fish mortality. However, some fish may die without showing any of the clinical signs associated with IHN. Clinical signs include distended abdomen, haemorrhaging, anaemia, pale gills, exophthalmia (pop-eye) and skin darkening (Fig. 20.1). This can be accompanied by a pale liver, spleen and kidney due to the anaemia. Ascites can accumulate and the stomach can be filled with watery fluid (red to yellow) rather than food. This can be accompanied by petechial haemorrhages in the visceral mesenteries, swim bladder, adipose tissue, peritoneum, meninges and pericardium (Bootland and Leong, 2011). The disease can progress to a lethal necrosis of the haematopoietic tissues of the kidney and spleen. In acute viral epizootic disease, a generalized viraemia with associated necrosis can be observed in all tissues – this is thought to be caused by the virus passing from the gills to the circulatory system, which then distributes the virus to all organs and tissues. Death probably is due to renal failure caused by electrolyte imbalance (Rodríguez Saint-Jean et al., 2012). Fig. 20.1. Gross pathological symptoms of IHN disease in rainbow trout. Panel A shows IHNV-infected fish displaying darkened body coloration compared to lighter naïve fish. Panel B shows severe exophthalmia or popeye symptom, which is a classic sign of IHN. Panel C shows the pale gills and liver that are clinical signs of the anaemia caused by IHN. Histological manifestations of IHN include destruction of the haematopoietic tissue. The stained section of a normal kidney is shown in comparison to the kidney of an IHN-infected animal in Fig. 20.2 (panels A and B, respectively). The total destruction of the haematopoietic tissue is an obvious clinical sign of this disease. IHNV can be transmitted both horizontally through fish-to-fish contact, and vertically with virus associated with the egg. However, the disease is transmitted mostly by fish to fish through direct contact or through water in which virus was shed by infected fish through faeces, urine, sexual fluids or external mucus. Vertical transmission through eggs is also possible, although its presence inside the egg itself is not fully demonstrated, and somewhat controversial. The risk of egg-associated transmission of the virus can be significantly reduced through the use of iodophor disinfectants and virus-free water supplies. The aetiologic agent of IHN is a virus. The IHNV virions are bullet shaped, measuring approximately 110 nm in length and 70 nm in diameter (Fig. 20.3A). IHNV is the type species in the genus Novirhabdovirus within the family Rhabdoviridae. Other fish Novihabdovirus species are viral haemorrhagic septicaemia virus (VHSV), hirame rhabdovirus (HIRRV), snakehead rhabdovirus (SHRV), eel virus B12 (EV-B12) and eel virus C26 (EV-C26). The genome of IHNV contains a single-stranded, negative-sense RNA of approximately 11.1 kb (Morzunov et al., 1995; Schutze et al., 1995). There are six genes in the viral genome that are, in the 3′ to 5′ order: nucleocapsid (N), polymerase-associated phosphoprotein (P), matrix (M), surface glycoprotein (G), nonvirion protein (NV) and virus polymerase (L) (Schutze et al., 1995). These are shown in the diagram in Fig. 20.3A. The presence of the NV protein is a distinguishing feature of the Novirhabdovirus genus within the Rhabdovirus family (Kurath et al., 1997). There is a 60-nt leader sequence on the 3′-end and a 101-nt trailer sequence on the 5′-end of the genome (Fig. 20.3B). The non-coding sequences at either end of the viral genome are complementary to each other and are likely to form a panhandled structure for priming the initiation of RNA synthesis similar to other members of the family Rhabdoviridae (Banerjee and Barik, 1992). In IHNV-infected cell lines, all six virally encoded genes have been identified. Among these genes, the N protein gene (N gene) is expressed 2–3 hours post-infection and is the most abundant protein produced during an IHNV infection owing to the presence at the 3′-end of the genome (Kurath and Leong, 1985; Leong and Kurath, 2010). Therefore, the N gene is a good target for early detection of IHNV infection. Compared with the N gene, the P and M genes are expressed later, at 6–7 hours post-infection, followed by the G (at 9–10 hours) and L (at 15 hours) genes, respectively. The N protein is present in the mature virion and remains tightly associated with the viral genome (Gilmore and Leong, 1988). During the late phase of virus infection, the N gene is highly expressed, while its corresponding protein has been shown to play a crucial role in regulating the balance between viral transcription and replication. This N protein contains 413 amino acids with a molecular mass of 40.5–44 kDa (Fig. 20.3A). Both the N- and C-terminals of the N protein contain highly hydrophilic residues, whereas the middle third of the protein contains hydrophobic residues. During the late phase of IHNV infection, the N protein binds to the nascent RNA transcript and prevents recognition of the mRNA transcription signals by the viral polymerase protein. This inhibition results in formation of a full-length plus-strand template, which is used for synthesis of the progeny minus-strand genome (Biacchesi et al., 2000a, 2000b). Fig. 20.2. Histological manifestations of IHN disease in rainbow trout. Panel A is a section of normal kidney. Panel B is a section of kidney from IHN-infected fish. Note total destruction of the haematopoietic tissue. Panel C is anterior kidney organ imprint stained with DiffQuik. Note the ‘foamy’ macrophage in the centre of the slide and necrotic debris. Fig. 20.3. (A) A schematic representation of the structure and protein components of IHNV. The IHNV virus contains five structural proteins: L (150–225.2 kDa), G (67–70 kDa), N (40.5–44 kDa), P or M1 (22.5–27 kDa) and M or M2 (17.5–21.8 kDa). The SDS gel is schematically represented on the left with arrows pointing to the location of the viral proteins. (B) A schematic representation of the genome organization of IHNV. IHNV genome contains a negative-sense, single-stranded RNA virus of approximately 11 kb. The location of different genes in the genome in the 3′ to 5′ order are N, P, M, G, NV and L. There are untranslated region on both 5′- and 3′-end of the genome. The P protein, previously called the M1 protein, is a highly basic (pI = 8.4) and phosphorylated protein with unknown function. The basic nature of the IHNV P protein is in sharp contrast to the homologous phosphoprotein of mammalian rhabdovirus, rabies virus and vesicular stomatitis virus (VSV). The IHNV P protein contains 231 amino acids with a molecular mass of 25.6 kDa. Sequence analysis of the P genes of different IHNV strains revealed that the P gene might also contain a small ORF encoding a highly basic, arginine-rich protein with 42 amino acids with a molecular mass of 4.8 kDa and an estimated pI of 10.1. However, this putative protein has not been identified in IHNV-infected cells, although a similar small protein encoded by an overlapping ORF has been reported in other rhabdoviruses such as vesicular stomatitis virus and rabies virus. Like the P protein, the M protein is also a highly basic protein (pI = 10.08) that contains a number of basic amino acids at the N-terminal end of the protein that are conserved among the homologous matrix proteins of other fish rhabdoviruses, including VHSV and HIRRV. The M protein has been shown to induce apoptosis using in vitro cell culture assays when transfected with plasmid DNA containing the M gene (Chiou et al., 2000). However, the molecular pathways leading to apoptosis with IHNV-infected fish in vivo have not been determined. The G gene in the IHNV genome encodes a G protein that forms a part of the structural protein in the mature virion. It is a membrane-associated protein that forms spike-like projections on the surface of the mature virion (Fig. 20.3A) and antibodies targeted to the G protein neutralize viral infectivity (McAllister and Wagner, 1975; Engelking and Leong, 1989). The predicted amino acid sequence of the IHNV G gene contains 508 residues with a molecular mass of 67–70 kDa (Fig. 20.3A). Both the N- and C-terminal regions contain hydrophobic domains. The N-terminal 20 amino acid residues represent a signal sequence, whereas the C-terminal hydrophobic region is likely to represent a transmembrane domain. A comparison of the predicted amino acid sequence of the IHNV G protein with that of other rhabdoviruses identified a number of conserved cysteine and proline residues between IHNV, VSV and rabies. Unlike the N- and C-terminal regions, the middle of the G-protein (also called mid-G; amino acid residues 365–430) is very hydro-philic and highly variable among different IHNV isolates, which makes the mid-G section of the G-protein a good region for phylogenetic analysis (Troyer et al., 2000; Garver et al., 2003; Kurath et al., 2003). Epidemiological studies of North American IHNV isolates using mid-G protein sequences revealed three major genetic subgroups, designated as U, M, and L, based on the prevalence of the isolates in the upper (U), middle (M), and lower (L) parts of the IHNV geographic range (Troyer et al., 2000; Garver et al., 2003; Kurath et al., 2003). The U genogroup is the most prevalent in Alaska and British Columbia. However, it is also found in fish in coastal Washington, and the Columbia River basin, including Washington, Oregon and Idaho. The M genogroup occurs in the Columbia and Snake River basins, and recently in Washington coastal watersheds. In contrast, the L genogroup occurs in California and on the southern coast of Oregon. Although IHNV isolates belonging to each genogroup are capable of infecting multiple host species, there is a general trend in host-specificity among IHNV isolates in North America. The IHNV isolates from the U genogroup are highly virulent to sockeye salmon (O. nerka) and M genogroups causes low mortality; in rainbow trout (O. mykiss), M genogroups are highly virulent and U genogroups causes low mortality (LaPatra et al., 1990). The NV (‘nonvirion’) gene that encodes a protein of 111 amino acids is located between the G and L genes and is highly conserved among some of the fish rhabdoviruses, IHNV, VHSV and HIRRV. The NV gene is present only among the rhabdoviruses infecting fish; this distinction led to the creation of a new taxonomic genus, Novirhabdovirus (van Regenmortel et al., 2000). The NV protein does not show significant similarity to other protein sequences in the GenBank database. The function of this protein was unknown until recently, when it was shown that a recombinant IHNV knockout with no NV gene was severely impaired for growth in cell culture and had reduced pathogenicity in rainbow trout (Thoulouze et al., 2004; Biacchesi, 2011). These two studies clearly showed that the NV protein is needed for optimal growth of the virus. However, for another Novirhabdovirus species, snakehead rhabdovirus (SHRV), the NV protein was found to be non-essential for virus replication (Alonso et al., 2004). It has been speculated that the NV protein is perhaps essential for cold-water fish Novirhabdoviruses, such as IHNV, but not for warm-water fish Novirhabdoviruses, such as SHRV, reflecting temperature adaptation of IHNV versus SHRV to infect different hosts. It would be interesting to determine the effect of replacing IHNV NV gene with SHRV NV gene and vice versa to evaluate the complementation of the NV protein on virus phenotype, pathogenicity and host adaptation using a reverse genetics approach (Biacchesi, 2011). Further insight into the role of the NV protein in IHNV pathogenicity has been elucidated by Choi et al. (2011). These authors demonstrated that the NV protein supports the growth of IHNV through inhibition of the induction of host IFN systems. The 111 amino acid-containing NV protein contains a nuclear localization signal (32EGDL35), which plays an important role in the inhibitory activity of NV. The L gene encodes a protein of 1986 amino acids with a predicted molecular mass of approximately 225 kDa, and shows similarity to the RNA-dependent RNA polymerase (RdRp) genes of other rhabdoviruses. The IHNV RdRp contains six conserved domains similar to other unsegmented, negative-strand RNA viruses (Poch et al., 1990). Phylogenetic analysis based on the L gene revealed that Novirhabdoviruses are more related to plant rhabdoviruses than to rhabdoviruses that infect terrestrial animals (Bourhy et al., 2005). After waterborne exposure, IHNV enters a fish through the gills, skin, fin bases, mouth and oesophagus/cardiac/stomach regions and then the virus replicates in epidermal cells (Mulcahy et al., 1982; Yamamoto et al., 1990; Helmick et al., 1995; Arkush et al., 2004; Foott et al., 2006; Harmache et al., 2006). In rainbow trout, upon viral entry, transient replication of the virus occurs in fin bases, gills and skin before spreading to internal organs, although some researchers reported that skin did not serve as a portal for entry of the virus (Brudeseth et al., 2002). Harmache et al. (2006) used a recombinant infectious IHNV expressing the Renilla luciferase gene and bioluminescent imaging to show that the fin bases of rainbow trout serve as the portal of entry for IHNV. Upon entry, the virus replicates at the fin base but not in other tissues (spleen, kidney, heart and liver) as early as 8 hours post-infection (Harmache et al., 2006). In addition, fish that survived acute infection had localized bioluminescence in the fins, indicating that IHNV permissive cells are localized in the fins (Harmache et al., 2006). Therefore, it is possible that in asymptomatic carrier fish, the fins may serve as a reservoir for the virus. Foott et al. (2006) reported that in Chinook salmon the gills and the skin support replication of the virus for almost 6 weeks in the absence of detectable virus in the internal organs. Drolet et al. (1995) proposed that the virus infection progresses through two parallel routes, from the mouth into the gastrointestinal tract and from the gills to the circulatory system, with systemic viraemia resulting from both routes. An ultrastructural study confirmed that the attachment and internalization of IHNV occurs via the mucosal epithelial cells of the oesophagus/cardiac/stomach region (Helmick et al., 1995). Irrespective of the route of entry, typically within 24 hours post-infection a low titre of the virus is detected in gills, skin and intestine. Within 2–4 days, the virus spreads to the kidney and subsequently spreads to other organs. In young fish, the haematopoietic tissues of the kidney and spleen are severely affected (Fig. 20.2). Within 5–10 days post-exposure, young fish show signs of IHNV infection and mortalities as high as 80–100% can occur. Fish that survive IHNV infection may display spinal curvature deformities such as scoliosis and lordosis (LaPatra et al., 2001). The manifestation of IHN disease depends on the size and age of the fish, the species and strain and the environmental conditions, including water temperature (LaPatra et al
3Clear Springs Foods, Inc., Buhl, USA
20.1 Introduction
20.2 Host Range and Clinical Signs
20.3 Virus Transmission
20.4 Genome Organization and Gene Expression
20.5 Viral Pathogenesis
Stay updated, free articles. Join our Telegram channel

Full access? Get Clinical Tree
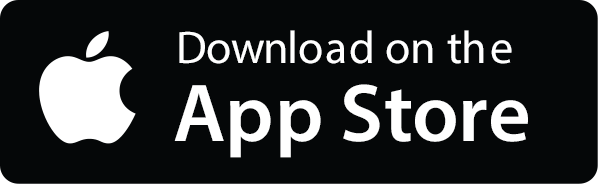
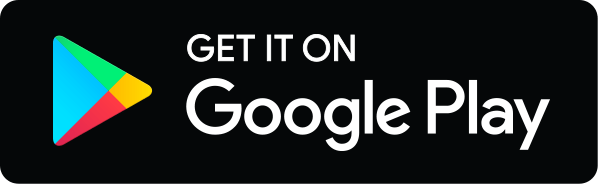