Chapter 60 Thomas Passler Departments of Clinical Sciences and Pathobiology, College of Veterinary Medicine, Auburn University, Auburn, Alabama, USA Bovine viral diarrhea virus (BVDV) is a pathogen of cattle and other artiodactyls with substantial impact on the health and well-being of affected livestock. Originally described by two North American research groups in 1946,1,2 infections with BVDV are still cause for enormous financial losses to the cattle industries worldwide. While the two earliest reports on BVDV described a disease that was mostly of severe acute nature, less severe subacute cases were also observed.1,2 In the decades following their original descriptions, BVDV infections in immunocompetent animals were reported to be mainly subclinical, and it was estimated that 70–90% of acutely infected animals did not display clinical signs.3 The perception of BVDV as a mainly apathogenic bovine virus changed in the early to mid 1990s with the description of two new BVDV-associated syndromes, hemorrhagic syndrome and severe acute BVD that were responsible for severe clinical signs with mortality rates of up to 100%.4,5 Genetic sequencing of BVDV isolates from severe outbreaks led to the description of a second genotype of BVDV (BVDV 2) based on its genetic dissimilarity of greater than 30% from classic BVDV strains.5,6 Subsequent retrospective genetic typing revealed that BVDV 2 had been present in Ontario as early as 1981 without causing severe disease symptoms.4 Variation of the severity and observed clinical signs among BVDV-associated disease outbreaks emphasizes the influence of the tremendous genetic variability of BVDV isolates. BVDV is an RNA virus and is the prototypic member of the genus Pestivirus within the family Flaviviridae. Currently, the genus Pestivirus contains four recognized species: BVDV 1 and 2, border disease virus, and classical swine fever virus (CSFV).6 Additional genetically distinct genotypes such as Pronghorn pestivirus, Giraffe pestivirus, strain V60 (Reindeer-1), and HoBi strain have been described, but have not yet been officially classified.7–11 The lack of strict host specificity and antigenic relatedness of pestiviruses has caused difficulties with the traditional method of classification based on the host from which a strain was isolated, and more accurate taxonomic information is now based on monoclonal antibody binding assays and phylogenetic analysis of genomic sequences.12–16 Although sequence variations are located throughout the entire genome, the 5′ untranslated region (UTR) is most commonly used for the differentiation of pestiviral species.12,13,15,17,18 Pestiviruses are enveloped viruses with a single-stranded RNA genome that is approximately 12.5 kb in length and contains a single large open reading frame flanked by UTRs at the 5′ and 3′ termini.19 During replication of BVDV in the host cell cytoplasm, mutation and variation occurs readily with an approximate mutation frequency of 10–4 base substitution per base site.20 At this high mutation frequency, at least one point mutation occurs per replication cycle of the viral RNA.21 Frequent mutation is the consequence of error-prone viral RNA polymerases and results in variation of each progeny virus from the infecting parent virus.20 Although millions of varying progeny virions are produced daily during the peak of infection, only copies with a selective advantage and without deleterious mutations will progress in the course of an infection.20,22 Consequently, a swarm of mutant virions exists in the host during a BVDV infection.20 The virions of this mutant swarm are similar in base sequence and as adapted to the host’s organism and are referred to as a quasispecies.20 The existence of BVDV as a quasispecies with frequent mutation events offers a significant advantage to viral survivability, as viral mutants are readily available that are capable of adapting to adverse conditions such as the host’s immunity. With respect to virulence, adaptation to either lower or higher virulence may benefit viral survivability. While low virulence and host survival, as are common for host-adapted viruses, enable prolonged shedding, viral mutations that increase rates of replication and virulence allow for a burst of viral shedding.20,23 BVDV isolates can be classified as noncytopathic or cytopathic, and this classification is based on the effects of an isolate on cultured cells. Cytopathic isolates cause cytoplasmic vacuolation and death of cultured cells, but infections with noncytopathic isolates are inapparent.24,25 The categorization into biotype is not associated with the virulence of a BVDV isolate, and mild or severe disease can be observed with either biotype. The noncytopathic biotypes are more prevalent in nature and 60–90% of isolates from specimens at diagnostic laboratories are noncytopathic.26,27 Only noncytopathic BVDV strains can induce persistent infections, and these noncytopathic strains are believed to be the most common source for cytopathic BVDV strains following homologous or heterologous recombination in the NS2–3 genomic region.20 A third biotype of BVDV, the lymphocytopathic biotype, has been proposed, and refers to BVDV isolates that have cytopathic effects only on cultured lymphoid but not epithelial cells.28 Lymphocytopathic isolates are BVDV 2 strains that were associated with severe clinical disease.28 Following the initial descriptions in North America, BVDV has been detected in cattle populations worldwide. The prevalence of seropositive animals depends largely on the management of cattle, including type of husbandry, use of vaccination protocols, addition of new herdmates, and presence of persistently infected (PI) animals.29,30 Cattle herds that do not use vaccination programs or only use killed vaccines and do not have PI animals have fewer numbers of seropositive cattle than herds containing PI cattle.29 In North America, serosurveys demonstrated seropositive rates between 40 and 90%.31,32 The individual seroprevalence rate among unvaccinated dairy heifers in Canada for BVDV 1 and BVDV 2 was 28.4% and 8.9%, respectively, and the herd-level prevalence was 53.4% for BVDV 1 and 19.7% for BVDV 2.33 In feedlot cattle, seroconversion to respiratory pathogens including Pasteurella haemolytica, Mycoplasma spp., and BVDV following arrival occurred in 40% of cattle,34,35 but varied between 0 and 100% of cattle in 11 pens.34 Similar rates of seroprevalence have been detected in cattle herds worldwide, but the observed range varied widely among countries (13–90%) and was largely influenced by the examined population, potentially reflecting global differences in cattle management.36–52 In contrast to seroprevalence rates, the prevalence of PI cattle is considerably lower and is generally believed to be less than 1% of all cattle. Persistently infected cattle may be found in clusters within groups of cattle, elevating the prevalence within populations.53 The prevalence of PI calves arriving at feedlots has been demonstrated to be between 0.1 and 0.3%, and this rate is similar to the reported rate of 0.17% for beef cow-calf operations in the United States.34,54–56 Pestiviral infections have been identified in various species of the mammalian order Artiodactyla, and lack of host specificity may play an important role in the pestiviral survival strategy.57 Among pestiviruses, BVDV has been the species most intensely studied as an infectious agent of heterologous hosts. Evidence of BVDV infection exists in over 50 species of artiodactyls in the families Antilocapridae, Bovidae, Camelidae, Cervidae, Giraffidae, Suidae, and Tragulidae.58 The implications of heterologous pestivirus infections are twofold. First, heterologous BVDV infections may hamper the success of control strategies, as are in progress for BVDV in cattle and CSFV in swine. Second, heterologous BVDV infections may threaten the health and well-being of other mammalian hosts, such as free-ranging and captive wildlife. The role of heterologous infections in the epidemiology of BVDV is incompletely understood, but transmission and maintenance of BVDV within three host clusters –domestic small ruminants and swine, free-ranging and captive wildlife, and camelids – has been postulated.59 The close phylogenetic relatedness of small ruminants to cattle and resulting greater possibility of permissive infection may promote a unique role of sheep and goats in the ecology of BVDV. Sheep and goats often have closer contact with cattle compared with other artiodactyls, especially in certain management conditions such as subsistence agriculture, alpine farming, or hobby farming. While one report did not identify a positive correlation between BVDV seropositivity of sheep flocks and presence of cattle,60 other studies have reported significantly greater seroprevalence rates in sheep on farms with cattle, especially where communal alpine pasturing of sheep, goats, and cattle was practiced.61 Several serological surveys have demonstrated the occurrence of BVDV in small ruminants; however, cross-reactivity of pestiviral antibodies warrants caution when evaluating the results of such surveys. In recent reports on pestiviral seroprevalence in sheep, cross-neutralization assays identified highest reactivity against BVDV 1 rather than border disease virus.60,61 When antibodies against pestivirus are detected in small ruminants, the distinction of pestiviral species may hold critical information on epidemiology, prevention, and control.62 Infections with BVDV in domestic small ruminants result in clinical signs of border disease. Postnatal infections commonly cause mild clinical signs, including pyrexia and leukopenia.63 Experimental inoculation of juvenile sheep resulted in anorexia, tachycardia, pyrexia, and lung lesions especially associated with the pulmonary vasculature.64 Infections with BVDV in pregnant small ruminants may result in uteroplacental pathology, pregnancy loss by fetal resorption or abortion, and birth of mummified or stillborn fetuses.65 As in cattle, congenital BVDV infection in sheep and goats can result in damage to the central nervous system, and neuropathogenicity may be more severe with cytopathic biotypes.66 Although fetal death and nonviability of lambs are common sequelae of transplacental BVDV infections in sheep, reports of viable PI offspring exist.67,68 In contrast to cattle and sheep, viable PI goat kids appear to be rare and reproductive failure is the more common outcome of BVDV infection in goats.65,69 The close antigenic relationship between ruminant pestiviruses and CSFV has important implications for diagnostic testing and control of CSFV. Currently, examination of blood samples for CSFV antibodies relies on a combination of enzyme-linked immunosorbent assay (ELISA) and virus neutralization techniques, and considerable efforts are necessary in establishing a CSFV-specific diagnosis.70 During the CSFV outbreak in the Netherlands in 1997/1998, 26.5% of CSFV ELISA-positive samples were caused by the presence of antibodies to ruminant pestiviruses.70 Only a limited number of BVDV serosurveys have been performed in domestic swine, and reported seroprevalence rates are relatively low compared with other domestic livestock. Risk factors associated with seropositivity of domestic swine include the presence of cattle on the same farm, high density of small ruminants near swine populations, vaccination with BVDV-contaminated vaccines, and age of tested swine.71–73 Postnatal BVDV infections in nonpregnant swine rarely result in clinical signs.74,75 Experimental infection of 8-week-old pigs with either BVDV 1 or BVDV 2 did not result in clinical signs or increases in body temperature in any group, despite the use of a BVDV 2 isolate that was demonstrated to be virulent for cattle.75 In contrast, BVDV infection of pregnant gilts resulted in reproductive disease, including intrauterine infection, pregnancy loss, and reduction in litter size.76 While transplacental BVDV infection in pigs appears to less common than in ruminants, the birth of PI piglets has been reported.77 BVDV infections were identified through detection of antibodies as early as 1975 in Old World camelids (OWC) and 1983 in New World camelids (NWC).78,79 However, most seroepidemiologic and experimental infection studies suggested that BVDV may cause infections without presenting a serious risk to NWC. Reports of BVDV isolation and identification of persistent infections in alpacas have prompted increased interest in BVDV infections in camelids, and BVDV is now considered an emerging pathogen of NWC.80 The first description of a PI alpaca was made in Canada following natural exposure of a pregnant alpaca to a chronically ill cria.81 From the PI cria, a BVDV 1b strain was isolated from buffy coat cells on different occasions before euthanasia of this animal. Several cases of PI alpacas have since been reported in North America and the United Kingdom.80,82,83 PI alpacas may survive for several months but are affected by low birthweight, failure to thrive, inappetence, lethargy, chronic diarrhea, and chronic recurrent infections especially of the respiratory tract. In OWC, reproductive disease including abortion, stillbirth, weak calves, early neonatal death, and neonatal hemorrhagic disease have also been reported.84 Noncytopathic BVDV has also been isolated from tissues of camels in which histopathologic changes were consistent with a BVDV infection.85 From NWC in Chile, both BVDV 1 and BVDV 2 have been isolated.86 In contrast, in reports from North America and the United Kingdom in which the subgenotype of the infecting isolate was determined, all isolates belonged to BVDV 1b.80–82 A study analyzed 46 BVDV isolates from over 12 000 North American alpacas and classified all isolates as noncytopathic strains of subgenotype 1b with 99% or more nucleotide homology in the 5′-UTR.83 Two explanations for this apparent predominance of BVDV 1b in NWC have been proposed: first, exposures of alpacas to BVDV are rare and spread of the existing BVDV 1b strain is by extensive movement of a few PI animals; or, second, only unique 1b subgenotypes are able to establish transplacental infections in alpacas.83 Movement of alpacas (including dams with cria by foot) between farms, mainly for breeding purposes, is common practice and have been described in reports of reproductive disease and birth of PI offspring, highlighting the importance of sound biosecurity practices.81,82,87 Although sources of BVDV infections in free-ranging wildlife are unknown, a likely source is contact with cattle. This is supported by the absence of antibodies in deer that were without contact with cattle for over 50 years.88 Significantly greater seroprevalence rates were detected in white-tailed deer on ranches where cattle were present compared with ranches without cattle.89 In contrast, an association between BVDV seroprevalence and cattle density was not detected in another study.90 Similar findings have been described in a recent report from Minnesota where a greater percentage of deer were seropositive in a region with a lower cattle density.91 The authors of this report concluded that cattle use and management (i.e., dairy or beef) may have an important impact on interspecific BVDV transmission, as there is likely less wildlife contact with housed dairy cattle compared with pastured beef cattle.91 Various factors likely influence the transmission of BVDV between cattle and wildlife as has been described for other pathogens such as bovine tuberculosis.92 With presence of suitable environmental and management factors, transmission of BVDV from cattle to deer is possible as has been demonstrated in a cohabitation experiment.93 In this study, BVDV was efficiently transmitted from PI cattle to white-tailed deer and resulted in seroconversion in all adult deer and birth of PI fawns from four of seven pregnant does.93 Maintenance of BVDV within a cervid population without presence of cattle was suggested by the presence of antibodies in over 60% of caribou that had not been in contact with domestic ruminants for over 25 years.94 The presence of endemic BVDV infections as indicated by high seroprevalence rates was also suggested in reindeer in Norway and US cervid populations.95–97 This is supported by identification of high seroprevalence rates in eland populations of Zimbabwe, in which a PI and a virus-isolation-positive eland were identified.98 Only a limited number of studies have surveyed free-ranging wildlife populations for the presence of BVDV or BVDV antigen. Anderson and Rowe98 utilized an antigen-capture ELISA to detect BVDV in a subset of 303 seronegative animals during a serosurvey in Zimbabwe and detected two BVDV-positive eland. In Germany, cytopathic BVDV was detected in spleen samples from two of 203 deer and both animals were seronegative roe deer.99 Three surveys utilizing immunohistochemistry (IHC) or ELISA investigated the occurrence of BVDV in free-ranging cervids in the United States and results suggest that PI cervids exist in wildlife populations.100–102 In Alabama, one of 406 skin samples from white-tailed deer was positive on IHC and the antigen distribution resembled that of PI cattle.101 The skin sample of one of 5597 deer in Colorado was positive on IHC and this result was confirmed by detection of viral RNA in skin and lymph node samples.100 In Indiana, two of 745 white-tailed deer were positive for BVDV by antigen-capture ELISA with subsequent isolation of cytopathic and noncytopathic BVDV.102 To date, validation of BVDV assays for use in wildlife has not been performed, and this may be critical as considerable variations were observed among the IHC and antigen-capture ELISA on skin samples of white-tailed deer (T. Passler and P. Walz, unpublished observations). In addition to surveys, isolation of BVDV was successful in free-ranging roe deer in Hungary, a mule deer in Wyoming, and two white-tailed deer in South Dakota.97,103,104 In these reports, clinical illness including emaciation, weakness, and death prompted further investigations leading to the isolation of BVDV from tissues. Few reports on the clinical outcome of BVDV infection in wildlife exist, but reported clinical signs are similar to BVDV infections in cattle. Experimental inoculation with BVDV NY-1 did not result in clinical signs in four mule deer and one white-tailed deer fawns, despite evidence of viremia and nasal shedding.105 Similar findings were made in yearling elk that were inoculated with either BVDV 1 Singer or BVDV 2 24515.106 In two young reindeer, loose, bloody, and mucoid feces, transient laminitis, or coronitis were observed after inoculation with BVDV Singer and mild lesions were detected at necropsy.107 Naive white-tailed deer fawns developed moderate pyrexia and marked to moderate decreases in lymphocyte populations in response to either BVDV 1 or BVDV 2; and lethargy or coughing was observed in individual fawns.108,109 Similar to other species, BVDV infections in wildlife may have the most important implications for reproductive health. Experimental inoculation of pregnant white-tailed deer may result in reproductive failure, including fetal resorption, fetal mummification, stillbirth, and abortion.110,111 Infections with BVDV may result in a wide variety of clinical manifestations as the outcome of infection depends on the triad of host-associated factors, environmental stressors, and viral characteristics.112 Host-associated factors include (i) immune status and immunocompetence, (ii) pregnancy status, and (iii) the gestational age of the fetus at the time of infection. Viral characteristics that influence the outcome of an infection include the biotype of the infecting strain and genotypic variation that determine virulence and antigenicity. Infection with both species of BVDV may result in a broad spectrum of clinical manifestations, but severe acute BVD, thrombocytopenia, and hemorrhagic syndrome, as reported in North America, were associated only with BVDV 2.4,5,113 Postnatal infections in cattle that are able to immunologically respond to the BVDV infection are referred to as “acute” or “transient” infections, and the severity of clinical signs depends on the infecting BVDV strain. After infection of susceptible cattle, the tonsils and respiratory tract are the first sites of BVDV replication. Subsequently the pathogen disseminates to many other epithelial and lymphoid tissues, resulting in infection of gastrointestinal, integumentary, and respiratory tissues.114,115 The majority of BVDV infections in immunocompetent seronegative cattle proceed subclinically.3 However, close observation of infected animals usually reveals mild signs including hyperthermia, leukopenia, and decreased milk production. Affected animals develop neutralizing antibodies.116 Symptomatic infections are most commonly observed in 6–24-month-old cattle following waning of maternal immunity, in colostrum-deprived calves, or in seropositive cattle as a result of infection with a heterologous BVDV strain.117 Clinical signs in affected animals include pyrexia, lethargy, leukopenia, ocular and nasal discharge, oral erosions and ulcers, blunting of oral papillae, diarrhea, and decreased milk production. More virulent BVDV strains can also cause epithelial erosions at the interdigital spaces, coronary bands, teats, or vulva. Although BVDV-associated immunosuppression can result in pneumonia, the tachypnea observed in acutely infected animals is likely the result of hyperthermia and other nonpulmonary factors.117 In the 1990s, severe acute BVD was described in cattle herds in the United States and Canada.5,113 The outbreaks were characterized by a peracute course and caused unusually high rates of morbidity and mortality in all ages of cattle. The described clinical signs and postmortem findings were principally those of mucosal disease, with severe diarrhea, fever, and ulcers and erosions of the upper alimentary tract.4 Histopathologic findings included a dramatic lymphocytolysis and lymphoid depletion of Peyer’s patches, necrosis of intestinal crypt epithelium, and diffuse ulcerative lesions in the upper alimentary tract. Sudden death without premonitory clinical signs, abortions, and pneumonia were also prominent findings in some affected herds. Interestingly, the gross and histopathologic postmortem changes were more pronounced and more likely to be observed in older animals, which is in contrast to most previous descriptions of transient BVDV infections.4 The viral isolates from all reports of severe acute BVD were determined to be different from traditional BVDV isolates in genotype and monoclonal antibody binding patterns, prompting the designation BVDV 2.5,15,118 Another form of severe acute BVD associated with BVDV 2 is the hemorrhagic syndrome, which is characterized by marked thrombocytopenia. Hemorrhagic syndrome is observed only in a minority of cattle with severe acute BVD, contributing to a diverse clinical picture in an outbreak.117 Clinical signs in affected cattle include petechiation and ecchymoses of mucosal surfaces, epistaxis, bloody diarrhea, bleeding from injection sites or trauma, fever, and death.113 The marked thrombocytopenia and leukopenia of affected animals is accompanied by altered function of platelets, and thus quantitative and qualitative platelet defects contribute to the observed hemorrhagic diathesis.119 In addition to direct effects on the host organism, BVDV suppresses the number and function of various innate and adaptive immune components, further damaging tissues by enabling infections with secondary pathogens. BVDV-induced immunosuppression is especially important in polymicrobial diseases such as the bovine respiratory disease complex of feedlot cattle and dairy calves. Infection of cells of the innate immune system may result in impairment of function and decreases in the number of circulating leukocytes. The microbicidal, chemotactic, and antibody-dependent cell-mediated cytotoxicity was impaired in neutrophils that were infected with BVDV.120 Infected monocytes may undergo apoptosis, and a reduction of 30–70% in the number of monocytes has been observed after BVDV inoculation of calves.121–123 Infection with BVDV diminishes the ability of antigen-presenting cells to present antigen to T-helper cells by reduction of Fc and C3 receptor expression and downregulation of major histocompatibility complex (MHC)II and B7 molecules.121,124–126 A strain-dependent inhibition of function with reduced ability to kill bacterial and fungal pathogens was observed following BVDV infection of cultured macrophages; however, significant functional inhibition was induced only by virulent strains of BVDV.125 Similar to innate immunity, adaptive immune responses are affected quantitatively and qualitatively by BVDV infections. On infection with BVDV, circulating lymphocytes are reduced and lymphocytes in lymphoid tissues are depleted, and this depletion is strain-dependent.121,127 Of the T-lymphocyte subpopulations, CD8+ cytotoxic T-lymphocytes are most dramatically depleted, followed by CD4+ T-helper cells, with little reduction of circulating γ/δ T-cells.128 In addition to lymphocyte depletion, functional alterations of CD4+ cells are pronounced, and noncytopathic BVDV infections result in a shift toward the Th2 response, with reduced cell-mediated but high levels of humoral immunity.129–131 Virulent BVDV isolates can cause reductions in MHCI expression of infected cells, thus affecting cytotoxic CD8+ cell function; however, this reduction appears to be strain-dependent.125 The humoral immunity to BVDV infection depends largely on the quantity of neutralizing antibodies and the antigenic relatedness of the infecting strain with that of the original exposure. Although an amount of cross-reactive protection is conferred by vaccination against heterologous strains, and even between BVDV 1 and 2, superior protection from infection appears to result from homologous exposure.132,133 While BVDV-associated suppression of the host immune response and respiratory disease are cause for the greatest losses in feedlot and stocker operations, susceptible cow-calf and dairy herds suffer considerable economic damage from BVDV-induced reproductive failure. Reproductive losses as a result of BVDV infection can occur throughout gestation, and BVDV infection at the time of breeding can result in reduced conception rates and embryonic losses. In heifers experimentally infected with BVDV 9 days prior to insemination, a conception rate of 44% was detected compared with 79% in control animals.134 In the same study, other heifers were infected 4 days after artificial insemination (AI) resulting in a conception rate of 60% by day 20; however, significant embryonic loss was detected by day 79 when only 33% of animals were still pregnant. Detrimental effects of BVDV infection on fertility were also reported in field studies, in which poor conception rates, early embryonic losses, and pregnancy losses were detected.135–138 In contrast, heifers exposed to PI calves for 220 days beginning at 50 days prior to the breeding season had the same pregnancy rates as unchallenged control heifers, resulting from development of protective immune responses prior to breeding.139 Highlighting the benefits of a controlled breeding season, this study demonstrated that BVDV infections can be self-limiting in certain herds, when all animals are protected from BVDV during gestation and PI calves are not born.139 Both male and female gonads and the entire female reproductive system are a refuge for BVDV replication.140 In postpubertal heifers experimentally infected with a noncytopathic BVDV, the virus was isolated from the corpora lutea of two animals and BVDV antigen staining was detected predominantly in the stroma of ovarian cortex. In this study, prolonged antigen staining and associated chronic lymphocytic oophoritis were detected from 6 to 60 days following intranasal inoculation.141 Similarly, experimental inoculation of susceptible heifers with cytopathic BVDV resulted in chronic oophoritis for days 5–61 after infection, and BVDV was detected in the ovaries of a subset of cattle immunized with a commercial vaccine containing cytopathic BVDV.142,143 Ovarian inflammation may alter ovarian cytokine concentrations or disrupt tissue macrophages, thus interfering with normal ovarian dynamics, and while the effects of BVDV on ovarian function remain incompletely understood, alteration of follicular formation and inhibition of ovulation were demonstrated following BVDV infection.144 A decrease in the number of subordinate follicles and retarded growth of dominant anovulatory and ovulatory follicles were observed in heifers experimentally infected with BVDV, but the infection did not result in alterations of peripheral estradiol or progesterone patterns.144 In contrast, experimental infection with noncytopathic BVDV 2 days prior to estrus resulted in significantly reduced plasma estradiol concentrations but did not affect progesterone secretion or plasma prostaglandin (PG)F2α concentrations.145 While the average number of follicles in each size category was not affected in BVDV-infected superovulated heifers, infection resulted in the absence or delay of the preovulatory surge of luteinizing hormone, reduced number of corpora lutea and recovered ova/embryos, and oophoritis with necrosis of granulosa cells.146–148 Alteration of sex hormone concentrations with potential for disruption of ovulatory follicles to form a competent corpus luteum was also detected in heifers experimentally inoculated 9 days before synchronized ovulation.149 Collectively, these studies suggest that transient BVDV infections can interfere with normal ovarian dynamics and result in transiently or prolonged reduced reproductive ability.148,150 Other causes for reproductive losses associated with BVDV infection at the time of breeding have been proposed and include disruption of the hypothalamic–pituitary–gonadal axis, deficiency of gonadal leukocytes, and incompatibility of the oviductal and uterine environment for embryonic development.140,150 Infection with BVDV was demonstrated in oviductal cells following intravenous inoculation with noncytopathic BVDV and intrauterine infection with cytopathic BVDV resulting in salpingitis for 21 days.151,152 In uterine flushes from a subset of experimentally infected heifers, BVDV was isolated on days 8 and 16 following infection with BVDV, demonstrating a potential risk for the developing embryo in the uterine environment.152 Widespread BVDV infection of the reproductive system and reduced reproductive performance are described in PI cattle. Extensive BVDV immunostaining and pathoanatomical abnormalities including significantly reduced follicular structures and ovarian and follicular hypoplasia were described in PI cows.153 In addition to ovarian structures, including follicular epithelium, theca cells, cumulus oophorus, stromal macrophages, and walls of small arteries, BVDV antigen is present in oviductal epithelial cells, uterine glandular and luminal epithelia, arterial walls, and smooth muscle of PI cattle.154,155 While oocytes in primordial and secondary follicles did not contain BVDV antigen in one study, approximately 20% of oocytes in all stages of follicular development contained BVDV antigen in another report.145,155 Infections with BVDV can have deleterious effects on the bovine pregnancy, and infections before the third trimester are most harmful. BVDV is highly efficient in crossing the placental barrier, and fetal infections are common with either biotype.156 Increasing rates of fetal infection of 33, 33, 86, and 100% were detected in cows experimentally infected at 4, 11, 18, and 30–34 days after insemination, respectively.157 In ewes infected with BVDV at 18 days of gestation, the virus was detected by reverse transcription polymerase chain reaction (RT-PCR) and IHC in fetal tissues approximately 100 hours following infection, and BVDV-positive cells were more numerous in fetal than maternal tissues.158 Fetal infections occur in an orchestrated fashion, beginning with infection of fetal membranes by 72 hours, followed by infection of bulbus cordis and fetal aorta by 7 days, and subsequent spread to many fetal tissues by days 10–14.158,159 Further dissemination through fetal tissues can be observed as the infection proceeds, and a primary target organ of fetal BVDV infection is the brain.160 While viral antigen can be detected in most tissues from PI fetuses, histopathologic changes are primarily detected in the brain, liver, and spleen.161 Invasion of the fetal brain may occur in a “Trojan horse” mode of invasion, in which BVDV enters the central nervous system (CNS) within infected microglial precursors.162 To date, the mechanism of BVDV entry into the fetus is poorly understood. Infection of pregnant cows with noncytopathic BVDV results in pronounced upregulation of interferon (IFN)-stimulated genes such as ISG15 in the first week after infection, and blood concentrations return to baseline by 45 days after infection.163,164 In heifers carrying persistently or transiently infected fetuses, BVDV infection results in measurable serum concentrations of type I IFN, but this antiviral activity is not capable of preventing fetal infection.164 This may be due to failure of maternal type I IFN and antibodies to enter the fetal bloodstream as a result of the blood–placental barrier.164 Likewise, BVDV infection results in measurable IFN responses in fetal blood, which is pronounced in fetuses infected after the susceptible window for development of persistent infection.165 While IFN responses are also present in PI fetuses and resulting PI calves, these responses are of a more modest and chronic nature and are not able to eliminate the infecting BVDV in the absence of specific adaptive immune responses.165,166 The outcome of fetal infection depends on the viral virulence and biotype, and the gestational age. Fetal death and abortions can occur at any gestational age, but are most common during the first trimester.150 Fetal death during early pregnancy may result in fetal resorption or mummification (Figure 60.1) and expulsion of the fetus may occur up to 50 days following exposure to BVDV.167 Of four heifers infected between 29 and 41 days of pregnancy, two abortions and two fetal resorptions were observed.168 While infections in the preimplantation phase (before day 40 of pregnancy) result in large numbers of pregnancy losses, fetuses that survive infection with noncytopathic BVDV of low to moderated virulence from approximately 40 to 125 days become PI.169,170 Persistent infections can only occur with noncytopathic BVDV strains, and experimental infections with cytopathic isolates do not result in the development of PI calves.170,171 Experimental inoculation of seronegative cattle with noncytopathic BVDV at 75 days of gestation results in persistent infection in up to 100% of fetuses, and this gestational age is commonly used to induce persistent infection in research studies.164,166,172,173 The pathophysiology of persistent infection is incompletely understood, but this phenomenon occurs in fetuses before completion of immune system development, when viral antigens are recognized as self antigens, resulting in negative selection of BVDV-specific lymphocytes.150 The negative selection of reacting CD4+ lymphocytes is highly specific and the exchange of a single amino acid in the BVDV antigen may be sufficient to stimulate immune responses in CD4+ lymphocytes from PI cattle.174 The lack of immune responses results in widespread distribution of BVDV throughout the host’s tissues, and PI cattle shed BVDV in multiple secretions and excretions, including nasal discharge, urine, semen, colostrum, milk, and feces.175 Figure 60.1 Fetal mummification in white-tailed deer. Fetal death was estimated to have occurred at 94 days of gestation, approximately 50 days after BVDV infection of the dam. The mummified fetus was born together with a viable persistently infected fawn after completion of the full gestational period. (a) Mummified fetus as found in birthing nest; (b) fetal membranes removed. Fetal infections during the period of organogenesis (approximately 100–150 days of gestation) may result in congenital malformations, many of which are associated with abnormal development of nervous tissues. Defects of the CNS include cerebellar hypoplasia (Figure 60.2), hydranencephaly, hydrocephalus, porencephaly, microencephaly, spinal cord hypoplasia, and a variety of ocular changes such as cataracts, optic neuritis, retinal degeneration, and microphthalmia.150,176,177 Less commonly, congenital BVDV infection can cause CNS hypomyelination, which has been reported with both species of BVDV.178,179 In addition to CNS abnormalities, congenital BVDV infections can result in brachygnathism, osteopetrosis, thymic aplasia, hypotrichosis, hair coat abnormalities, pulmonary hypoplasia, and growth retardation.150,175,176,180 Figure 60.2 Cerebellar hypoplasia in an 11-day-old bull calf. Calf had clinical signs of cerebellar disease including intention tremors, ataxia, and hypermetria. Histopathologically, diffuse cerebellar hypoplasia characterized by shortened and blunted cerebellar folia with marked decrease in cellularity of the granular cell layer, paucity of Purkinje cells, and a markedly thin molecular layer were identified. Image courtesy of Dr Joanna Hyland, Auburn, Alabama. While abortions are still possible in later stages of gestation, congenital infections following the completion of organogenesis and immunocompetence result in effective immune responses and clearance of the virus. These calves are born with precolostral serum antibody titers and usually appear normal at birth.150 However, one study suggested that calves born with precolostral antibodies against BVDV are more likely to develop severe illness during the first months of life, indicating that late gestational infections with BVDV may not be benign.181 Although PI calves may survive to reproductive age, many are born weak, stunted, and die shortly after birth.112 PI cattle have a 50% greater risk of leaving the herd before the first year of age, dying, or being slaughtered due to unthriftiness as compared to normal cohorts, which may be partly due to a poor immune response and susceptibility to opportunistic infections.182,183 An additional risk for PI cattle is the development of fatal mucosal disease, which develops when cattle that are PI with a noncytopathic BVDV become superinfected with a cytopathic strain. The severity of the developing clinical signs depends on the homology between both strains, and mucosal disease can be classified as acute, chronic, or delayed onset.184 Acute mucosal disease occurs when the superinfecting cytopathic strain is in close antigenic homology with the PI strain, which most commonly is the result of mutational events that alter the PI strain’s biotype.185 Because of the close homology of both strains, acute mucosal disease results in case fatality rates that approach 100%.117 Because acute mucosal disease affects only PI cattle, the disease is characterized by a low morbidity but very high mortality.117 However, in groups of cattle with multiple PI cattle that are infected with similar BVDV strains, outbreaks of mucosal disease have been reported.186 Clinical signs of acute mucosal disease include fever, anorexia, tachycardia, polypnea, nasal discharges, and profuse foul-smelling diarrhea with mucosal shreds, fibrin casts, and blood. In addition, erosion and ulcers are commonly present on the alimentary epithelia (Figure 60.3), coronary bands, prepuce, and vulva.117 Figure 60.3 Severe esophageal erosions and ulcers in a steer with acute mucosal disease. Image courtesy of Dr Jenna Bayne, Auburn, Alabama.
Infectious Agents: Bovine Viral Diarrhea Virus
Introduction
Prevalence in cattle populations
Host range
BVDV in small ruminants
BVDV in swine
BVDV in New and Old World camelids
BVDV in wildlife
Clinical disease syndromes
Postnatal infections in immunocompetent cattle
Infections of the reproductive organs
Infections of the conceptus
Infections in persistently infected animals (mucosal disease)
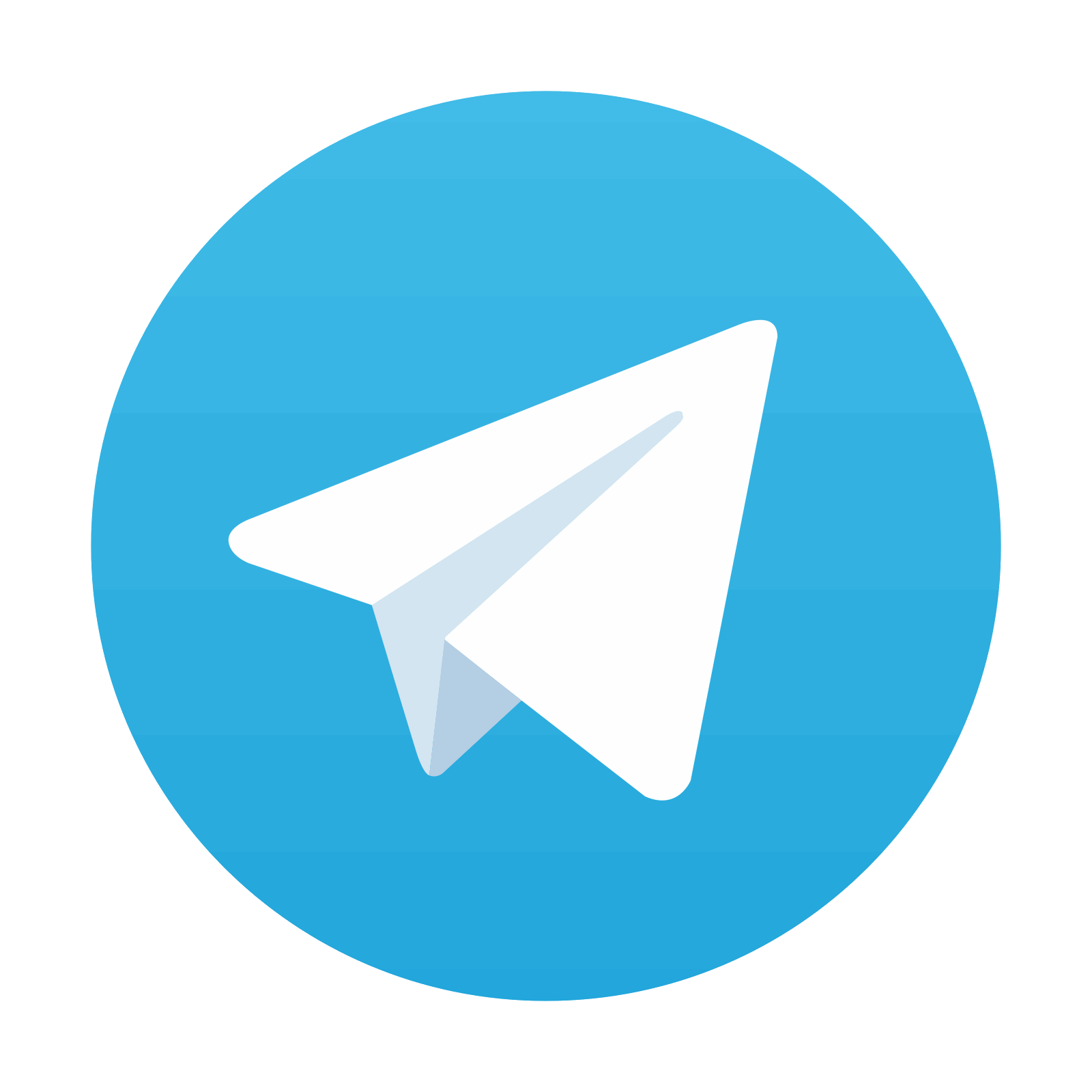
Stay updated, free articles. Join our Telegram channel

Full access? Get Clinical Tree
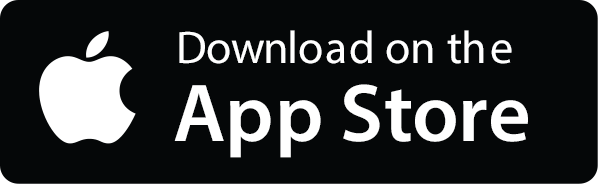
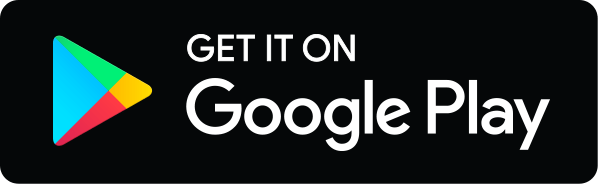