CHAPTER 72 Immunotherapy
As our understanding of immune responses and the pathogenesis of infectious disease has increased over the last two decades, there has been a concomitant increased interest in immunotherapeutics. Immunomodulators are substances that enhance or suppress immune responses (Table 72-1). This diverse group of therapeutic agents includes both nonspecific immunomodulators and drugs with highly selective targets within the immune system. For treatment of horses, recommendations are difficult because of the limited number of studies evaluating their efficacy in that species.
IMMUNOSTIMULANTS
Induction of Nonspecific Immune Responses
Many immunostimulants activate innate immunity and promote release of endogenous immune mediators (e.g., cytokines) as an aid in the treatment of immunodeficiency conditions, chronic infections, or cancer. In the 1890s, Dr. William Coley, a surgeon at New York Memorial Hospital, used killed Streptococcus pyogenes and Serratia marcescens (Coley’s vaccine) to treat sarcomas, carcinomas, lymphomas, melanomas, and myelomas in his patients.1 This treatment originated from the observation that tumors regressed when spontaneous acute infections occurred, especially with high fever. Coley and others initially used live bacteria to induce infection and fever; however, fatal infections eventually led to the use of an inactivated organism.
Immunostimulants induce a nonspecific activation of the immune system, unless they are associated with antigens (e.g., adjuvants in vaccines), and may amplify different effectors of the immune response, including phagocytosis and intracellular killing of organisms, antigen presentation, cytotoxic and antiviral activity, cytokine release, and antibody production.2 Immunomodulators predominantly activate macrophages and dendritic cells in the liver, spleen, skin, and lungs.3 The route of administration is designed to bring the drug into contact with antigen-presenting cells (APCs). In the horse, pulmonary intravascular macrophages are likely important for recognition of foreign antigens in circulation.4,5 These large, mature, permanent resident macrophages of the pulmonary capillary lumen phagocytose particulate material in the circulation, including bacteria, endotoxins, fibrin, and leukocytes. These cells likely secrete inflammatory mediators that result in alterations in systemic vascular resistance and permeability, are chemoattractants for neutrophil margination into the pulmonary vascular system, and produce additional proinflammatory mediators.
The innate immune response is a nonspecific recognition of and subsequent response to pathogens. Signal transduction pathways activate oxidative burst activity and the production of cytokines and chemokines. These responses initiate microbial defenses and inflammation. Toll-like receptors (TLRs) are type I transmembrane proteins expressed in cells responsible for this first encounter with a pathogen and the presentation of processed peptide to lymphocytes: macrophages, dendritic cells, and in some species, mucosal epithelial cells and dermal epithelial cells. Therefore, activation of TLRs may be induced in conditions in which an immune response is desired (e.g., vaccination). Blocking of TLR pathways may be beneficial to prevent life-threatening inflammation (e.g., sepsis).10 In addition to TLRs, other important receptors, including mannose and complement receptors, are expressed on APCs.
The contribution of TLRs to the immune response was first observed with Aspergillus fumigatus infection of Drosophila.7,8 Since then, as many as 12 TLRs have been identified in most mammals.9 Each TLR recognizes a different pathogen-associated molecular pattern (PAMP); therefore, together they can mediate response to a wide range of organisms (Figure 72-1). In addition, TLRs may recognize low-molecular-weight synthetic molecules.
After antigen processing of an immunostimulant, intracellular signaling pathways for expression of proinflammatory genes and endogenous cytokines (IL-1, IL-6, TNF-α, and IFN-α) are activated (Figure 72-2). These mediators, while promoting the desirable immune responses, exert adverse systemic effects, including transient fever, lethargy, and decreased appetite.6 Toxic effects of these crude or live bacterial products include increased vascular permeability, hypotension, pulmonary edema, diarrhea, hypersensitivity reactions with infiltrative/granulomatous cell reaction, autoantibody production, and collapse.
Bacterial Particles
Propionibacterium acnes
Propionibacterium acnes is a gram-positive bacterium studied since the 1950s (when it was known as Corynebacterium parvum) for its antitumoral and antiviral properties. Immunostimulatory activity has been demonstrated in vitro and in vivo in humans and mice. These actions include (1) stimulation of monocytes and macrophages with production of inflammatory cytokines (IL-1, IL-6, and TNF-α), chemokine production (IL-8), phagocytosis and reactive oxygen species activity; (2) activation of CD8+ T cells (CTLs, cytotoxic T cells), natural killer (NK) cells, and lymphokine-activated killer (LAK) cells with interleukin-2 (IL-2) and interferon-alpha (IFN-α)–dependent cytotoxicity; (3) increase in resistance to intracellular bacteria and viruses in mice; (4) in vivo antitumoral effect; and (5) potential adjuvant with antigens.11–14 The recognition of P. acnes PAMP is mediated by the TLR2 and TLR9 on monocytes and macrophages. Activation results in nuclear factor kappa B (NF-κB) activation and expression of interleukin-6 (IL-6), interleukin-8 (IL-8), and interleukin-1 (IL-1), leading to a T-helper cell type 1 (Th1) immune response.15,16,16a
Propionobacterium acnes (Eqstim, Neogen Corp., Lexington, Kentucky) is licensed for adjunctive therapy in the treatment of viral and bacterial infections of the respiratory tract of the horse in association with other conventional therapy. In healthy young horses, a series of three intravenous (IV) injections of P. acnes extract resulted in immunomodulatory responses.17 It is suspected that the bacterial extract is rapidly taken up by the intravascular pulmonary macrophages, which in turn produce inflammatory cytokines and clinical effects (indicated by mild fever). In peripheral blood, nonopsonized phagocytosis is increased. In bronchoalveolar lavage (BAL) fluid, there is a decrease in total leukocyte counts, especially lymphocytes, with a proportional increase in macrophages. The CD4+/CD8+ T-lymphocyte ratio and LAK cell activity are increased in peripheral blood and BAL fluid after 3 days of administration of this immunostimulant. Peripheral blood mononuclear cells exhibit an increase in interferon-gamma (IFN-γ) and the NK-lysin antimicrobial peptide for up to 7 days after treatment.18
An open, randomized clinical trial demonstrated that administration of two doses of P. acnes before shipping reduced the incidence of transport-stress–induced respiratory disease by more than 60% compared with horses in a placebo-treated group.19 In two blinded, randomized clinical studies of horses with naturally occurring respiratory disease treated with conventional therapy, 79% and 96% of the horses that received P. acnes recovered within 14 days of treatment, compared with 47% and 35%, respectively, of the horses from the placebo group.20
Bacille Calmette-Guérin Vaccine and Mycobacterium Cell Wall Extracts
Microbiologists Calmette and Guérin from the Pasteur Institute took 13 years to attenuate a strain of Mycobacterium bovis by 230 serial passages in culture. This resulted in a loss of virulence without loss of immunogenic properties in the attenuated strain. Since then, genetic drift has resulted in different strains of bacille Calmette-Guérin (BCG), which induce the distinct immunogenic and therapeutic effects observed at present.21 BCG was originally created for use as a vaccine against Mycobacterium tuberculosis in humans. Use as a nonspecific immunomodulator began in the 1970s for treatment of superficial bladder cancer.22,23 Currently, BCG cell wall fractions and recombinant BCG (rBCG) combined with foreign antigens (viral, bacterial, or parasitic particles) or cytokines (e.g., human IFN-α gene) are available for clinical use and research.24 The recombinant forms are studied for their application as adjuvants in specific immune responses.
Low-dose BCG vaccine and inactivated Mycobacterium preparations have a potent immunomodulatory effect on macrophages and dendritic cells primarily via TLR2 and TLR4.25,26 In addition to enhanced production of the cytokines IL-12, IL-1, and tumor necrosis factor alpha (TNF-α), BCG-stimulated dendritic cells promote the upregulation of CD80, CD86, and CD40, which are important co-stimulatory molecules for T-cell activation. BCG vaccine–stimulated monocytes and macrophages induce Th1-type CD4+ T-cell proliferation in vitro.27 BCG vaccine stimulates NK cell cytotoxicity and IFN-γ production via monocyte and macrophage IL-12 secretion. A comparable direct effect of BCG vaccine on NK cells in the absence of APCs has also been demonstrated in vitro.28,29 In addition, BCG vaccine stimulates the expression of integrins, IL-1α and IL1β, IL-8, macrophage inflammatory protein 1α and 1β (MIP-1α, MIP-1β) and resistance to apoptosis in neutrophils.30 Together, the immunostimulatory effects promote neutrophil and macrophage attraction and activation, which subsequently induces Th1-type CD4+ T-cell activation and NK-cell cytotoxic response.
A randomized, double-blind clinical study in horses with naturally occurring respiratory disease and treated with conventional therapy suggested that 83% of horses receiving one IV dose of purified Mycobacterium spp. cell wall extract recovered from respiratory clinical signs in a shorter time (7 days) than the placebo group (36%).31 Live BCG vaccine or preparations of BCG cell wall fraction (Regressin-V, Bioniche Animal Health) have been used with quantifiable success (about 60%-70%) for intratumoral treatment of sarcoids in horses.32 This therapy is more efficacious for treatment of facial lesions and in horses with a positive delayed hypersensitivity reaction to purified protein derivative before treatment and leukocytosis after the first injection.33 Nonresponsive cases are associated with lesions elsewhere on the body (limbs, body wall), large size, or the presence of multiple sarcoid lesions.
Adverse reactions to BCG vaccine (attenuated form of mycobacterium) are more common in children. These reactions may include persistent local abscess at the site of injection and regional suppurative lymphadenopathy.34 Adverse reactions to intravesical instillation of BCG vaccine for the treatment of superficial bladder carcinoma in human patients include disseminated BCG infection in distant organs: granulomatous hepatitis, miliary pulmonary disease, pulmonary granulomas, renal abscess, and retroperitoneal lymphadenopathy.35 In addition, sepsis and hypersensitivity reactions (fever, pancytopenia, anaphylaxis, hepatitis, renal insufficiency) have been reported.36 Pulmonary toxicity could be septic and secondary to mycobacteremia, which induces sensitization before a second BCG exposure. Hypersensitivity reactions a few weeks after immunotherapy may be observed in patients with disseminated pulmonary and hepatic granulomas, characterized by noncaseating epithelioid granuloma with Langhans-type giant cells and lymphocytes. In these cases, cultures for mycobacteria from blood and affected tissues are negative.35,37
In horses, adverse effects after immunotherapy with mycobacterial preparations include reaction at the injection site, fever, lethargy, and decreased appetite, likely related to the induced endogenous cytokine release. An acute reaction with pancytopenia, fever, hypotension, tachycardia, and tachypnea has been observed in a horse treated intralesionally for a sarcoid lesion (Flaminio, unpublished data). This horse had been previously treated with a BCG formula several months before this reaction. Four horses with a history of cough developed severe inflammatory reaction in the respiratory tract after immunomodulator administration. These horses had interstitial pneumonia, multifocal pulmonary granulomas, and bronchiolitis, with subsequent development of lung fibrosis despite glucocorticoid therapy.38 Because of these types of adverse reactions, veterinary mycobacterium compounds have been removed from the market in some countries.
Unmethylated Cytosine-Phosphate-Guanosine Motifs
Cytosine-phosphate-guanosine (CpG) motifs are unmethylated dinucleotides present in high frequency in bacterial but not in vertebrate genomes. CpG motifs are recognized by TLR-9 expressed in APCs.39 Synthetic oligodeoxynucleotide (ODN) motifs can mimic natural CpGs and stimulate macrophages, dendritic cells, and B cells. In general, CpG-ODN motifs induce a Th1-type immune response, with both cellular and humoral components.40 Therefore, CpG-ODNs can be potentially used as immunomodulators for treatment of immune-mediated disorders or as adjuvants in vaccines.41–43
In murine B cells, CpG motifs induce robust T-cell– independent B-cell proliferation; enhance production of IL-6, IL-10, and antibodies; promote isotype switching; and prevent B-cell apoptosis.44 In monocytes, macrophages, and dendritic cells, CpG motifs induce the production of large quantities of IL-12, and smaller quantities of IFN-α, TNF-α, and IL-6.45 There is maturation of dendritic cells with upregulation of cell surface major histocompatibility complex class II (MHC II) molecule and co-stimulatory molecules, including CD86.46 Therefore, these APCs become more capable of presenting processed antigen to T cells and promoting their activation. The increased production of IL-12 by APCs results in potent stimulation of NK cells and T cells, with subsequent IFN-γ release.
There is species specificity in the recognition of CpG motifs, and certain CpG motifs may have dissimilar effects on immune cells according to their sequence.47 CpG-ODN– induced proliferation in vitro correlates well with in vivo responses, and this method is used to screen different motifs for clinical application.48 Other synthetic products that contain a bicyclic heterobase in which the C in CpG is replaced by R (rybofuranosyl, RpG) are currently under investigation.49
As with many other immunomodulating agents, CpG motifs may be toxic and promote an undesirable, exacerbated immune response. Toxic responses to CpG motif have been demonstrated in mice after repeated administration of high doses of CpG motifs. A lethal synergism is observed when CpG motif administration is followed by endotoxin (lipopolysaccharide, LPS) challenge.50 Despite promoting B-cell activity, CpG does not induce an anti-DNA antibody response or accelerate autoimmune disease. Further studies of the use of this immunomodulatory agent are necessary to identify CpG sequences with biologic effect, the type of response generated, potential clinical applications, and safety in the horse.
Exogenous Cytokines
Cytokines are proteins or glycoproteins secreted by immune cells and other cells in direct contact with microorganisms. Their effect is primarily autocrine or paracrine, and they function as messengers or mediators of the immune system.70 Interleukins and other cytokines may be administered as immunomodulating agents to stimulate enhanced immune activity in the treatment of infectious, neoplastic and autoimmune diseases.
Interferon-α
Endogenous interferons (IFNs) are multifunctional proteins that play important roles in antiviral defense and cell proliferation/viability by binding to cell surface receptors on virus-infected cells and inducing transcription of specific genes. High IFN levels can control the rate of virus replication in early infection.51 Type I IFNs are produced primarily by the innate immune system leukocytes (e.g., IFN-α) or fibroblasts (e.g., IFN-α and IFN-β) after viral infection.52 Viral binding to extracellular receptors or the presence of viral products (dsRNA) bound to cytoplasmic receptors can induce expression of type I IFNs. Type II IFNs (IFN-γ) are produced by NK cells and T cells (in response to cytokines IL-12 and IL-18). Both types of IFN promote an “antiviral state” by interfering with the mechanisms of cell proliferation, translation, and subsequently, viral replication. In addition, IFNs make infected cells more susceptible to apoptosis (procaspase activity) and to recognition by CD8+ cytotoxic T cells by enhancing the expression of major histocompatibility complex class I (MHC I) on infected cells.
One of the most important antiviral activities of IFNs is induction of proteins that prevent proliferation or promote destruction of ribonucleic acid (RNA) molecules in the cell. These include the dsRNA-dependent protein kinase R, which controls transcription and translation of other genes with antiviral effect (e.g., NF-κB). The gene 2′-5′-oligoadenylate synthetase inhibits protein synthesis by promoting RNase activity. The Mx proteins interfere with virus polymerase and upregulate adenosine deaminase (ADAR), which is important for RNA editing, effectively inhibiting viral multiplication. Caspase proteins promote apoptosis.53 IFNs are so important in the control of viral infection and replication that many viruses have developed mechanisms to inhibit the IFNs.54
Besides the potent direct antiviral effects of IFN-α, this cytokine promotes cell-mediated immunity. It enhances the cytotoxic effects of NK cells and LAK cells by increasing levels of perforins and granzymes (apoptotic effect), production of IFN-γ, and cell proliferation. Once IFN-γ secretion is enhanced, this stimulation is self-sustained with cell-to-cell contact for a time, even in the absence of additional IFN-α.55 IFN-γ induces activation and maturation of APCs, potentiated even further by IL-12 and IL-15. IL-12 drives CD4+ T cells into a Th1-type response, resulting in additional IFN-γ production. Interleukin-15 supports the survival and proliferation of activated and memory T cells.56 In addition, IFN-γ induces the production of reactive oxygen (O2−, superoxide; OH−, hydroxyl; H2O2, hydrogen peroxide; HClO−, hypochlorite) and reactive nitrogen (NO, nitric oxide; NO2, nitrogen dioxide; HN2, nitrous acid) products in phagocytes for effective microbial killing.57
Commercially available IFN-α is found in natural (in vitro purified human IFN-α with multiple subtypes) and recombinant forms (Escherichia coli clone expressing human IFN-α-2a subtype DNA). The natural form of IFN-α may provide broader biologic function compared with the single recombinant subtype. Low-dose IFN-α therapy is more efficient in antiviral activity than high doses, which may induce an excessive inflammatory response, downregulation of IFN receptors, and production of neutralizing antibodies. Studies using IFN-α as an adjuvant for influenza peptide vaccines in mice revealed a potential benefit in the induction of cytotoxic T lymphocyte (CTL) activity.58
IFN-α has been used therapeutically via oral or parenteral (subcutaneous, intramuscular) routes in people and horses for its antiviral and antiproliferative (antitumoral) activities. Parenteral high-dose human IFN-α-2a has been used to treat human patients with chronic hepatitis C, West Nile virus (WNV) infection, hairy cell leukemia, chronic myelogenous leukemia, and AIDS-related Kaposi’s sarcoma.59–62 Adverse effects associated with parenteral high-dose IFN-α-2a treatment include fever, fatigue, and myalgia. Contrasting results of in vitro experiments question the susceptibility of WNV to IFN-α. There is evidence of both resistance and susceptibility of the virus to the cytokine.63,64 Nevertheless, human patients infected with WNV treated with high parenteral doses of IFN-α had rapid recovery and fewer sequelae. Additional clinical trials are underway.65
Oral IFN-α acts directly on oropharyngeal-associated lymphoid tissues by activating the antiviral state in those cells.66 In horses, low-dose oral administration of natural human IFN-α has been used for the treatment of inflammatory airway disease and viral respiratory diseases.67 In a double-blind, randomized, block-design study of horses with inflammatory airway disease (characterized by poor performance and exudate in the upper and lower airway), natural human IFN-α given orally reduced airway inflammation, pharyngeal lymphoid hyperplasia, nasal discharge, and cough compared with horses receiving placebo. The cytokine profile of BAL fluid cells returned to normal in horses that received oral IFN-α. A commercially available recombinant IFN-α-2a, which contains only one subtype of IFN-α, failed to reduce virus shedding and respiratory disease in experimental herpesvirus type 1 infection in horses.68 A double-blind, randomized clinical trial testing the effect of oral treatment with natural or recombinant human IFN-α on inflammatory airway disease revealed that both IFN-α forms decreased the time to recovery and number of relapses compared with placebo.69 In horses, IFN-α has been used parenterally for the treatment of WNV; however, to date, randomized, placebo-controlled trials have not been completed.
Other Cytokines: the Present and the Future
The two most common cytokines used in cancer immunotherapy in human patients (e.g., renal cell carcinoma) are IL-2 and IFN-α, which can be used independently (high doses) or in combination (lower doses).71 Because only a small percentage of patients respond to cytokine therapy alone, this mode of treatment is often combined with traditional chemotherapy.72 Effective response and toxicity are both dose dependent with this cytokine; therefore, to minimize systemic effect, peripheral blood or tumoral infiltrating lymphocytes (TILs) can be activated and expanded with cytokines (IL-2) in vitro and returned to the patient for a potentiated effect against tumor cells.73 Hyporesponsive lymphocytes from the malignant effusion of lung cancer patients can be reactivated into CTLs when treated in vitro with IL-2 (or IL-15) and anti-CD3 antibodies.74
The use of cytokines in combination with conventional antibiotic therapy or as vaccine adjuvants has been beneficial in the treatment and prevention of infection with intracellular bacteria. The use of IL-12 alone has limited clinical application, but it has promising adjunctive properties to induce Th1-type immune response when paired with peptides in vaccines. In murine models, intranasal administration of IL-12 in combination with an antibiotic or antifungal medication improves survival and clearance of Mycobacterium avium, Francisella tularensis, influenza virus, or cryptococcal infection.75–78 In human patients with asthma, treatment with IL-12 with the objective to inhibit Th2-type and promote Th1-type responses failed to improve airway hyperreactivity. Work with a cytokine fusion protein consisting of allergen (or allergen cDNA) fused to IL-12 or IL-18, or allergen cDNA fused with IL-18 cDNA, reveals redirection of immune responses into a Th1 type and reduction of airway hyperreactivity in a mouse model.79,80
Plasmid DNA coding for human IL-12 has been injected directly into equine melanoma tumors and promoted tumor regression with no adverse effects.81 The antitumor effect of the plasmid DNA encoding IL-12 has been observed in murine tumor models; however, many of these therapies failed when applied to human patients with cancer.
The use of other cytokines to induce Th1-type immune response in clinical patients has had limited effect. Administration of IFN-γ to prevent Pseudomonas aeruginosa infection in a stress mouse model revealed recovery of IL-12 secretion but did not improve bacterial clearance or reduce mortality.82
Neonatal mice treated with fms-like tyrosine kinase 3 (Flt-3) ligand, a hematopoietic growth factor for dendritic cells, B cells, and NK cells, demonstrated a 100-fold increase in the innate resistance to herpes simplex virus type 1 and Listeria monocytogenes.83 The dendritic cell induction was independent of mature T and B cells and their cytokines. This finding has important implications in neonatal immune defense because the adaptive immune system is not well developed.
Granulocyte-macrophage colony-stimulating factor (GM-CSF) is a cytokine used to reconstitute myeloid cells in the bone marrow and promote macrophage and dendritic cell development. This cytokine has also been evaluated as an adjuvant in vaccines or in combination with other cytokines (IFN-γ) to promote dendritic cell maturation for use in cancer immunotherapy. In the horse, subcutaneous administration of recombinant human granulocyte colony-stimulating factor (G-CSF) stimulates in vitro bone marrow cells from Standardbred horses with familial neutropenia and accelerates bone marrow production of neutrophils in foals with alloimmune neonatal neutropenia.84,85 Response to G-CSF is immediate, and in the presence of normal myeloid precursors in the bone marrow, neutrophil counts may be within normal reference ranges within 48 hours of administration.
Other Immunomodulators
Parapoxvirus ovis
The use of poxvirus as an immunostimulant originated from observations related to the smallpox eradication program, in which vaccinated human patients had improvement in viral diseases and tumors. In vitro studies using murine, human, bovine, ovine, and swine cells suggest that the Parapoxvirus envelope contains proteins that promote the activation of NK cells, enhance phagocytic activity, and increase the release of INF-α, IL-2, TNF-α, and GM-CSF.86 The mechanism of stimulation of APCs involves the CD14 molecule, with production of antiinflammatory IL-10 and Th2-type IL-4 cytokines.87 Pretreatment with Parapoxvirus ovis protected mice in a dose-dependent manner from lethal vesicular stomatitis virus and herpes simplex virus type 1 infections.88
Administration of Parapoxvirus ovis (ecthyma virus or Orf virus) strain D 1701 is indicated for the prophylaxis of stress-induced respiratory diseases caused by transportation, hospitalization, and weaning; for the metaphylaxis and therapy of infectious diseases; and to enhance immunization response. In the horse, a blinded field study suggested that prophylactic administration of Baypamun (Bayer, Germany) to foals 6 and 4 days before weaning, and at 5 days thereafter, assisted in preventing and reducing the incidence of respiratory disease from 24% to 7.9%.89 In a controlled field trial, Thoroughbred foals from the same farm received three doses of the immunotherapeutic drug or placebo immediately after birth and 24 or 48 hours later. These foals were monitored for 4 weeks, and 20% to 30% of the foals from the placebo group developed respiratory infections, whereas the foals in the groups receiving the immunomodulator did not. Parapoxvirus ovis was suggested to minimize but not to prevent respiratory clinical signs (based on nasal exudate scores) in horses naturally challenged by contact with virulent equine herpesvirus type1 or type 4 (EHV-1 or EHV-4). Young horses under stress induced by weaning, transportation, and commingling that were treated with this immunomodulating agent were more resistant to EHV-1 and EHV-4 infection, as well as development of respiratory clinical signs, when receiving Parapoxvirus ovis, compared with horses receiving placebo.
Levamisole Phosphate
Levamisole phosphate is a synthetic anthelmintic with reported immunomodulatory properties that have been rarely observed and poorly characterized in vitro and in vivo. In horses the effect of levamisole was tested in pregnant mares with weekly injections starting 4 to 6 weeks before parturition. Colostrum of mares receiving the immunostimulant had greater immunoglobulin G (IgG) and IgG(T) concentrations than did colostrum from control mares.90 In sick foals, levamisole has been administered in the attempt to improve cell-mediated responses and phagocytic activity.
Caprine Serum Fraction
Caprine serum fraction is a sterile, filtered, purified, and standardized fraction of goat serum preserved in phenol and thimerosal (Pulmo-Clear, Colorado Serum Co., Denver). It is recommended as an immunomodulator for adjunctive therapy of lower respiratory tract disease in horses. A clinical efficacy trial in horses with unspecified lower respiratory tract disease suggested improvement in airway inflammation, as evaluated by endoscopic examination score after two doses of the immunomodulator.91
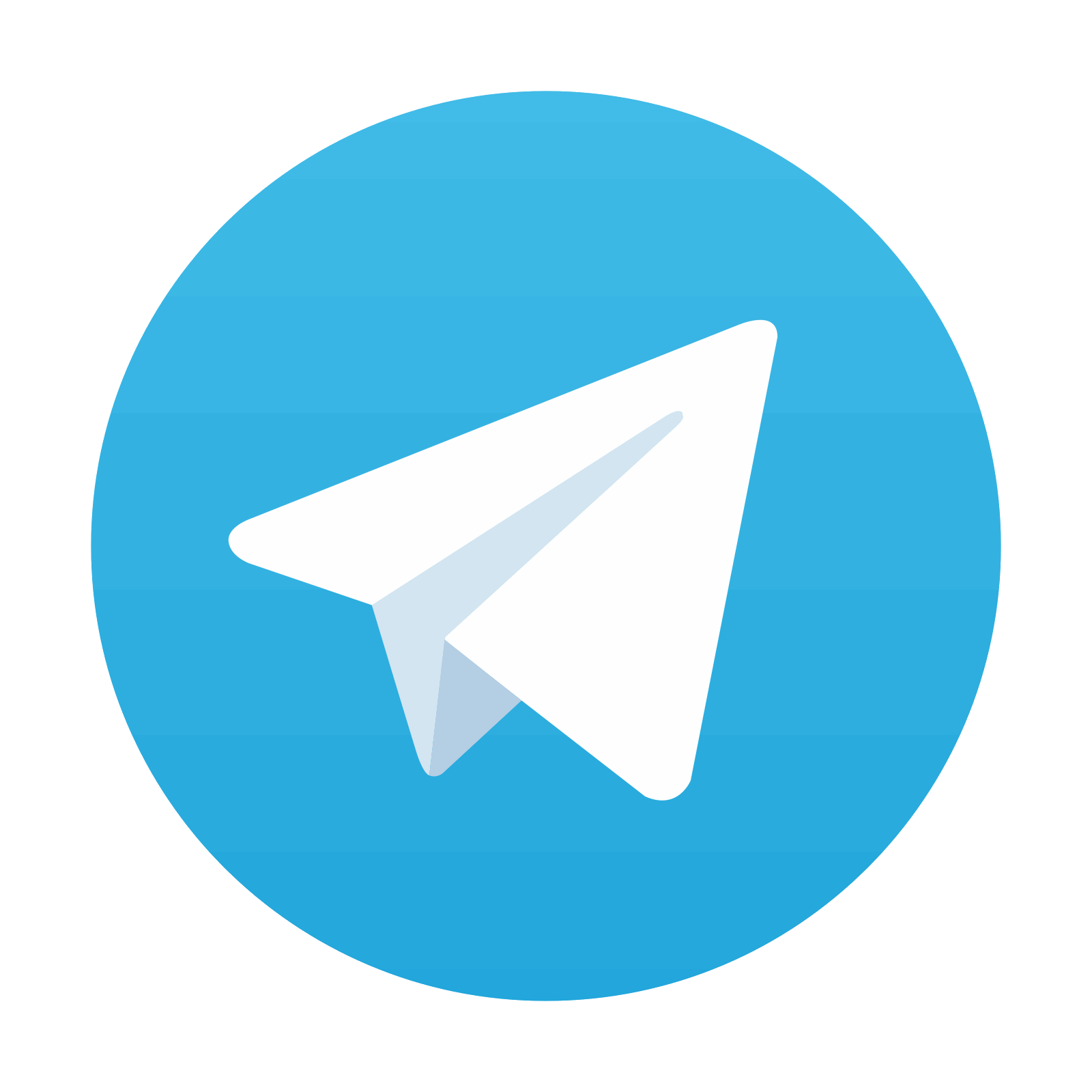
Stay updated, free articles. Join our Telegram channel

Full access? Get Clinical Tree
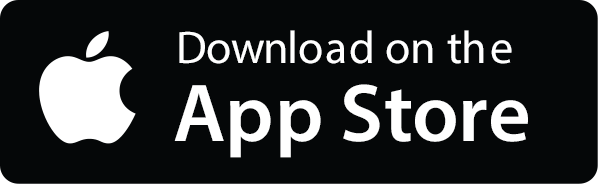
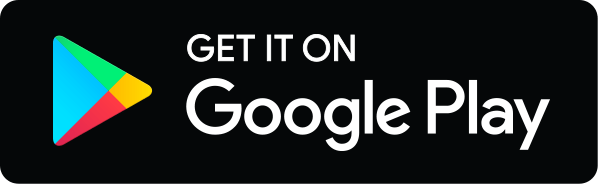