CHAPTER 70 Immunoprophylaxis
Immunoprophylaxis is the prevention of infectious disease through induction or enhancement of specific protective immune responses. Immunity can be passively acquired by natural transfer or by exogenous administration of humoral or cellular factors from animals previously immunized through natural exposure or vaccination. However, the preferred approach is to induce protective responses actively through administration of vaccines containing (1) inactivated or live pathogens modified to attenuate their pathogenicity, (2) microbial components, (3) inactivated microbial products such as toxoids, or (4) genetic material encoding for expression of protective antigens. Of the infectious agents, viruses typically have the least complex set of antigenic determinants involved in protective immune responses; therefore, viral diseases are generally more amenable to control through active immunization than diseases caused by bacteria and, to an even greater extent, by fungal, protozoal, and metazoal agents.
ACTIVE IMMUNIZATION
Basic Principles
Active immunization typically involves administration of a primary series comprising one or more doses of vaccine to “prime” the immune system and generate effector proteins (antibodies) as well as clones of memory cells (lymphocytes and plasma cells) that are the basis for immunologic memory and “recall.” Typically, “booster” doses of vaccine are administered periodically to enhance the level of specific antibody and memory. On subsequent exposure to the specific pathogen, memory cells can be recruited to quickly generate specific antibodies and effector cells such as cytotoxic T lymphocytes (CTLs) to neutralize the pathogen before it causes disease. Some vaccines are capable of inducing sterile immunity, in which case infection and replication of the pathogen are completely blocked, whereas many vaccines induce clinical protection without completely blocking infection or replication of the organism, although the latter is typically at a much reduced level.
Types of Vaccines
Dead Vaccines
Inactivated Pathogen Vaccines.
Inactivated pathogen vaccines are the most common form of equine vaccine in current use and comprise microorganisms that have been treated with heat or chemicals to inactivate them while preserving their immunogenicity (Table 70-1). Phenol, formaldehyde, and β-propiolactone are among the inactivating agents used most frequently in the preparation of inactivated bacterial and viral vaccines.1 Inactivated vaccines are inherently biologically safe because they have, in theory, no residual virulence and have a high stability in storage. They are typically suitable and safe for use in pregnant mares, debilitated or immunocompromised animals, geriatric horses, and colostrum-deprived foals. However, such vaccines typically require multiple doses and regular boosters, and efficacy frequently depends on use of potent adjuvants and high antigenic mass. Compared with vaccines of other types, disadvantages often associated with inactivated vaccines include slow onset of immunity, increased risk of local and allergic complications (high antigenic mass and inclusion of adjuvants are two factors that increase the likelihood of reactions), need for multiple doses in the primary series, need for parenteral (typically IM) administration, need for regular boosters at frequent intervals to maintain reasonable immunity, and shorter duration of immunity than achieved with modified live vaccines. Further, because inactivated vaccines are known to be weak inducers of cell-mediated immunity, they are not very efficient in eliminating virus-infected cells.2
Protein or Subunit Vaccines.
Recombinant DNA technology permits synthesis of specific antigens that are important for immunity to pathogens. Such subunit immunogens can be produced only if the genetic sequence encoding for expression of the specific protective protein antigens, as well as the immunogenic characteristics of these proteins, are known. Recombinant subunit equine herpesvirus type (EHV) 1 and equine influenza vaccines have been produced experimentally but, as yet, have shown poor protection, probably because of the poor immunogenicity of the recombinant proteins.3,4
Live Vaccines
Modified Live Vaccines.
Attenuation usually is achieved by in vitro passage through one or more cell types, selection of spontaneous or induced temperature sensitive mutants, use of reassortants obtained by co-infection of the same cell with two different viruses with segmented genomes, or use of chemicals that induce mutations.5–7 Several modified live vaccines are currently marketed for use in horses, including vaccines for prevention of equine influenza, strangles, EHV-1, and equine viral arteritis (EVA).
Recombinant Vectored Vaccines.
Viruses and bacteria can be genetically engineered to serve as carriers or vectors for the expression of foreign DNA through the use of recombinant technology. A prerequisite to using this technology is thorough definition of the protective antigens of the specific pathogen of interest. Such vectors allow the introduction of the transgene into host cells, leading to production of protein antigens and stimulation of both B-cell and T-cell responses.8 Adenoviruses and herpesviruses are being considered and evaluated as potential vaccine vectors, and a recombinant modified vaccinia Ankara virus (rMVA) vaccine for equine influenza has already shown efficacy in challenge studies in horses.9,10 In addition, a variety of recombinant poxviruses are currently commercially available. In contrast to many poxviruses, which have a very broad vertebrate host range, the canarypox and fowlpox viruses are host restricted to certain avian species and produce an abortive infection in mammals. Canarypox and fowlpox are therefore ideal candidate vectors for mammalian vaccines because they express the inserted foreign genes in the absence of productive replication. Avian poxvirus–vectored vaccines have the potential to induce a broad array of immune responses in the absence of an adjuvant, although the canarypox-vectored West Nile virus (WNV) and influenza vaccines currently marketed for use in horses do contain a polymer adjuvant.11–13 Further, it appears that canarypox virus recombinants do not trigger a neutralizing response against the vector, which would preclude an immune response against the transgene when subsequent booster doses of vaccine are administered.
Chimera Vaccines.
Chimera vaccines are similar to live vectored vaccines in that they use components of one viral agent to transport genes encoding for expression of important protective antigens of the pathogen of interest. In the case of chimera vaccines, the vector and the pathogen of interest are typically in the same family. To date, this technology has been applied most extensively to immunize against dengue, Japanese encephalitis (JE), and WNV, all of which are flaviviruses, using the highly attenuated 17D vaccine strain of the related flavivirus, yellow fever virus (YF), as the vector. The premembrane (prM) and envelope (E) structural proteins of the pathogen of dengue, JE, or WNV are exchanged for the homolog proteins of YF 17D using complementary DNA (cDNA) templates encoding for these proteins.14 A single dose of the nonadjuvanted YF-WNV chimeric vaccine generated using this technology has been shown to induce solid protection in horses subjected to intrathecal challenge with virulent WNV as a part of USDA immunogenicity studies as required for licensing. In addition, this vector, while affording high levels of protein expression, does not replicate to detectable levels in the horse, thereby further enhancing its safety.15
DNA Vaccines
Another novel vaccination technology currently under investigation, and recently licensed for use in an equine WNV vaccine, is DNA vaccination. The basis for DNA immunization is that host cells take up naked DNA, and these in vivo transfected cells express the foreign genes that signal in vivo synthesis of antigenic proteins in a manner identical to that occurring in natural infection.6,16 Consequently, DNA vaccines appear to stimulate both humoral and cellular immunity. DNA vaccines offer many of the potential benefits of live vaccines without the same inherent risks, such as reversion to virulence. Because the vaccine consists of a single gene, it will not induce infection or disease. In addition, DNA vaccines typically induce expression of only one or a few of the many protein antigens present in the parent microorganism, thereby raising the possibility of developing companion diagnostic tests to differentiate vaccinated animals from animals that are carriers of the disease. Another advantage of having the host respond to a single protein rather than the myriad of proteins present in a pathogen is that many pathogens have proteins that can downregulate the immune response to the desired protein. Further, DNA vaccines are typically able to overcome maternally derived immunity in neonates or in very young animals.6
To date, no adverse effects have been associated with the use of DNA vaccines; however, safety concerns, including the potential integration of plasmid DNA into the host genome or the generation of anti-DNA antibodies, have been determined to be of remote risk. DNA vaccines stimulate a relatively weak serologic response. This response can be improved by the co-expression of the antigen along with cytokines, the administration of the DNA-containing plasmid through needleless devices, and the use of adjuvants. Investigations of the use of equine DNA vaccines are at an early stage, but already it has been demonstrated that they are effective at protecting horses from influenza virus and WNV infection.17,18 Recently, an adjuvanted DNA WNV vaccine was licensed by the U.S. Department of Agriculture (USDA) for use in horses and represents the first DNA vaccine licensed for use in any species.19 As of August 2006, this vaccine has not been marketed for use in the horse.
Marker Vaccines
Marker (or DIVA) vaccines are needed in situations where the differentiation between a naturally infected and a vaccinated animal is important. Such vaccines can be subunit, gene-deleted, vectored, or DNA vaccines and are always used in conjunction with a diagnostic test. The basis of marker vaccines is that vaccinated animals do not induce antibodies to a marker protein that is absent from the vaccine, but included in a diagnostic test. No marker vaccines are yet available for use in equids, but such vaccines are being developed against equine arteritis virus.20 This approach will permit control by serologic testing and exclusion to continue in parallel with vaccination to prevent acquisition of infection, and it may greatly facilitate international movement of horses as well as ultimate eradication of this disease from horse populations in individual countries, should industry and regulatory authorities deem this approach to be desirable.
Adjuvants
Mechanisms of Action
The mechanisms of action of most adjuvants remain poorly understood because immunization often activates a complex cascade of responses, and the primary effect of the adjuvant is difficult to discern clearly. In general, adjuvants appear to exert their effect by enhancing antigen presentation, improving antigen stability, or acting as immunomodulators.21,22 A single adjuvant may have more than one mechanism of action. Adjuvants that influence antigen presentation can affect this complex process by improving antigen uptake by antigen-presenting cells (APCs), the cells that process antigens and present epitopes to T cells in association with major histocompatibility complex (MHC) molecules. Some adjuvants appear to trap the antigen at the injection site and provide a continuing supply to local APCs, whereas others may work by saturating Kupffer cells in the liver and subsequently may increase the amount of antigen reaching the APCs. Most adjuvants can effectively stimulate T-helper (Th) cells and humoral immunity (Table 70-2). Some, such as liposomes, also appear to deliver antigens to pathways that lead to the presentation of MHC class I molecules and the induction of a CTL response. Immunomodulation is another mechanism of action of adjuvants and is achieved by altering the cytokine network. Adjuvants can influence the type of immunity by enhancing some cytokines and reducing the concentration of others, which may shift the immune response toward a T-helper cell type 1 (Th1, cell-mediated) or T-helper cell type 2 (Th2, humoral) response. By shifting the balance of cytokines, adjuvants such as saponins may stimulate cell-mediated immunity to an antigen that would normally induce only antibodies.
Adverse Effects
In addition to enhancing the immune response, adjuvants can also increase the adverse effects of the vaccine. Adverse effects are influenced by the interactions of the specific adjuvant and antigen.23 Systemic, nonspecific adverse effects can include lethargy, anorexia, fever, arthritis, uveitis, and soreness. More often, however, adjuvants cause local reactions, including inflammation and, more rarely, granulomatous or sterile abscesses. Although most of these reactions are minor and transient, severe inflammation can trap antigens at the injection site and prevent them from being recognized by the immune system. Further, local inflammation and granulomas after vaccination have been linked to the development of vaccine-associated tumors in small animals. Such association has not been reported for horses. Adverse reactions should be reported to the vaccine’s manufacturer or the USDA (1-800-752-6255).
Licensing and Safety of Vaccines
The USDA Animal and Plant Health Inspection Service (APHIS) is the federal agency charged with licensing and overseeing the production and use of veterinary vaccines and other biologic products marketed in the United States. The Canadian Department of Agriculture has similar authority in Canada. These agencies license the facilities in which vaccines are produced and regularly inspect them to ensure that facilities and production methods meet established standards. All vaccines are checked for potency, stability, and safety before licensing and at regular intervals thereafter. Safety is established by testing for sterility, toxicity, freedom from extraneous organisms and other material (i.e., purity), and confirmation of the identity of the antigens or organism(s) included in the vaccine. Both “in-house” and field safety studies involving several hundred animals (typically >500 in the case of horses) are also completed before final licensing to confirm that the risk of inducing local or systemic adverse reactions is at an acceptably low level.
The USDA has traditionally placed more emphasis on documentation of the purity, stability, and safety of veterinary vaccines than on their efficacy.24,25 Consequently, vaccines for use in horses are typically safe when stored, handled, and administered according to label directions. Many vaccines, however, particularly those directed at pathogens of the respiratory and gastrointestinal tracts, have been found to be of questionable efficacy in the field. Furthermore, published data documenting the efficacy of equine vaccines in well-controlled blinded challenge or field studies have been sparse until recently. For those vaccines for which challenge data were available, duration of immunity (DOI) was rarely established because challenge was typically performed a few weeks after completion of the primary series, when immunity would be expected to be maximal. Without data to define DOI, label directions for revaccination were often arbitrary or ambiguous. Similarly, efficacy studies were not typically performed on foals; therefore the potential inhibitory effects of maternally derived antibodies and the age at which to commence primary immunization were typically not established. Fortunately, “the bar has been raised” considerably during recent years, to the extent that solid challenge data, including information on DOI, are available for virtually all new equine vaccines granted full licenses during the last decade. It is hoped that this type of information will be generated in the future for vaccines that were first licensed many years ago.
If unacceptable reactions occur repeatedly, the need for continued annual or more frequent revaccination against individual antigens should be carefully reevaluated, taking into account risk of disease, balanced against the risk of an adverse reaction. Many of the horses that experience adverse reactions have received many doses of many vaccine antigens, repeated over many years. In these horses the vaccination protocol should be “pared down” so that only the most essential antigens are administered and the maximum possible interval between boosters is employed. For diseases such as rabies and tetanus for which resistance can reasonably be correlated with circulating antibody titer, one possible approach to define the maximum or optimal interval between booster doses would be to measure the antibody titer to define the need for revaccination. Unfortunately, this approach is currently limited by a paucity of laboratories that offer this type of testing on a routine basis, inexpensively, and with a short turnaround time. The introduction in recent years of commercially available enzyme-linked immunosorbent assay (ELISA) testing for antibodies to the SeM protein (Equine Biodiagnostics-Idexx, Lexington, Kentucky) and neutralizing antibody testing for WNV (Cornell University, Ithaca, New York, and EDART Laboratory, University of Florida, Gainesville, Fla) has made it possible to refine vaccination protocols for these diseases in horses that experience adverse reactions to vaccination. In addition, testing for antibodies to other pathogens may be available through state diagnostic laboratories.
Safety of Vaccines in Broodmares
Few vaccines carry specific label recommendations for use in pregnant mares, and little published data exist to document the safety of equine vaccines during pregnancy. Of the available fully licensed vaccines, the two EHV-1 vaccines (Pneumabort-K + 1b, Fort Dodge, and Prodigy, Intervet) marketed for use in pregnant mares as an aid to prevention of EHV-1 abortion, the vaccine marketed for prevention of type B botulism in foals (BotVax-B, Neogen), and the Calvenza line of influenza and EHV vaccines (Boehringer Ingelheim, St. Joseph, Missouri) include directions for use in pregnant mares. In addition, the conditionally licensed vaccine for prevention of rotavirus infection in foals (Equine Rotavirus Vaccine, Fort Dodge) is similarly labeled for use in pregnant mares. Although not specifically labeled for administration during pregnancy, widespread use in practice over many years has failed to document that any of the inactivated vaccines currently marketed for use in horses poses an unacceptable risk to pregnant mares. Therefore, pregnant mares are routinely vaccinated with inactivated vaccines directed against tetanus, eastern equine encephalomyelitis (EEE), western equine encephalomyelitis (WEE), WNV, influenza, EHV-4, strangles and to a lesser extent Potomac horse fever (PHF), rabies, and Venezuelan equine encephalomyelitis (VEE). Similarly, adverse impacts on pregnancy have not been documented for intranasally administered strangles and influenza MLVs or the parenteral EHV-1 MLV (Rhinomune, Pfizer). In addition, safety of the recombinant WNV vaccine (Recombitek, Merial) should not be a significant concern because the modified live canarypox vector lacks the ability to infect mammalian cells. In contrast, modified live-virus EVA and VEE vaccines and live–anthrax spore vaccines should not be used in pregnant mares. Protection of mares against the potential abortigenic effects of EVA infection is therefore best accomplished by completing the primary immunization series before the mare enters the broodmare band and by administering subsequent boosters during the open period before rebreeding.26
PASSIVE IMMUNIZATION
Use of Exogenous Antibodies
Several USDA-licensed tetanus antitoxin products have been available in North America for many years as adjuncts to the prevention of tetanus (see later section on tetanus) In recent years, two plasma products (Polymune R and Polymune REA, Plasvacc USA) have been granted full USDA licenses for prevention of Rhodococcus equi pneumonia. A third product (Rhodococcus equi Antibody, Lake Immunogenics) has been granted a conditional license pending acceptance by USDA of results of a published study documenting a high level of efficacy in preventing R. equi pneumonia on farms where the disease is endemic. Efficacy of these products is optimized if one dose is administered during the first week of life and a second dose is administered 3 to 6 weeks later.27 Other USDA-licensed plasma products include Polymune-J (Plasvacc USA) harvested from donors immunized against the J5 core antigen of E. coli. This product has documented efficacy as an adjunct to treatment of horses with endotoxemia and colitis and is also indicated for the treatment of foals with gram-negative sepsis.28 Polymune-B (Plasvacc USA) is a Clostridium botulinum type B antitoxin licensed by the USDA for prophylactic administration to horses experiencing likely exposure to C. botulinum type B toxin or spores. This product has also been used in treatment protocols to neutralize type B toxin in foals with shaker foal syndrome and horses of all ages suspected of experiencing exposure. This univalent type B antitoxin will not neutralize other toxin types. One conditionally licensed plasma product, Streptococcus equi Antibody, Equine Origin (Mg Biologics), is marketed for use in horses that are clinically ill from infection with S. equi subsp. equi and claims to reduce duration of signs, severity of disease, and mortality. Another potential indication would be prevention of disease in horses exposed to S. equi subsp. equi, although published data regarding efficacy of this product are not available at this time. Similarly, one conditionally licensed plasma product, West Nile Virus Antibody, Equine Origin (Lake Immunogenics), is currently marketed as an aid in the treatment and control of WNV infection. Efficacy is supported by data showing reduction in the level of viremia experienced by hamsters challenged either 1 day before or 1 day after administration of plasma. Evidence of efficacy of this product, and two similar products manufactured by Plasvacc USA and Mg Biologics, in horses has not yet been documented in either experimental or field studies.
Passive Transfer of Maternal Immunity
Passive transfer of maternal antibodies from the mare to the foal through colostrum constitutes by far the most important form of passive immunization in horses. The epitheliochorial placentation present in the mare prevents transfer of maternal immunoglobulins to the fetus during gestation, rendering the foal essentially agammaglobulinemic at birth. Therefore, protection of the foal against specific infectious diseases that it is likely to encounter during the first few months of life, as its own immune system matures, relies heavily on postnatal absorption of specific antibodies and perhaps other factors that the dam has concentrated in colostrum during late gestation. The duration of protection afforded by maternally derived antibodies (MDAs) depends on many factors, the most important of which are the characteristics of the specific infectious agent, the challenge dose, and the magnitude of the postnursing titer of specific antibody. The latter is influenced by the dam’s history of disease exposure and vaccination, age, parity, normalcy of gestation, and prepartum leakage of colostrum (i.e., factors that influence concentration of specific antibody in colostrum). Above all, interval between foaling and nursing, the volume of colostrum ingested, and ingestion of macromolecules other than colostrum before ingesting colostrum play major roles in determining passive transfer of specific MDAs. Although several immunoglobulin isotypes are present in colostrum, the subisotypes of IgG are absorbed into the systemic circulation of the foal in much higher concentration than either IgM or IgA.29 Specific IgA, however, is secreted continuously in milk and may provide passive protection against pathogens such as S. equi and enteric organisms by helping coat the pharyngeal and intestinal mucosa, thereby neutralizing pathogens.29,30 When foals ingest adequate amounts of high-quality colostrum during the first 12 to 24 hours after birth, titers of specific antibody in the serum of the foal are typically very similar to the serum titer in the dam at the time of foaling. Whereas the rate of decay of specific subisotypes of IgG varies to some extent, the overall half-life of decline of maternal IgG antibodies is typically between 25 and 40 days.31–37 Thus the magnitude of the postnursing antibody titer and the sensitivity of the assay used to detect passively transferred antibodies will determine persistence of these antibodies at measurable levels in the serum of foals. Use of ELISA and other sensitive assays has made it possible to detect persistence of MDAs at detectable levels for 6 months or longer in some cases.32,37
It is widely assumed that pregnant mares are fully capable of mounting appropriate cellular and humoral immune responses to vaccines; however, this issue has received little research attention. Whereas mares that have been primed before breeding appear to mount appropriate anamnestic responses to vaccines administered during late gestation, it appears that the humoral response to primary vaccination with at least the inactivated WNV vaccine is downregulated during gestation. In one study, more than 75% of naive mares, vaccinated for the first time against WNV while pregnant, failed to mount a detectable serologic response to two doses of an inactivated WNV vaccine administered 4 weeks apart.38 Consequently, their foals failed to acquire any WNV antibodies through colostrum and were rendered potentially susceptible to infection. At this time it is not known whether this apparent pregnancy-associated suppression of serologic responses to primary immunization applies to vaccines other than the inactivated WNV vaccine. Nevertheless, it is wise to complete primary immunization before breeding and administer booster doses of selected antigens late in gestation rather than attempting to complete the primary series during gestation, regardless of the vaccine. The common practice of administering booster doses of multiple antigens to mares during late gestation raises the possibility that “competition” between multiple antigens will compromise the response to some or all of them and increase the risk of an adverse local or systemic reaction. Although these issues have not been addressed in controlled research studies, it is nevertheless good practice to administer no more than four antigens at one time and to allow an interval of 3 to 4 weeks between administration of vaccines containing additional antigens.
VACCINATION OF FOALS AND INFLUENCE OF MATERNAL ANTIBODIES ON VACCINE RESPONSES
In addition to passively protecting the foal, maternal antibodies have been shown to exert a profound inhibitory effect on the immune response of foals to antigens, including those contained in vaccines. Several studies reported by groups in Holland, Ireland, and the United States during the 1990s brought this issue into focus by demonstrating that foals less than 6 months of age consistently failed to mount serologic responses to inactivated influenza vaccines.33,35,37,39–42 Of potentially greater concern was the finding that not only a high proportion of foals vaccinated under the cover of MDAs failed to seroconvert in response to the recommended primary series of two or three doses of influenza vaccine, but also many failed to respond to multiple additional doses administered during the next year, suggesting induction of a potentially detrimental “immunotolerance-like” phenomenon.39,40,43 Our studies confirmed an apparent lack of response of foals to multiple doses of inactivated influenza vaccines when the hemagglutination inhibition (HI) test was used to detect serologic responses. When the same samples were retested using sensitive isotype-specific ELISA tests, it was found that 6-month-old foals did mount a response that included all IgG subisotypes but that was less vigorous for the more important virus neutralizing IgGa and IgGb subisotypes than for the less effective IgG(T) subisotype.37 Whereas there appeared to be some differences in responses to different vaccines containing different adjuvants, this “misdirection” of isotype responses in favor of IgG(T), likely influenced by MDAs, was consistently observed.37 Subsequent studies, in which titers of total, rather than antigen-specific, IgG subisotypes were determined, documented that the age-related increase in concentrations of IgGb lagged significantly behind increases in concentrations of other isotypes and remained below adult levels beyond 6 months of age.44
Maternal antibody interference has now been documented as a significant issue for many other antigens, including tetanus, EEE, WEE, EHV-1, and EHV-4, contained in vaccines administered to foals.36,37,45–48 Even low levels of antibody, below those detectable by many routine serologic tests and below those thought to be protective, can completely block the serologic response to some vaccines, resulting in a potentially prolonged period of susceptibility before the foal is capable of responding appropriately to vaccines.47 These findings also indicate that it is not typically feasible to test samples from foals serologically to predict whether they will respond to particular vaccines. We now recommend that primary immunization with most vaccines containing inactivated antigens should be delayed until foals are 6 months of age or older and, with the exception of rabies vaccine, three doses of vaccine should be included in the primary series rather the two doses routinely recommended by vaccine manufacturers. Typically, the third dose stimulates a serologic response of greater magnitude and durability than two doses and may also contribute to a higher “set point” for the response to subsequent booster doses.37,38,47,49 In contrast to the results previously cited, MDAs do not appear to exert a marked inhibitory effect on the response of foals to either the inactivated or recombinant live WNV vaccines (West Nile-Innovator, Recombitek), thus permitting antibody-positive foals as young as 3 months of age to be immunized successfully.38
Assessing risk takes into account both the incidence of disease (i.e. the likelihood that the foal will become infected) and the risk of serious sequelae or death if the horse does become infected. If the disease affects the foal early in life, as with rotavirus infection, there is usually insufficient time to induce a protective immune response by actively immunizing the foal. Under these circumstances, the approach should be to maximize the degree of protection passively transferred from the dam through colostrum. Other diseases, such as rabies, affect horses of all ages, but the risk of acquiring infection is generally low.
VACCINATION RECOMMENDATIONS FOR SPECIFIC DISEASES
Tetanus
All horses are at risk for developing tetanus, an often-fatal disease caused by a potent neurotoxin elaborated by the anaerobic, spore-forming bacterium Clostridium tetani. These organisms are present in the intestinal tract and feces of horses, other animals, and humans and are ubiquitous in soil. Tetanus is expensive to treat and has a high mortality rate; therefore all horses should be actively immunized using tetanus toxoid as part of the core vaccination program. Active immunization reduces the need to administer tetanus antitoxin, the use of which is associated with risk of inducing potentially fatal serum hepatitis. Protection against tetanus appears to be mediated by circulating antibodies, and these antibodies are transferred efficiently through colostrum. The many available vaccines are typically formalin-inactivated, adjuvanted toxoids that are inexpensive and safe and that induce an excellent serologic response and solid, long-lasting immunity. Manufacturers recommend administration of a primary series of two doses of toxoid at 3- to 6-week intervals, followed by annual boosters. Titers of specific antibody increase within 14 days after administration of the second dose in the primary series and, in adult horses, persist at detectable levels for 12 months or longer, depending on the adjuvant system used in the vaccine.50,51
No published challenge studies are available to document the speed of onset or duration of protection induced by tetanus toxoid preparations currently licensed in North America; conclusions regarding their efficacy are therefore based on the serologic response obtained in laboratory animals. However, a challenge study conducted in Europe more than 40 years ago found that horses were resistant to challenge 8 days after receiving a single injection of tetanus toxoid, before antibody could be detected in their serum.52 A second study demonstrated that a series of three doses of tetanus toxoid induced protection lasting for at least 8 years, and perhaps for life, even when antibodies could no longer be detected.53 In contrast, tetanus has been documented in vaccinated horses in North America,54 although survival was strongly associated with previous vaccination. Thus it would not be prudent to recommend extension of the annual interval for revaccination with tetanus toxoid, pending publication of data documenting duration of immunity. Vaccinated horses that sustain a wound or have surgery more than 6 months after receiving their previous tetanus booster should be revaccinated with tetanus toxoid.
The annual booster for pregnant mares should be administered 4 to 8 weeks before foaling to protect the mare if she sustains foaling-induced trauma and to enhance concentrations of specific immunoglobulins in colostrum. Colostrum-derived antibodies significantly interfere with the immune response of foals vaccinated with tetanus toxoid until they are about 6 months of age.37,51 If a foal received appropriate transfer of colostral antibodies from a vaccinated mare, that foal should receive its primary series of three doses of tetanus toxoid beginning at age 6 months or older. Foals born to nonvaccinated mares should receive this initial three-dose series starting at 1 to 4 months of age. The three-dose primary series is recommended for foals because a high proportion of foals fail to seroconvert in response to two doses of tetanus toxoid, regardless of whether maternal antibodies are detectable at administration of the first dose.37,51 Optimally, the third dose in the primary series should be administered 2 to 3 months after the second dose.
Tetanus antitoxin is produced by hyperimmunization of donor horses with tetanus toxoid. Administration of one vial of antitoxin (1500 IU) to nonvaccinated horses induces immediate passive protection that lasts no longer than 3 weeks.51 More prolonged protection may be accomplished with higher doses. In addition to the use of high doses of tetanus antitoxin to treat tetanus, indications frequently cited include administration to newborn foals born to nonvaccinated mares and to nonvaccinated horses that sustain an injury. In these cases the concurrent administration of tetanus antitoxin and tetanus toxoid at different sites using separate syringes has been advocated, followed by administration of additional doses of toxoid at 4- to 6-week intervals to complete the primary series.55 Because a small but significant number of horses experience serum sickness and fatal hepatic failure (serum hepatitis) several weeks after receiving tetanus antitoxin,56,57 a preferred approach to the nonvaccinated horse that sustains a puncture or deep laceration is to thoroughly clean and debride the wound, initiate active immunization by administering tetanus toxoid, and institute a course of antimicrobial treatment with penicillin or an alternate antimicrobial that is active against C. tetani.
Equine Encephalomyelitis (Sleeping Sickness)
Although correlates for protection against EEE, WEE, and VEE are not well established, circulating antibodies are assumed to be important because infection is acquired by vascular injection (mosquito bites), and current inactivated vaccines are protective.58,59 However, despite that virtually every manufacturer of equine vaccines in North America produces one or more equine encephalomyelitis vaccines, no randomized, double-blind challenge trials using these vaccines have been published. Available vaccines are inactivated, adjuvanted, bivalent whole-virus products containing EEE and WEE (Encevac with Havlogen, Intervet; Encephaloid Innovator, Fort Dodge; Cephalovac EW, Boehringer Ingelheim) or trivalent products that also contain VEE (Cephalovac VEW, Boehringer Ingelheim). Veterinarians and horse owners often use combination products containing other antigens, such as tetanus, influenza, WNV, or equine herpesviruses for primary or booster immunization of horses against encephalomyelitis viruses.
Primary immunization of nonvaccinated adult horses is accomplished by administration of two doses of inactivated vaccine 3 to 6 weeks apart. In areas where EEE is not a threat and mosquito vectors are active for less than 6 months of the year, annual revaccination in the spring, before the peak insect vector season, is recommended. In areas such as the Gulf States where EEE is endemic and mosquitoes are active virtually year-round, most veterinarians prefer to revaccinate horses semiannually to ensure more uniform protection throughout the year. Inactivated encephalomyelitis vaccines are considered to be safe for use in pregnant mares; therefore, booster vaccination 4 to 8 weeks before foaling is routinely recommended to enhance colostral concentrations of specific immunoglobulins. Neutralizing antibodies to WEE and EEE are transferred passively to foals through colostrum and decline with an estimated half-life of 33 and 20 days, respectively. Maternally derived antibodies (MDAs) appear to confer protection and are detectable in the serum of many foals from vaccinated mares for at least 3 months and up to 7 months, depending on the postnursing titer.31,36,60,61
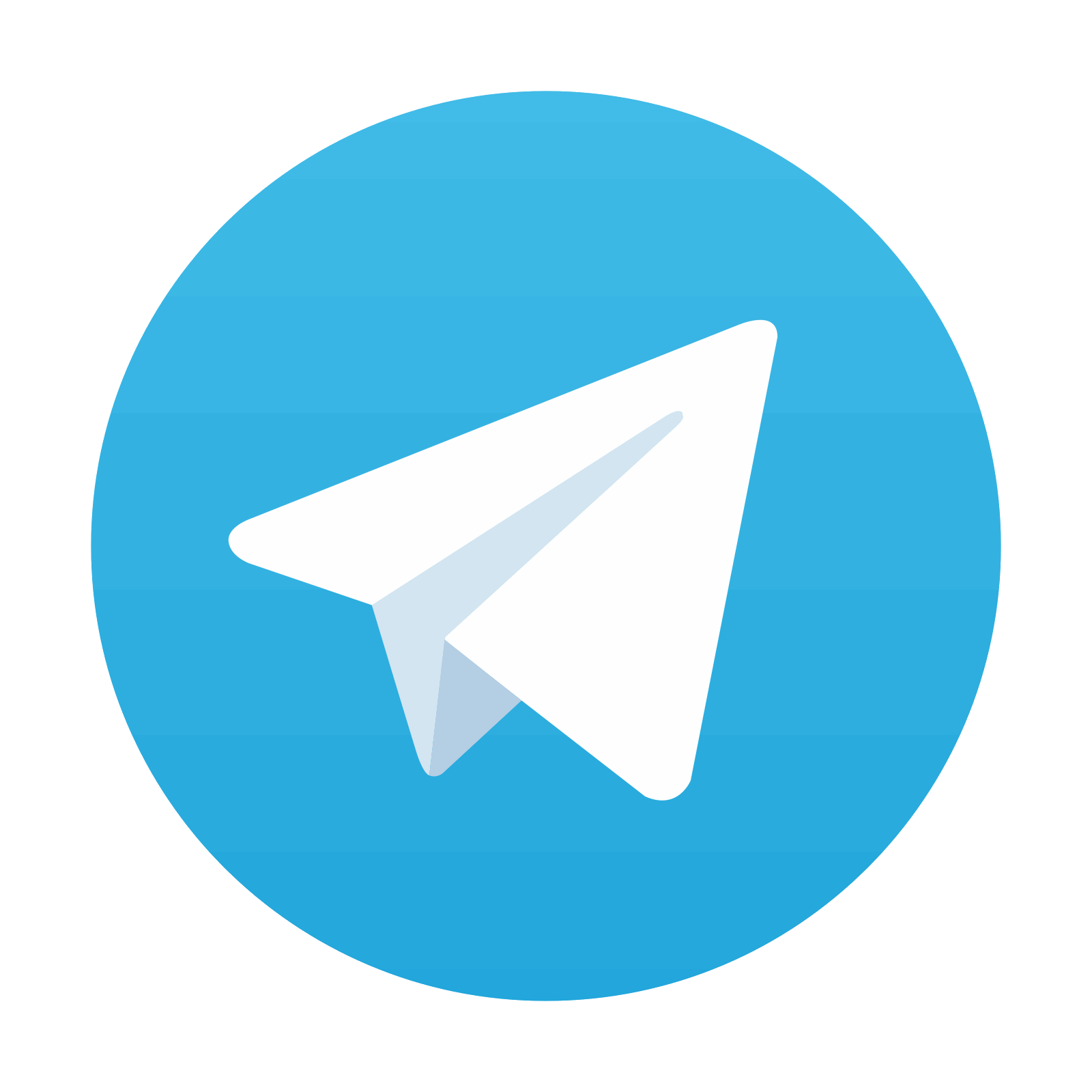
Stay updated, free articles. Join our Telegram channel

Full access? Get Clinical Tree
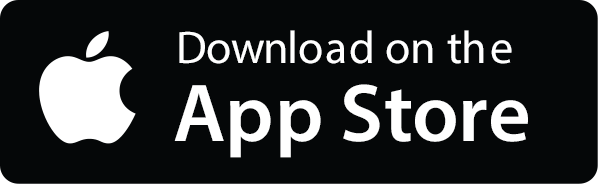
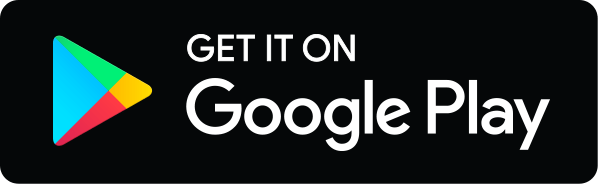