Fig. 1.
The immune system activation following intracerebral hemorrhage. (a) Activation of innate immune system mechanisms Extravasated erythrocytes and extracellular serine proteases (thrombin, tissue plasminogen activator, cathepsins) immediately activate an antigen presenting cell (APC, macroglia-macrophage cell line and dendritic cells). As the major source of cytokines and chemokines, APC induce chemotaxis and trigger inflammation by activation of cell signaling cascades. Leukocytes (polymorphonuclear neutrophils and T-lymphocytes), attracted by leukotrienes, interleukins, and lymphokines, infiltrate the brain through a disrupted brain–blood barrier (BBB) and promote early brain injury while stimulating macrophages to release pro-inflammatory mediators including reactive oxygen species (ROS) and secretion of pro-inflammatory proteases. The activated complement system components mediate erythrocyte lysis and brain edema formation. Natural killer cells (NK) produce cytokines and release cytolytic/cell killing molecules and, similar to gamma delta T-cells, are considered a bridge between innate and adaptive immune systems. Also, the complement system and phago -cytic activation and release of growth factors are important for hematoma clearance and post-hemorrhage brain repair. (b) Activation of adaptive immune system mechanisms In experimental ICH T-lymphocyte accumulation occurs 2–3 days post injury and lasts for at least 28 days. A sub-population of T cells (T-cytotoxic or T-helper) are activated by signaling events, when the T-cell receptor (TCR ) and CD 8 or CD4 coreceptors bind antigen-specific class I or II MHC (MHC-I or MHC-II) molecules expressed by the target cells. T-regulatory cells function in ending the T cell-mediated immunity activation i.e. immune tolerance. Currently, the role of gamma delta cells as well as a memory T cells in ICH brain injury is not clear.
The innate immune response to ICH injury is non-specific and immediate. It represents mechanisms of (a) inflammation, i.e. release of cytokines, chemokines, and leukotrienes which locally activate microglia and attract migration of neutrophils through a disrupted blood–brain barrier, and (b) a complement cascade in which lysis or opsonization and killing of pathogens activates via lectin and alternative pathways (4–6). Further, adaptive immune mechanisms and complement system (classical pathway, immunoglobulin-mediated), triggered by activated antigen-presenting cells (APC: monocyte–monophage cell lineage and dendritic cells) and heme catabolism products, all aggravate an initial impact contributing to secondary ICH-injury development (7, 8).
Current concepts outline a two-step immune system impact: initially, it exacerbates ICH-injury progression, while at later time points it appears to be involved in brain tissue repair and is beneficial to post-ICH brain remodeling (2, 3, 8, 9). The inflammation and complement cascade improves post-stroke neuroregeneration via release of the growth factors and clearance of cellular debris (2). Adaptive immunity via bystander mechanisms are involved in maintenance of administrated stem cell activity in experimental ICH (10, 11).
Since the immune system is extensively involved in ICH injury progression and recovery, it is a potential therapeutic target. It is worthy to note that under experimental settings, the method of hemorrhage induction and species choice alters the ability to detect the level and time course of immune system markers (12). Comparison of collagenase and blood injection ICH models demonstrates accelerated cytokine release (tumor necrosis factor-alpha, TNF-alpha), delayed expression of matrix metalloproteinase (MMP)-related genes, and cytotoxic T-cell markers (CD8-alpha) in collagenase-induced ICH, while expression of microglia/macrophage markers does not differ between these two models (4, 8, 13, 14) (Table 1).
Dependent on the research focus, different tissues can be obtained and studied. Numerous methods such as immunohistochemistry (IHC), histology, Western blot (WB), and polymerase chain reaction (PCR) can be performed on the fixed and stored tissues, while others (flow cytometry (FC), blood cell counting) require an immediate processing of the freshly harvested tissue samples. To avoid technical artifacts, special care should be taken during sample collection as immune cells are highly sensitive to tissue degradation and can be easyily activated.
The assessment techniques differ in their sensitivity and are reflective of the time course of immune response to ICH brain injury (Table 1). For instance, cytokine-related gene expression precedes expression of cytokine proteins as detected by immunohistochemistry in paraffin-embedded brain sections (IP, Table 1). While IP identified the peak of interleukin 6 (IL-6) and TNF-alpha activity at 2 days following intracerebral blood injection, it was detected at 6 h post-ICH using messenger ribonucleic acid assay (mRNA, Table 1) (8, 13). In addition, the peak cytokine protein levels, revealed by enzyme-linked immunosorbent assay (ELISA), appeared to precede mRNA detection (interleukin 1 beta (IL-1 beta), Table 1). There are no clear visible differences between Western Blot and immunohistochemistry (using Avidin Biotin Complex (ABC) method) sensitivity in detection of activated complement cascade markers (3, 6). In contrast, the sensitivity of FC for detecting ICH brain injury is not clear. At days 1 and 4, post-ICH no CD8-alpha positive cells in animal brains were identified using FC (4). In a similar animal model of ICH, but using IP, the number of CD8-alpha positive cells was increased at 2–3 days and remained detectable for 28 days after ICH (14) (Table 1). These differences could be related to time of assessment or immunophenotyping. Also, other methods such as volumetric cytometry or magnetic microspheres (beads) cell sorting can be more efficient. In regards to method sensitivity, the new multi-analyte profiling beads (xMAP) technology (i.e. Luminex assays) looks promising. This technology combines proven platforms and assays—flow cytometry, fluorescent-dyed, microbeads, lasers, digital signal processing, and traditional chemistry. Luminex assays enable the detection and quantification of multiple ribonucleic acid (RNA) or protein targets simultaneously and provide data with concordance in ELISA and mass spectrometry.
Table 1
Time course of immune system response to experimental ICH brain injury in rodents
Cell types/markers/method | ICH model | 1 hr | 4 hr | 6hr | 1d | 2d | 3d | 4d | 7 d | 1 wk | 4 wks | References |
---|---|---|---|---|---|---|---|---|---|---|---|---|
INNATE IMMUNE MECHANISMS | ||||||||||||
Cellular | ||||||||||||
Neutrophyls (IP,IH) | B | – | – | + | +++ | ++ | – | – | ||||
CD45hiGR-1+ (FC) (neutrophils) | B | + | + | (4) | ||||||||
Microglia/macrophages (IF, IH) RCA+ | B | – | – | – | – | +++ | ++ | (12) | ||||
Microglia/macrophages (RCA+) | C | +++ | ++ | (12) | ||||||||
CD45loGR-1- (FC) | B | – | – | (4) | ||||||||
ICAM-1 (IP) | B | + | + | ++ | +++ | ++ | (3) | |||||
Humoral | ||||||||||||
Pro-inflammatory cytokines | ||||||||||||
IL-1beta mRNA ELISA | B B | + ++ + 3h | +++ ++ 8h | ++ ++ | + | + | (9, 13) | |||||
IL-6 mRNA IP | B B | +++ + | ++ + | +++ | + ++ | + | (3, 13) | |||||
TACE (mRNA) | B | + | ++ | ++ | +++ | (13) | ||||||
ICE (mRNA) | B | + | ++ | +++ | +++ | (13) | ||||||
TNF-alpha IP IP mRNA | B C B | + | + + 12 h +++ | ++ +++ +++ | +++ +++ | ++ + | + + | ++ | + 3wks | |||
Anti-inflammatory cytokines | ||||||||||||
IL-1ra (mRNA) | B | ++ | ++ | ++ | + | (13) | ||||||
IL-10 (mRNA) | B | +++ | ++ | ++ | ++ | (13) | ||||||
TGF- beta | B | + | + | +++ | +++ | (13) | ||||||
VFGF (IP) | B | + | ++ | +++ | +++ | +++ | (13) | |||||
Matrix metalloprotease-related genes (mRNA) | ||||||||||||
MMP2 ↑ | C | – | – | +++ | – | (8) | ||||||
MMP3 ↑ | B C | – | – +++ | – | – | – – | – | (8, 13) | ||||
MMP7 ↑ | C | ++++ | – | + | – | (8) | ||||||
MMP9 ↑ | B C | – +++ | – | – | – | – +++ | – | (8, 13) | ||||
MMP12 ↑ | B C | – | + – | ++++ | – | ++ ++++ | – | (8, 13) | ||||
TIMP-1 ↑ | B C | + | ++ ++ | +++ | – | ++ +++ | – | (8, 13) | ||||
TIMP-2 ↓ | C | ++ | – | ++ | – | (8) | ||||||
TIMP-3 ↓ | C | – | – | ++ | – | (8) | ||||||
Complement system | ||||||||||||
C9 (IH (ABC); WB) | B | ++ | ++ | (6) | ||||||||
C3 (IF) | B | + | + | ++ | +++ | + | (3) | |||||
C3d (IH (ABC); WB) | B | + | +++ | (6) | ||||||||
Clusterin (IH (ABC); WB) | B | ++ | (6) | |||||||||
CR2 receptor for C3d (IF) | B | + | ++ | +++ | ++ | + | (3) | |||||
CR3 (mRNA) microglia activation | B | + | + | +++ | ++ | (13) | ||||||
ADAPTIVE CELLULAR IMMUNE RESPONSE | ||||||||||||
CD4+ (CD3+, CD45hi) FC | B | – | ++ | (4) | ||||||||
CD 8 alpha FC IP | B B | – | – | – | +++ | ++ | – | + | (4, 14) | |||
CD 8 alpha | C | – | – | – | – | +++ | +++ | ++ | + | + | (12) | |
NF kappa B (IP) | B | + | ++ | +++ | ++ | + | (3) | |||||
Apoptosis | ||||||||||||
TUNEL+ cell counts Apoptag in situ kit | B | + | + | ++ | +++ | ++ | ++ | + | + | + | (12) | |
TUNEL + cell counts Apoptag in situ kit | C | + | + | ++ | ++ | +++ | +++ | +++ | ++ | + | (12) | |
Astrocytosis | ||||||||||||
GFAP (mRNA) | B | + | ++ | +++ | +++ | (13) |
Each method of immune system assessment has its advantages and disadvantages. ELISA quantification is rapid and highly sensitive, but is an expensive method as it recognizes only one specific binding site (epitope) and requires specific monoclonal antibodies. Western Blotting is technically demanding and also expensive. It is subject to interpretation (presence or absence of bands; intensity of those bands) but remains the gold standard quantitative technique to validate immunohistochemistry. Immunohistochemistry has the tremendous advantage of being able to localize given proteins within the tissue examined at relatively low cost. Its major disadvantage is that, unlike immunoblotting techniques where staining is checked against a molecular weight ladder, it is impossible to show in immunohistochemistry that the staining corresponds with the protein of interest. Flow cytometry allows high-speed identification and sorting of individual cells by their size, structure, or specific identifiers such as cell receptors based on optical signal (fluorescence-activated and volumetric methods) (15). The clear advantage of FC over other technologies is that a large number of parameters can be analyzed on each and every cell. However, the disadvantage is that flow cytometry, even with high-speed systems, is very much slower than automated image-processing systems (16). While FC is a valuable technique for blood cell sorting, it is a subject of interpretation for testing cells within parenchymatous tissue; depending on the method of cell collection the cell membranes may be damaged and results can be misinterpreted. In addition, there are some common problems which fluorescent immunophenotyping share between all fluorescent-based assessment techniques. When the primary antibody used to detect an antigen on the blot is labeled with an enzyme or fluorescent dye (i.e. direct detection method), its immunoreactivity can be altered which results in reduced signal amplification. When a primary antibody is first bound to the antigen and then followed by a labeled secondary antibody that is directed against the primary antibody (indirect detection method), the secondary antibody does not affect primary antibody immunoreactivity and strengthens the fluorescent signal. A disadvantage of the indirect method is that the secondary antibodies may produce nonspecific staining and result in cross-reactivity which is avoided by labeling with primary antibodies. However, labeled primary antibodies are more expensive and are not as readily available compared to the variety of labeled secondary antibodies. Typically, two methods, both quantitative and qualitative, should to be used to ensure assessment of the final results.
Fluorescent phenotyping allows monitoring of the local cellular immune response by visualizing lymphocytes: their abundance, morphology and types, as well as the dynamics of spatial and temporal distribution. The number of T-lymphocytes and their subsets in the brain can be used to evaluate the progress of immune response to ICH. T-cell subsets can be defined by recognition of cluster of differentiation (CD) molecule proteins on the lymphocyte surface (15, 17) (Table 2). There are three CD molecules which are commonly used as markers to identify T-lympocyte subpopulations. CD3 is specific for all T-lymphocytes and is the basis of its segregation from B-lymphocytes. CD3 is a multisubunit complex of proteins that associates directly with the T-cell antigen receptor (TCR, heterodimer composed of either alpha, beta or gamma and delta chains) (18, 19). CD3 is composed of five invariant polypeptide chains that associate to form three dimers: a heterodimer of gamma and epsilon chains, a heterodimer of delta and epsilon chains, and a heterodimer of two zeta chains or zeta and eta chains. CD8, a cell-surface glycoprotein, is a two-chain complex (alpha–alpha or alpha–beta) receptor that binds class I major histocompatibility complex (MHC) molecules presented by the antigen-presenting cells (15, 18). A primary function of CD8 is to facilitate antigen recognition by TCR and to strengthen the affinity of TCR-antigen interactions. CD4 is a membrane glycoprotein that contains four extracellular immunoglobulin-like domains. CD4 increases the avidity of interaction between the TCR and antigen II MHC molecules presented by APC (20). A combination of positive expression of CD3 (CD3+) and CD4 (CD4+) or CD8 (CD8+) cells indicates helper or cytotoxic T cells, respectively (15, 21) (Table 2). Some other leukocytes also express these CD molecules in different combinations: macrophages express low levels of CD4; dendritic cells express high levels of CD8 and monocytes express both CD4 and CD8 proteins (15, 22) (Table 2). In addition, CD3 markers can label Purkinje cells and cytoplasm of plasma cells and macrophages, while CD4 proteins are expressed on granulocyte surfaces (17).
To avoid confusion between T-lymphocyte identity as cytotoxic and helper T-lymphocyte subsets, double immunostaining of CD3/CD8 or CD3/CD4 is more appropriate while single CD4 or CD8 labeling is not unique for only these cell types (Table 2).
The advantage of multiple immunostaining is its ability to characterize the location of specific cellular components by virtue of the degree of overlap between multiple fluorescent labels where each has a separate emission wavelength (i.e. colocalization analysis). However, the immunohistochemical results can be affected by autofluorescence when immunolabeled tissue is excited with light of short wavelengths (23). Autofluorescence is the natural property of living cells (see Fig. 2). Endogenous fluorophores (fibronectin, lipofuscin, and elastin) naturally emit light which increase with aging and/or disease (24, 25). When older animals are used for ICH research, autofluorescence can pose a serious problem. Several solutions can help to solve this problem: (a) using thinner sections if possible; (b) chemically suppressing the autofluorescence signal prior to blocking for protein (c) utilizing technical advantages of imaging software: filters, background correction, modulation of exposure time, etc. (for details see ref. 29) (d) using an infrared scanner which emits light with a high wavelength (700–800 nm) thus eliminating autofluorescence. Immunohistochemistry with infrared immunolabeling allows analysis of the spatial T-cytotoxic and T-helper cell in ICH-injured brain tissue and is suitable for quantitative studies.
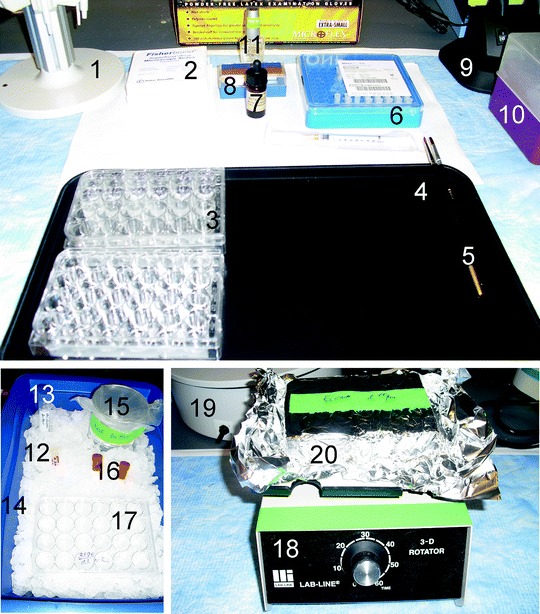
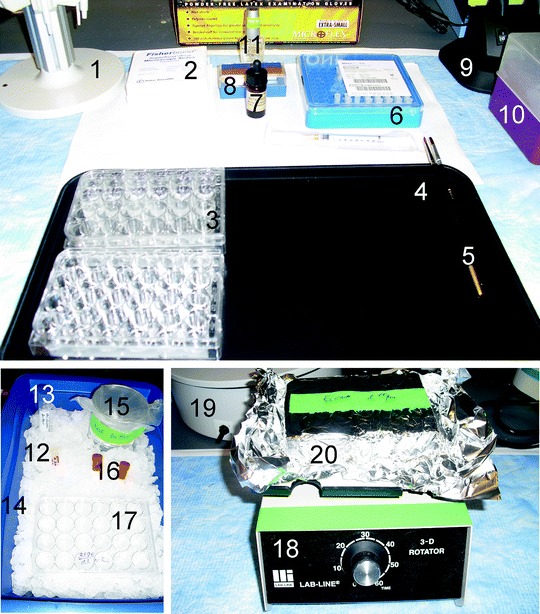
Fig. 2.
Equipment required for free-floating sections immunohistochemistry. (1) Pipettor stand and (2) Glass slides. There are no special brand preferences. However, slides should be compatible for use in fluorescent microscopy. For example, VWR Micro slides Superfrost Plus, VWR cat. No 48311-703, (3) Plates for tissue wash and incubation. Multiple Well Cluster Plate, 24 Well, Tissue Culture-Treated, Corning Costar #3526, (4) Black tray helps visualize slides while washing and transfer between plates. (5) Paint brushes to pick up tissue sections, (6) Syringe filters Millex-FG, 0.20 μm, hydrophobic PTFE, 4 mm, cat. #SLFGR04NL, (7) Vectashield mounting media. If mounting media is not a hard set, a small amount of nail polish can be applied on the cover slip edges to prevent its slipping. (8) Cover slips. It is critical to ensure that cover slips can be used for fluorescence. As example, Fisher scientific, Fisherfinest Premium cover glass, #12548B, (9) Gooseneck lamp, (10) Sterile tips, (11) Clear nail polish, (12) Serum. To avoid multiple freezing–thawing cycles, serum can be aliquoted into 2-ml tubes and saved at −20°C until usage, (13) Incubator buffer. All tubes and tips require sterilization to prevent contamination. (14) Ice container, (15) Wash buffer. During the procedure, all solutions and chemicals should be kept on ice, (16) Antibodies. Antibody conjugated with fluorescent dyes need to be light protected, (17) For negative control sections use a separate 24-well plate to avoid contamination, (18) Rotator. For overnight immunostaining, another rotator should to be placed into the refrigerator. (19) Water bath. Some antibody retrieval protocols require in water bath or microwave incubation. (20) During washing or incubation with antibodies containing a fluorescent dye, the plate should be covered with aluminum foil.
While protocols for the above discussed techniques are well established and published elsewhere (26–28), also see Subheading 2.11 below, the analysis of the brain tissue immunoreactivity by infrared systems is a relatively new approach. Its primary advantages are accuracy, simplicity, and low cost. In the detailed protocol described below, we demonstrate assessment of T-lymphocyte immunoreactivity following ICH. These data were extracted from 6-month-old Sprague-Dawley rats that underwent intracerebral collagenase injection to induce ICH. One brain section per animal at each time point was used to demonstrate the immunohistochemistry techniques. The two techniques presented here are immunofluorescence and infrared immunolabeling quantification. As the study of brain immunoreactivity following cerebral hemorrhage is the subject of intensive research, no clear timeline is suggested to identify T-cell activities. Existing information about the time course of immune system activation is summarized in Table 1.
< div class='tao-gold-member'>
Only gold members can continue reading. Log In or Register a > to continue
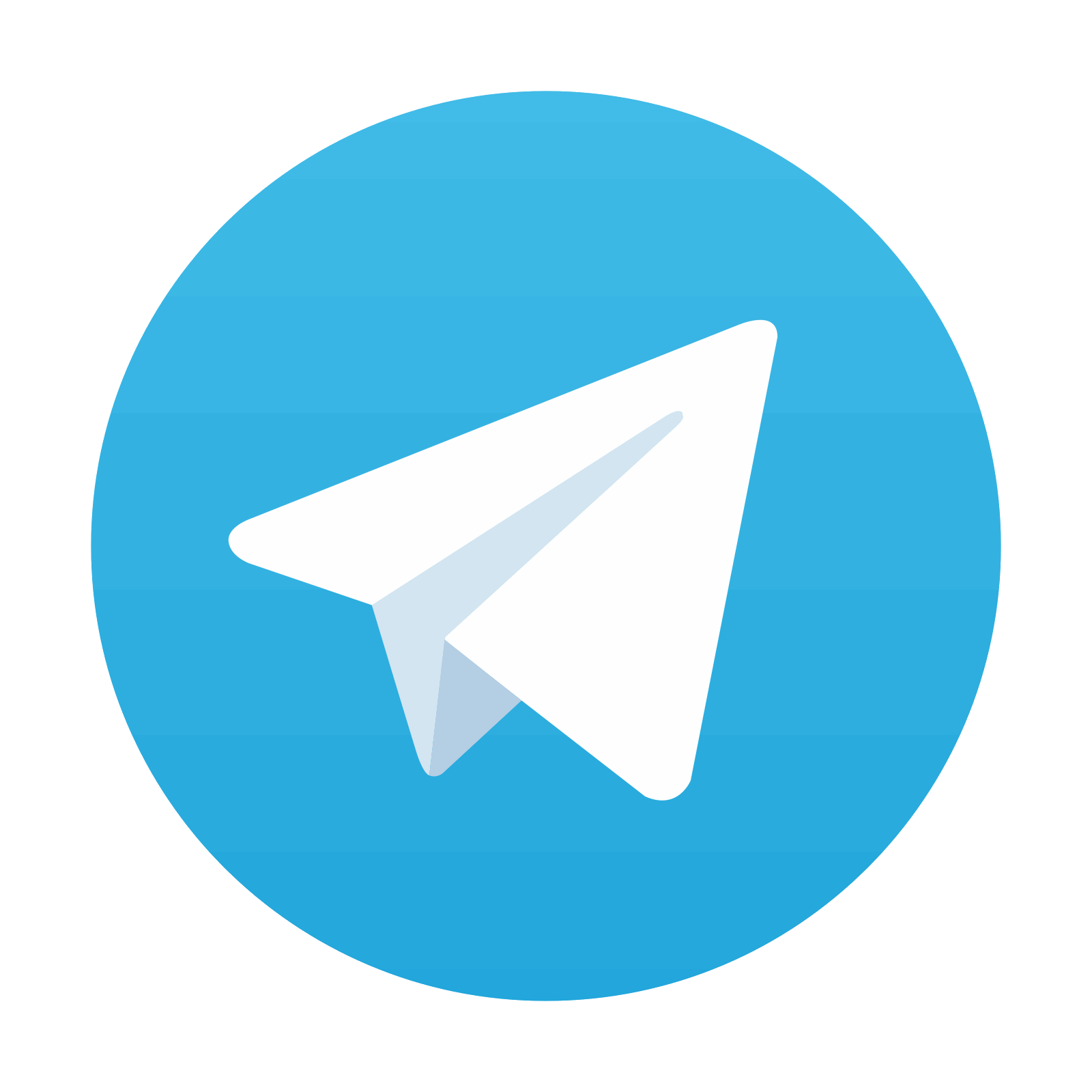