Introduction
Diagnostic imaging is an important component of the evaluation of injuries to the musculoskeletal system in the sports medicine and rehabilitation patient. Imaging techniques can be used to screen for areas of future concern and can help guide rehabilitation. For example, screening radiographs of a sound canine athlete might show mild degenerative changes in a joint. This information can help the sports medicine specialist and owner/handler determine an appropriate conditioning program to protect the joint from further damage, and to provide a baseline for long-term monitoring. This chapter reviews the basics of the most common and useful imaging modalities for canine sports medicine and rehabilitation.
Radiography
Radiography is the foundation of musculoskeletal imaging and is a basic component of a thorough musculoskeletal evaluation. Typical radiographic images include lateral and dorsal (dorsoventral, dorsopalmar/plantar, craniocaudal) projections. In most circumstances, these views will provide significant information. Subtle lesions in performance/working dogs may require additional views, including the dorsopalmar/plantar medial to lateral oblique (DPMLO) and dorsopalmar/plantar lateral to medial oblique (DPLMO). Oblique views will reveal surfaces obscured by superimposition on orthogonal views.
Additional views include the skyline or tangential view of the shoulder, used to visualize the intertubercular groove and to differentiate supraspinatus and biceps tendon mineralization (Flo & Middleton, 1990). Osteochondritis dissecans lesions on the lateral trochlear ridge of the talus are best viewed with flexed skyline and DMPLO images (Gielen et al., 2005).
Stressed views can provide critical information about soft tissue injury to the supporting structures of a joint. Stressed views can also help evaluate the degree of instability in the joint, which assists in determining a treatment plan.
Placing the limb in a stance position while applying pressure to mimic body weight can simulate weight bearing. Evaluation of carpal hyperextension includes stressed views to determine the level of the injury and assist with treatment options (Piermattei et al., 2006).
Computed Tomography (CT)
Computed tomography (CT) is a complex X-ray modality that creates multiplanar two-dimensional cross-sectional images (Figure 20.1). This allows for evaluation of the area in slices and eliminates superimposition of structures that complicate diagnosis in standard radiography. The CT scanner has an X-ray generator and an X-ray detector. The X-ray generator moves around the patient generating X-rays at multiple projection angles that are picked up by the X-ray detector. In a stop-step CT unit, after projections are acquired, the patient is advanced to obtain the next slice along the longitudinal (z-) axis. Most modern units are helical or spiral scanners that allow for continuous movement of the patient through the scanner. This creates a more contiguous scan image and volumetric information for three-dimensional reconstruction. Images are generated through processing of the attenuation information from the X-ray detectors that is filtered and back-projected to form the digital image. The digital image is then converted to a grayscale analog image.
Figure 20.1 Computed tomographic image of a cross-sectional slice through the elbow joint. Arrow indicates a fragmented coronoid process.
Image courtesy of Toby Gemmill, Willows Referral Service, UK.

Computed tomography images are not limited to two planes. This is a significant diagnostic advantage when evaluating for fracture configuration, osteochondral fragmentation, or joint incongruity. CT is commonly used to detect abnormalities associated with elbow dysplasia that can be difficult to assess with standard radiography (Reichle et al., 2000; Gemmill et al., 2006; Moores et al., 2008; Bottcher et al., 2009; Cook & Cook, 2009; House et al., 2009). In addition to viewing the individual slices for pathology, three-dimensional rendering can be performed (Figure 20.2) (House et al., 2009; Dennison et al., 2010).
Figure 20.2 Three-dimensional rendering of the radius and ulna including the humeroradial and radiocarpal joints.
Image courtesy of Dr. Sherman Canapp.

Although particularly valuable for osseous injury, CT can be used to evaluate soft tissue lesions. CT has a low-contrast resolution and through manipulation of the window and CT number (Houndsfield Units), visualization of soft tissue structures can be optimized. CT is commonly used for injuries to the spine (Rossmeisl et al., 2004; Newcomb et al., 2011). It has also been used to evaluate soft tissue pathology of the canine stifle, iliopsoas muscle, and upper airway (Gavin & Bagley, 2009; Samii et al., 2009; Stadler et al., 2011).
Magnetic Resonance Imaging (MRI)
Magnetic resonance imaging is superior for providing exquisite three-dimensional anatomic detail, especially of soft tissue structures (Figure 20.3). MRI relies on physical and chemical interactions between atomic nuclei, in particular the hydrogen atom (Berry, 2002; Cerezal et al., 2008). The magnetic resonance unit produces a signal secondary to changes in hydrogen atoms induced by the application of the magnetic field and radiofrequency pulses. These signals, utilizing Fourier transformation, generate a three-dimensional image through computer processing. The images are produced in high-resolution gray scale with increased signal appearing white and decreased signal black. Water and fat contain a higher proportion of hydrogen ions, so most of the signal acquired is from these components.
Figure 20.3 Sagittal T1-weighted image of the shoulder joint. T1-weighted images provide excellent anatomic detail.
Image courtesy of Dr. Pat Gavin.

Multiple image sequences are obtained. T1-weighted, T2-weighted, and Short T1 Inversion Recovery (STIR) are the foundation magnetic resonance sequences (Berry, 2002). The sequences selected depend on the type of unit (high field vs. low field), area being studied, and suspected pathology. In T1-weighted images, fat has an increased or hyperintense signal and water a relatively decreased signal. Anatomic detail is superior in T1-weighted images because of reduced tissue contrast. In T2-weighted images, water has an increased signal and fat a hypointense signal. T2-weighted images are best for visualizing pathological changes, but provide less anatomic definition. Increased fluid signal on MRI, a result of inflammation, edema, or hemorrhage, is a hallmark of tissue damage. STIR suppresses the fat signal within tissues and is often used to confirm or identify pathological changes in bone where there can be increased signal due to the fat in bone marrow. Sclerosis within bone is seen as decreased signal in non-fat-suppressed sequence images.
Gadolinium-based contrast agents can be used to enhance magnetic resonance sequences. They are commonly used to improve visualization in angiography and in studies of neoplasia and cerebrospinal disease. Indirect (performed by intravenous injection of the contrast agent prior to obtaining the desired MRI sequence) and direct (injection of the agent into the joint) arthrography are considered the gold standard for magnetic resonance evaluation of human joints (Applegate et al., 1993; Vahlensieck et al., 1996; Waldt et al., 2007; Jung et al., 2009; Pozzi et al., 2009; Van Dyck et al., 2009; Rakhra, 2011). Differences in specificity and sensitivity between these two techniques appear minimal, with the biggest disadvantage being lack of joint distention using indirect arthrography (Pozzi et al., 2009; Schaefer et al., 2010; Rakhra, 2011). Direct magnetic resonance arthrography has been used to enhance evaluation of canine stifle and shoulder joints (Banfield & Morrison, 2000). In the stifle, soft tissue structures were more readily identifiable, and in a group of military working dogs, some pathological changes were only visible with direct contrast arthrography (Fitch et al., 1997; Pujol et al., 2011).
While the focus of most canine magnetic resonance studies has been joints, there is increasing interest in imaging soft tissues, and reports include examination of the gastrocnemius, supraspinatus, and iliopsoas muscles, and the long digital extensor tendon (Berry, 2002; Drost, 2002; Murphy et al., 2008; Lafuente et al., 2009; Stahl et al., 2010).
Ultrasonography
Diagnostic ultrasonography generates an image through use of high-frequency sound waves that are pulsed through tissue from an ultrasound transducer. Images depend upon the wavelength, frequency, and velocity of the sound waves through the tissue (Drost, 2002; Nyland et al., 2002). These sound waves are reflected back to the transducer as echoes that are converted to an electrical signal to produce a grayscale image. The image produced is a real-time, anatomical picture of the area to which the ultrasonography transducer is being applied (Figure 20.4).
Figure 20.4 Cross-sectional images of the right and left triceps tendon. In the right limb, there is significant disruption in the fiber pattern of the triceps tendon when compared to the normal left tendon.

Acoustic impedance is the reflection characteristic of the tissues through which the sound waves travel. The acoustic impedance of soft tissue falls between that of air and bone, making ultrasonography good for the evaluation of soft tissues, but poor for air-filled structures and pathology beneath the surface of or behind bone.
Ultrasonography is not yet commonly used in diagnostic imaging of canine musculoskeletal injuries. In equine practice, diagnostic ultrasonography plays a principal role in the diagnosis of musculoskeletal damage, particularly of tendons and ligaments. The reluctance to use ultrasonography in canine practice might be due to the need to acquire and evaluate images in real time, and the lack of education on its use. Increasing recognition of soft tissue injuries in the canine athlete is making ultrasonography increasingly popular.
Ultrasonographic appearance of normal canine anatomy and normal and pathological canine conditions have been documented (Kramer et al., 1997, 1999, 2001; Long & Nyland, 1999; Gnudi & Bertoni, 2001; Knox et al., 2003; Lamb & Wong, 2005; Nielsen & Pluhar, 2005; Vandevelde et al., 2006; Liuti et al., 2007; Cannon & Puchalski, 2008; Cogar et al., 2008; Arnault et al., 2009; Caine et al., 2009; Cook & Cook, 2009; Seyrek-Intas et al., 2009; Turan et al., 2009; Marino & Loughin, 2010; Piorek & Adamiak, 2010; Hittmair et al., 2012). Ultrasonography is most useful for evaluating pathological changes in extra-articular soft tissue structures including tendons, muscles, and ligaments (Breur & Blevins, 1997; Gnudi & Bertoni, 2001; Kramer et al., 2001; Nielsen & Pluhar, 2005; Vandevelde et al., 2006; Cogar et al., 2008; Arnault et al., 2009; Cook & Cook, 2009; Seyrek-Intas et al., 2009; Hittmair et al., 2012). It is less useful when evaluating bone, and intra-articular structures such as cruciate ligaments and menisci. In two studies assessing the value of ultrasonography to diagnose cranial cruciate ligament rupture (CCL), only 19.6% and 15.4% of dogs with CCL rupture were identified (Gnudi & Bertoni, 2001; Arnault et al., 2009). This low sensitivity is not surprising considering the location of the cruciate ligament and the physics of ultrasonography. Bone fragments, osteochondral lesions, and fragmented medial coronoid process are difficult to evaluate for similar reasons (Cook & Cook, 2009; Seyrek-Intas et al., 2009).
Diagnostic ultrasonography can be performed in the awake or lightly sedated patient, and the cost of equipment is significantly less than magnetic resonance, making it more widely accessible to the sports medicine specialist. Though it is used primarily to assess and identify musculoskeletal injuries, it can be used to monitor healing. Whereas multiple MRI exams are impractical, ultrasonography can be offered quite reasonably for recheck examinations.
Figure B20.2 T2-weighted sagittal image of the shoulder. The yellow arrow indicates the tear in the supraspinatus tendon at the insertion. The red arrow indicates fatty replacement of normal tendon at the myotendinous junction.
(Image courtesy of Dr. Pat Gavin.)

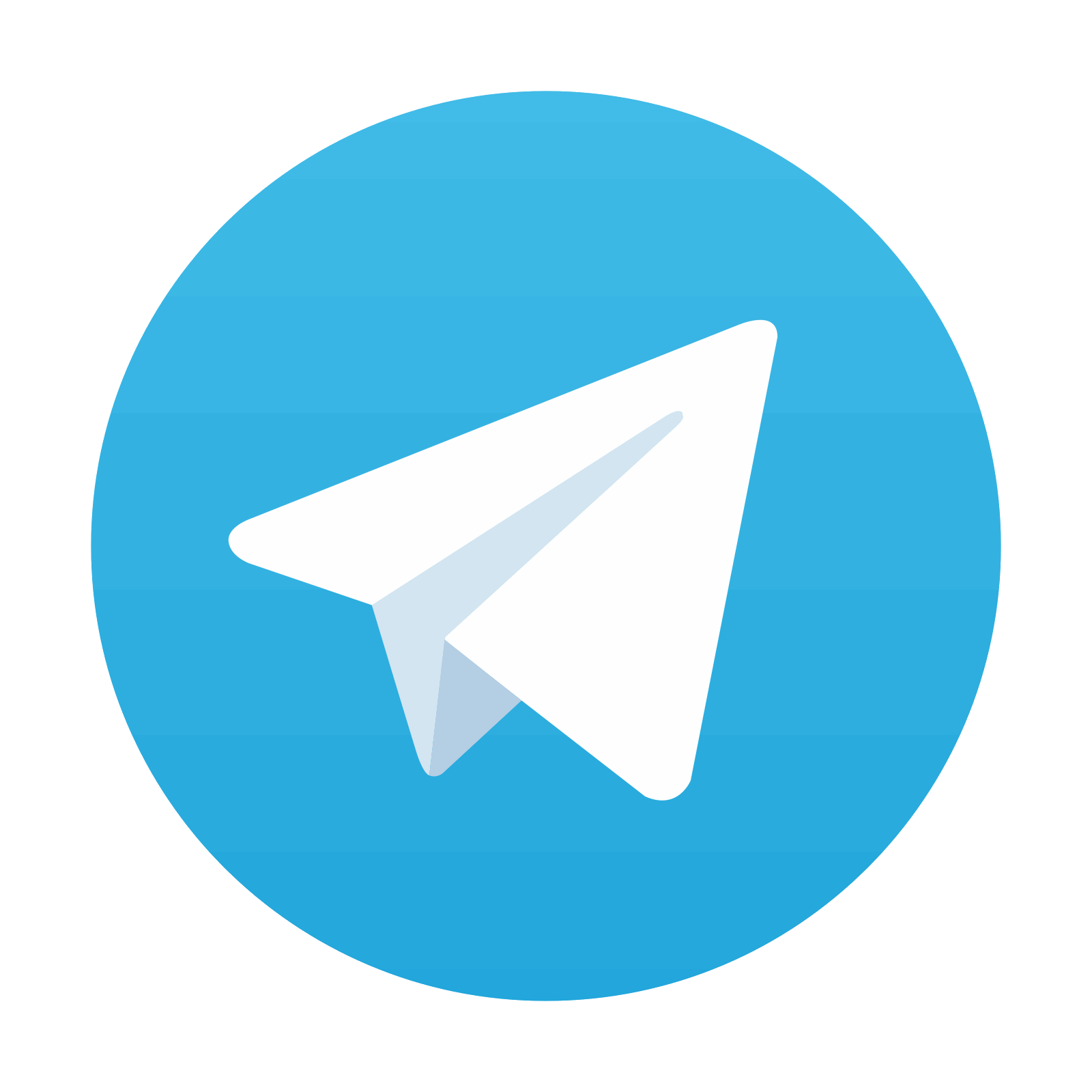
Stay updated, free articles. Join our Telegram channel

Full access? Get Clinical Tree
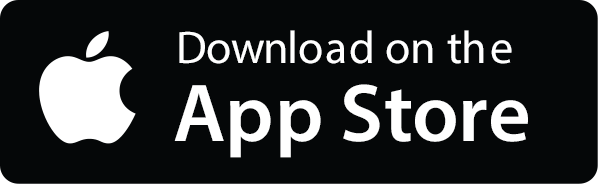
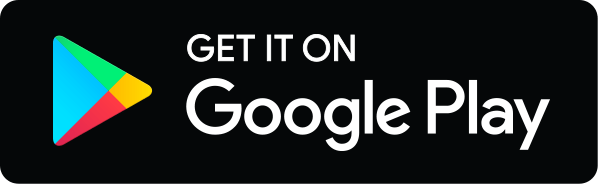