3 Hendra and Nipah Viruses International Center for Infectiology Research (CIRI), Hendra (HeV) and Nipah (NiV) viruses are two of the most dangerous viruses in the world. Their emergence from a zoonotic reservoir in South-east Asia and Oceania caused human outbreaks with fatality rates rising up to 100% among infected people. Due to their high pathogenicity and the lack of appropriate prophylaxis or treatment, HeV and NiV are classified as biosafety level 4 (BSL-4) pathogens. They are also classified as category C potential agents of bioterrorism. The study of these intriguing viruses is therefore particularly restricted and hampered for security reasons. In 1994, a new virus causing severe respiratory disease in horses was identified in the Brisbane suburb of Hendra, Australia. The Hendra virus was rapidly proved to cause severe illness in humans who had been in close contact with infected horses (Murray et al., 1995). In 1998, in Malaysia, a new disease emerged in pigs, causing large epizootics that were initially thought to be due to Japanese encephalitis virus (JeV). Because many of the people exposed to the porcine epizootic tested negative for JeV, the emergence of a new virus was suggested. In 1999, the Nipah virus was first isolated from a human fatality from the Sungai Nipah village (Mohd Nor et al., 2000). These viruses show features of the Paramyxoviridae family but possess particularities that led to the creation of the Henipavirus genus, named after the contraction of the two names. Henipaviruses are large-enveloped single-stranded RNA viruses of negative polarity (Fig. 3.1). Their genome is the largest among the Paramyxoviridae viruses (≈18.2 kb) and contains six transcriptional units coding for six structural proteins (N, P, M, F, G and F). Within the phosphoprotein (P) gene, the presence of a single editing site allows for the addition of one or two Guanine (G) residues during transcription by the viral polymerase. Translation of edited transcripts results in proteins that share the same amino-terminus as that of P protein but each carries a unique carboxyl-terminus. The protein with the +1 frameshift corresponds to the V protein and the protein with the +2 frameshift is known as the W protein. Another open reading frame within the P gene allows the production of a small protein known as C protein (Eaton et al., 2006). The P, V, W and C proteins were proved to be involved in the inhibition of the immune host response and act as virulence factors (Basler, 2012). HeV and NiV are both harboured by several species of fruit bats from the Pteropodidae family, which range from South-east Asia and Australia to Africa, and better known as flying foxes (Fig. 3.2). These giant bats, displaying a wingspan of up to 1.7 m, live in colonies of thousands of individuals and feed mainly on fruits and flowers, which they locate by smell. Once fruit bats were identified as the main reservoir for Henipaviruses, serological studies and immunosurveillance showed the circulation of Henipaviruses among the fruit bat population of the whole South-east Asia and Oceania. Recent studies revealed the circulation of Henipavirus-like viruses in Africa, where clinical cases have not yet being registered. Deforestation and agriculture intensification led to a decrease of bats’ natural habitat and represent critical factors responsible for the crossing of the species barrier (Pulliam et al., 2012). In this context, farm and domestic animals such as pigs or horses were more easily exposed to bat droppings, which are the primary source of contamination. Evidence of NiV infection was also found in cats, dogs, horses, cows and goats, but was mainly restricted to dogs for HeV (Geisbert et al., 2012). The respiratory form of the disease in domestic animals, especially in horses and pigs, induces high production of contaminating aerosol particles and allows transmission to humans. Concerning NiV, two other ways of human contamination were discovered in Bangladesh. The first suggests the drinking of raw palm sap contaminated with bat saliva and/or droppings (Salah Uddin Khan et al., 2011) and the second concerns human-to-human transmission through aerosol in the case of close contact with infected patients (Homaira et al., 2010). Fig. 3.1. Structure of Henipaviruses and their genome organization. (A, B) Schematic structure of Henipaviruses and arrangement of the viral proteins (C) The negative-sense genomic RNA of Henipaviruses is presented here in the 3′ to 5′ orientation. All genes, except the P gene, are monocistronic. The P gene encodes for three non-structural proteins: V, W and C, which are 454 aa, 449 and 166 aa long, respectively. In humans, NiV and HeV show similar clinical symptoms, although much more is known about the pathogenesis of NiV, given the larger number of human cases with this virus. After an incubation period of up to 2 weeks, patients develop flu-like symptoms, frequently followed by fatal encephalitis with multiple organ failure. The neurological symptoms may be associated with a pulmonary syndrome. NiV lethality ranges from 40 to 100% and HeV lethality varies with a similar range. Relapse and late-onset disease, even years after first exposure, was documented for both viruses (Wong and Tan, 2012). Fig. 3.2. Flying foxes, their distribution and the locations of disease outbreaks caused by Hendra virus and Nipah virus. The geographic distribution of the viruses strongly correlates with the animals’ home range. In naturally infected animals, and especially in pigs and horses, pulmonary symptoms are more prominent. In pigs infected by NiV, the morbidity rate rises to 100% and the mortality rate varies from 1 to 40%, depending on the age. In many cases, the symptoms appear to be extremely subtle, but in animals that clearly develop clinical signs, a combination of neurological and respiratory syndrome was observed. In porkers, the respiratory syndrome leads to a harsh non-productive cough, a condition commonly termed ‘barking pig syndrome’ and is considered to be the first characteristic of the disease. In sows, infection is usually related to sudden death or febrile syndrome (>39.9°C), followed by agitation and head pressing, champing of the mouth, drooling, nystagmus, myoclonus, tetanus-like spasm and seizures, varying degrees of rear leg paresis and pharyngeal muscle paralysis. In piglets, it remains unclear whether the high mortality rate is due to the viral infection itself or the inability of sows to nurse their litter (Mohd Nor et al., 2000). In HeV infected horses, the clinical course of the disease usually lasts less than 36 hours after an incubation period up to 12 days. The disease starts with an acute febrile syndrome (>40°C) followed by an increase in the respiratory and heart rates. The disease progresses to neurological (head pressing, head tilt, ataxia and circling) and respiratory signs (laboured breathing and frothy nasal discharge) (Field et al., 2010). In other domestic species such as dogs and cats, HeV infections are usually asymptomatic, but some infected animals may present similar respiratory and neurological clinical signs as in equines (Mills et al., 2009). The life cycle of Henipaviruses is quite similar to other viruses of the Paramyxoviridae family. However, they possess specific traits responsible for their high pathogenicity and their potent zoonotic properties. The broad tropism among mammalian species is related to their highly conserved cellular surface receptors, Ephrin B2 and B3, which are broadly distributed in many cell types including neurons and endothelial cells (Maisner et al., 2009). In natural cases, the infection happens mainly via the oronasal route. In this case, the first replication site is located in the epithelium of the oronasal and upper respiratory tract. Then, the virus uses leukocytes as conveyors to reach the second replication site, endothelial cells of the blood vessels, which strengthens the systemic dissemination (Mathieu et al., 2011). Thus, vasculitis is one of the major features observed in tissues as well as thrombosis and parenchymal necrosis. Studies in pigs suggest that Henipaviruses could use two different routes to invade the central nervous system, involving either a breach of the blood–brain barrier or neuroinvasion via cranial nerves. Once it reaches the brain, the virus can spread from neuron to neuron and cause focal destruction of the parenchyma as observed in the MRIs of infected patients. Indeed, Henipaviruses are cytopathogenic viruses that cause cell-to-cell fusion leading to the formation of giant multinucleated cells called syncytia. These syncytia are responsible for the death of cells and the subsequent vascular breach, haemorrhages and necrosis observed in tissues. The inflammation that follows, induced by necrosis and thrombotic areas, leads to the recruitment of leukocytes in the brain that participate in the development of the meningo-encephalitis observed in histology (Wong and Tan, 2012). The first immune mechanism to be triggered after pathogen entry is the type-I interferon (IFN-I) pathway. After its entry into the host cell, the viral genome is sensed by cytoplasmic RIG-like-receptors (RLR) – such as RIG-I or MDA5 – or by membranous toll-like-receptors (TLR) such as TLR3. This recognition leads to the phosphorylation and activation of several transcription factors, such as interferon regulatory factor 3 (IRF3), that translocate into the nucleus to stimulate the transcription of IFN-I genes. IFN-I is synthesized and released in the extracellular compartment (Fig. 3.3A). The released IFN activates surrounding cells, either by autocrine or paracrine means, expressing the IFN-I receptor (IFNAR). On its activation, IFNAR will phosphorylate signaling molecules, STAT1 and STAT2, leading to their dimerization. The STAT1/STAT2 dimer is translocated to the nucleus, where it activates the transcription of more than 300 IFN-stimulated genes (ISG) responsible for the production of proteins with antiviral properties such as PKR, OAS or Mx (Fig. 3.3B). In addition to their severe cytopathic effects, Henipaviruses are able to counteract this fine-tuned mechanism and have evolved strategies to control the IFN pathway. Indeed, the four proteins encoded by the P gene (P, V, W and C) could inhibit IFN-I signaling by different strategies. The V and W proteins have the ability to block the signaling pathway that leads to the activation of IFN-I production in the cytoplasm and in the nucleus, respectively. It seems that, regarding its nuclear localization, the W protein is much more efficient at blocking (Shaw et al., 2005) than the V protein. The V protein was also demonstrated to inhibit the activity of melanoma differentiation-associated protein 5 (Mda5) and laboratory of genetics and physiology 2 (LGP2) helicases, two sensors of dsRNA involved in triggering IFN-I production (Parisien et al., 2009). The precise action of the C protein still remains unknown, although it has been recently shown that it could regulate the production of proinflammatory cytokines in an infected host (Mathieu et al., 2012). When overexpressed in cells, these NiV proteins (P, V and W proteins) are also able to block IFN-I signaling by disturbing the formation and trafficking of STAT1/STAT2 dimers (Basler, 2012). Because STAT plays an important role in the activation of ISG, responsible for the production of antiviral proteins, inactivation of STATs has important consequences on the immune response. However, when observed in infected cells, the inhibition of IFN-I signaling pathways is not as strong as in overexpression experiments (Virtue et al., 2011). This suggests that in natural conditions the levels of P, V, W and C proteins are not sufficient enough to block IFN-I signaling. Therefore, the precise mechanism used by Henipavirus proteins to counteract the innate immune system remains to be understood. Fig. 3.3. IFN induction pathway and its inhibition by Henipaviruses. (A) Non-structural proteins V and W were shown to interfere with IFN induction at different levels. When overexpressed V, W and P protein could also inhibit IFN signaling (B), although this issue is still not completely resolved and the exact mechanism of complex interactions between Henipavirus and innate immune system remains to be understood. *Effects of viral proteins when overexpressed in cells remains to be confirmed during natural infection. Early diagnosis is a key issue to control disease expansion. Besides the clinical diagnosis, laboratory confirmation is essential, considering the huge health and economic implications that a confirmed case could represent. In 1999, in Malaysia, 1.1 million pigs were slaughtered and 956 farms were destroyed, representing a loss of 126.8 million euros for the Malaysian economy (Hosono et al., 2006) and causing the unemployment of 31,800 people (Lam, 2003). Laboratory diagnoses are ruled by regularly updated guidelines, edited by the World Organization for Animal Health (OIE), in the Manual of Diagnostic Tests and Vaccines for Terrestrial Animals (Anonymous, 2008). The accurate detection of NiV and HeV must be supported by an appropriate sampling procedure, which requires a comprehensive understanding of viral pathobiology. Indeed, the collection site will depend on the time after exposure: sample collection from excretion or tissues for agent identification may be possible during clinical exam or necropsy, whereas blood collection for serological analysis will be relevant 10–14 days after infection. Authorities recommend systematic samples from brain, lungs, kidney and spleen. Samples from mediastinal lymph nodes and, in pregnant animals, uterus, placenta and foetal tissues may also be of great diagnosis value. Samples should be transported at 4°C if they can arrive at the reference laboratory within 48 hours or be frozen using dry ice or liquid nitrogen if the shipping period is longer than 48 hours. However, samples should not be kept at –20°C for a long period. Because Henipaviruses are classified as BSL-4 microorganisms, their transportation must comply with the International Air Transport Association Dangerous Goods Regulations and samples must be submitted to reference laboratories in specially designed containers. Molecular tests give rapid results with very high sensitivity. They can be used for early detection in suspected animal outbreaks as well as for confirmation of human cases. Conventional polymerase chain reaction (PCR) was used with success for many years but was recently superseded by quantitative real-time PCR (qPCR), which is much more sensitive and specific. Several tests and primers have been developed, depending on the technology platform available, and the possibility of discriminating NiV from HeV. These primers are mostly based on the N gene, but also M, P and L genes (Feldman et al., 2009). Progress in next-generation sequencing (NGS) technologies allows massive sequencing of samples to detect emergence of new strains and control the genetic diversity among host isolates. Such analysis demonstrated that HeV outbreaks do not correlate with a particular isolate but seem to mainly depend on host and environmental factors (Smith et al., 2011). Virus isolation remains highly advisable to confirm a new outbreak or to identify a new host. However, given the high pathogenicity of both viruses, these experiments have to be carried out in BSL-4 laboratories. To obtain rapid results when such facilities are not available, experiments to confirm suspicion may be conducted in a BSL-3 laboratory, but highly stringent local guidelines must be applied to protect operators and the population. If cytopathic effects (CPE) compatible with paramyxovirus-like infection are observed, all samples, cultured cells and supernatant have to be transferred to a BSL-4 laboratory. Henipaviruses grow very well to high titre on Vero cells. CPE usually develop after 3 days of culture, even if authorities recommend two passages, 5-days each, to declare the sample negative (Anonymous, 2008). At low multiplicity of infection, CPE are characterized by formation of giant multinucleated cells called syncytia that may contain over 60 nuclei aggregated in the middle or distributed in a ring along the membrane, depending on its maturity (Hyatt et al
INSERM: U1111, Lyon, France
3.1 Introduction
3.2 Pathogenesis
3.2.1 Clinical signs
3.2.2 Pathobiology
3.3 Diagnosis
3.3.1 Sampling and submission of samples
3.3.2 Molecular diagnosis
3.3.3 Virus identification
Stay updated, free articles. Join our Telegram channel

Full access? Get Clinical Tree
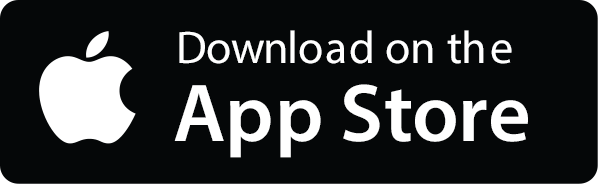
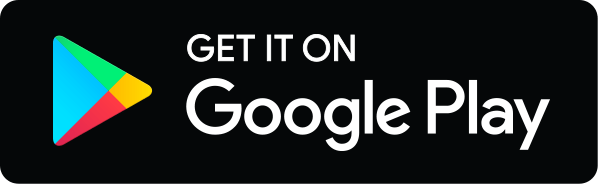