Chapter 6
Hemoglobin-Based Oxygen Carrier Solutions*
Marie K. Holowaychuk1 and Thomas K. Day2
1Critical Care Vet Consulting, Calgary, Alberta, Canada
2Veterinary Emergency Service/Veterinary Specialty Center, Middleton, Wisconsin, USA
*Reproduced and modified with permission from Wiley: Day, T.K. (2003) Current development and use of hemoglobin-based oxygen-carrying (HBOC) solutions. Journal of Veterinary Emergency and Critical Care 13, 77–93.
Introduction
The shortage of blood products in both human and veterinary medicine, the transmission of bloodborne diseases, and the growing concern regarding storage lesions have resulted in the continued attempt to develop solutions that are able to carry oxygen, similar to blood products that contain red blood cells (RBCs). The goal of hemoglobin-based oxygen carrier (HBOC) solutions is to provide a product that can carry oxygen to tissues at least as effectively as whole blood or packed RBCs, but without the concern of disease transmission, transfusion reactions, or other transfusion-related complications. Although HBOC solutions can deliver oxygen, the solutions are not meant to substitute all of the properties of blood (e.g., platelet and clotting factor function), nor is the goal to replace entire the entire blood volume. The term “blood substitute” is therefore a misnomer, although its use is widespread in the literature. A more appropriate term would be “red cell substitute” or “artificial oxygen carrier”.
HBOC solutions have been developed for two major purposes: (1) as a “bridge oxygenator” that functions as an alternative to blood transfusions in an attempt to avoid, reduce, or delay administration of allogenic blood and (2) to improve tissue oxygenation of organs with poor blood supply. HBOC solutions are especially ideal for pre-hospital resuscitation or for use in the battlefield when allogenic blood products are not readily available. In addition, HBOC solutions can be used for human patients with rare blood types when compatible allogenic blood products are not obtainable or for patients who refuse allogenic transfusion for religious purposes.
Because HBOC solutions are considered drugs, governing bodies such as the United States Food and Drug Administration (USFDA) must approve them before clinical use. Science and technology focused on creating HBOC solutions has resulted in the development of many products that have progressed through various stages of approval from the USFDA. However, only one solution has gained full approval from the USFDA, the veterinary product known as Oxyglobin® (Hemoglobin Oxygen Therapeutics LLC, Souderton, PA; previously Biopure, Cambridge, MA and OPK Biotech, Cambridge, MA). At the time of writing, Oxyglobin® was unavailable from the manufacturer, which stated it would resume distribution to the United States and European Union in the future.
Oxygen delivery and content
Blood is essential for the body to deliver oxygen to tissues. Blood accomplishes this by exposing RBCs to ventilated areas of the lung and then distributing these RBCs to systemic tissues. Oxygen delivery is expressed as the milliliters of oxygen delivered to the peripheral tissues each minute and is a product of cardiac output and arterial oxygen content. On average, tissues extract about 25% of delivered oxygen (oxygen extraction ratio), leaving 75% of oxygen in the venous blood as oxygen reserve. To a certain extent, if oxygen delivery decreases, the oxygen extraction ratio increases, to ensure that oxygen consumption remains relatively constant. This relationship varies from tissue to tissue depending on the normal oxygen extraction ratio of the organ. Once oxygen delivery decreases below the critical threshold, oxygen consumption becomes dependent on oxygen supply and anaerobic metabolism can occur. Therapeutic interventions are thus aimed at preventing the breach in the threshold of oxygen delivery.
Oxygen is poorly soluble in plasma and is only present at concentrations of 0.003 mL per 100 mL of plasma per 1 mmHg or approximately 0.3 mL per 100 mL of plasma at a partial pressure of oxygen (PO2) of 100 mmHg (Muir and Wellman 2003). The capacity of blood to transport much larger amounts of oxygen than can be carried in the plasma is therefore dependent on the concentration of hemoglobin (HGB) molecules in RBCs. Without HGB, cardiac output would have to increase more than 40-fold to maintain oxygen delivery above the critical value of 3.0–5.0 mL/kg/min that is required in most mammals (Lieberman et al. 2000).
Hemoglobin and oxygen affinity
The affinity of HGB for oxygen is determined by the PO2, partial pressure of carbon dioxide (PCO2), pH, body temperature, RBC concentration of 2,3-diphosphoglyceride (DPG), and the chemical structure of HGB. The oxygen-hemoglobin dissociation curve illustrates the relationship between the percentage saturation of HGB with oxygen (SO2) and the PO2 (Figure 6.1). The heme portion of the HGB molecule is responsible for oxygen binding and has a strong, but finite, cooperative effect with regards to its oxygen binding, as reflected by the sigmoid shape of the oxygen–hemoglobin dissociation curve. In other words, if one oxygen molecule binds to a heme, HGB changes its conformation in such a way that binding of more oxygen molecules to the remaining heme molecules is facilitated.

Figure 6.1 Oxygen hemoglobin dissociation curve. (From Aldridge, P. and O’Dwyer, L. (eds) Practical Emergency and Critical Care Veterinary Nursing, 1st edn. John Wiley & Sons.)
The PO2 at which the HGB is 50% saturated is termed the P50 and is used as a measure of HGB affinity for oxygen. Increases in the PCO2, body temperature, and RBC 2,3-DPG or adenosine triphosphate (ATP), or decreases in pH (acidosis), shift the oxygen-hemoglobin dissociation curve to the right, thereby increasing the P50 and thus decreasing the affinity of HGB for oxygen (Herrmann and Haskins 2005). This enables more oxygen to be off-loaded to tissues, such as during exercise when there is usually an elevated body temperature, CO2 production, and lactic acidosis; this ensures enhanced delivery of oxygen to the muscle.
The P50 of human HGB is 26–29 mmHg. This is a result of the presence of 2,3-DPG, which binds to the N-terminal histidine residue of the beta-chain of HGB and has a stronger binding with deoxyhemoglobin than with oxyhemoglobin. The P50 of canine HGB is approximately 29–31 mmHg, which is close to that of people. However, feline HGB has a higher P50 of 36 mmHg, meaning that the oxyhemoglobin dissociation curve is right-shifted and that feline HGB has a lower affinity for oxygen compared to dogs or people (Herrmann and Haskins 2005). Feline RBCs are unique in that they contain only small amounts of 2,3-DPG and thus have a considerably lower oxygen affinity compared to mammals with higher concentrations of 2,3-DPG. Instead, cats, as well as cows and other ruminants, rely on chloride as the major effector molecule for oxygen affinity (Ingerman 1997).
Bohr and Haldane effects
The Bohr and Haldane effects are used to describe changes in the affinity of oxygen for HGB. The Bohr effect states that the oxygen affinity for HGB decreases in the presence of CO2. Thus, with an increase in PCO2, HGB will bind oxygen with less affinity and facilitate its release into tissues. The Haldane effect refers to deoxygenation of blood increasing its ability to carry CO2. This is because reduced or deoxygenated HGB is a better proton acceptor than the oxygenated form. Thus, the enhanced affinity of deoxyhemoglobin for protons enhances synthesis of bicarbonate and subsequently increases the capacity of deoxygenated blood for CO2. In addition to enhancing removal of CO2 from oxygen-consuming tissues, the Haldane effect promotes dissociation of CO2 from HGB in the presence of oxygen, thereby off-loading CO2 in the lung.
Hemoglobin encapsulation
The encapsulation of HGB within RBCs has several important biological benefits, including the determination of oxygen saturation, prevention of nephrotoxicity, and maintenance of colloid osmotic pressure (COP). Free HGB has a lower intravascular half-life and P50 than blood and significantly increases the COP of whole blood. The high intracellular concentration of 2,3-DPG compared to plasma facilitates the release of oxygen from HGB at the level of the tissues (Muir and Wellman 2003). Once free in the plasma (such as with intravascular hemolysis), HGB dissociates into alpha and beta dimers, which initially bind to haptoglobin and are removed by macrophages in the liver. When haptoglobin stores become depleted, free HGB dimers are then cleared by the kidneys. Renal clearance of large quantities of HGB dimers can result in their precipitation in the proximal tubules, resulting in severe tubular injury in people (Tracz et al. 2007).
Early HBOC solutions
The search for a blood substitute dates back to 1656, when Christopher Wren attempted to replace the blood of animals with wine (Creteur et al. 2000). The theory was that the alcoholic spirit of wine could replace the “vital” spirit of blood and that “bad” blood caused disease. Interest in finding a true “blood substitute” for human patients arose in the 1930s when a bovine hemolysate solution was administered to different species of animals, including cats (Amberson et al. 1934). The first published report of using this solution in humans appeared in 1949, which described a successful “resuscitation” with small volumes of a HGB solution in a young woman with postpartum hemorrhagic shock (Amberson et al. 1949).
Thereafter, partially purified, unmodified stroma-free HGB solutions were used to treat hemorrhagic shock, resulting in marked clinical improvement of cardiovascular parameters (Rabiner et al. 1970). However, toxicity with products not completely free of stroma resulted in life-threatening anaphylactic shock. Subsequent to that, an HGB solution containing 1.2% residual lipid stroma was infused in healthy volunteers, resulting in side effects including abdominal pain, malaise, decreased heart rate, increased blood pressure, and altered kidney function (Savitzky et al. 1970).
The severe side effects of these and other solutions that were developed during this time were attributed to small amounts of erythrocyte cytoskeleton remnants and membranes in the products (Haney et al. 2000). Anaphylactic reactions were attributed to the lipopolysaccharide bound to HGB and intravascular coagulation was most likely the result of residual stroma in the HBOC solutions. Likewise, nephrotoxicity was a direct effect of HGB dimers derived from the degradation of HGB tetramers. Consequently, dozens of HBOC solutions have been developed and studied during the past several decades in an effort to create a safe product with minimal untoward side effects.
Development of an ideal HBOC solution
An ideal HBOC solution should lack any substantial toxic or adverse physiologic effects. Free HGB quickly dissociates into alpha and beta dimers that are eliminated via the kidneys within 1–2 hours, which can theoretically lead to a short half-life and nephrotoxicity. HBOC solutions therefore must contain HGB that is modified such that it has a sufficient half-life and does not induce nephrotoxicity or stimulate immunologic reactions, the coagulation cascade, the complement system, or other defense mechanisms. HBOC solutions should also not increase the white blood cell count or react with plasma substitutes or platelets, and the production of methemoglobin from oxidative reactions with HGB should be minimized. Finally, because free HGB is a nitric oxide (NO, potent vasodilator) scavenger, HBOC solutions must be altered so that vasoconstriction is minimized.
HBOC solutions should be cost-effective and at least comparable to allogenic transfusions. Allogenic blood transfusion requires blood typing and crossmatching prior to administration, needs special storage conditions, and has a limited shelf-life. Universal compatibility, long shelf-life, ease of use and storage (including stability at room temperature), and immediate availability in large quantities are sought-after advantages of HBOC solutions.
Hemoglobin source
An effective HBOC solution should provide sufficient oxygen uptake at physiologic conditions and be similar to endogenous HGB in terms of O2 and CO2 transport and delivery. Given that HGB contained in canine (and human) RBCs has a P50 of approximately 30 mmHg, deriving an HBOC solution with a similar P50 is desired. Unfortunately, human-derived HBOC solutions produced by lysis of RBCs obtained from outdated banked blood have a reduced P50 of 10–16 mmHg (Palaparthy et al. 2000). The resultant increase in oxygen affinity must be overcome by modification of the HGB to effectively deliver oxygen. Given the lack of available expired human blood products due to the ongoing shortage of human blood donors and lack of expired human blood products, interest in the use of an alternative HGB source arose.
Due to the readily available supply of bovine RBCs (from the beef cattle industry), HBOC solutions containing bovine HGB were developed. Despite the low levels of 2,3-DPG bound to bovine HGB, it has an oxygen affinity similar to human and canine HGB (Winslow 2000). This is because chloride ions take the place of 2,3-DPG and provide the majority of the oxygen-carrying capacity, similar to cats. Bovine HGB also has more pronounced Bohr and Haldane effects compared to human HGB, thereby making its oxygen uptake, transport, and release to tissues more efficient (Muir and Wellman 2003). Unfortunately, the risk of transmission of transmissible spongiform encephalopathy (TSE), although remote to non-existent, is a concern when using bovine RBCs as a source of HGB in some countries. The regulatory process for approval of new HBOC solutions in the United States therefore mandates that TSE clearance validation studies, in addition to other pre-clinical pharmacologic, pharmacokinetic, toxicology, and efficacy tests, are performed (Simoni et al. 2012).
Efforts have also been made to engage in large-scale production of recombinant HGB in Escherichia coli and yeast, whereby alpha globin chains are fused to yield an undissociable tetramer. These HGB molecules were also engineered to reduce NO affinity (Roberts and Prowse 2013). Unfortunately, the process of HGB purification by this method has been limited by low yields, high costs, and side effects similar to other forms of HGB (Vernado et al. 2013).
Purification of hemoglobin
Once the HGB moiety is removed from the RBC membrane there are several steps in the purification process, including washing, lysing, ultrafiltration, and pasteurization. Crosslinking and/or polymerization of HGB tetramers serves to provide a larger molecule, thereby increasing the molecular weight of the HGB moiety. The larger HGB molecule results in less extravasation and a longer half-life, and generates the COP of the solution. The development of modified HBOC solutions that are crosslinked and/or polymerized has resulted in marked improvement in oxygen-carrying capacity, a longer half-life, and fewer side effects (Haney et al. 2000).
Vasoactive properties
One of the most controversial effects of HBOC solutions is the increase in systemic vascular resistance. The balance between vasodilation and vasoconstriction closely follows the activity of NO, a ubiquitous highly diffusible gas that acts as a signaling molecule. When NO is produced in the vascular endothelium, it interacts with the enzyme guanylate cyclase to produce vasodilation. NO that diffuses into blood reacts with oxyhemoglobin (its principal scavenger) and deoxyhemoglobin to form S-nitrosohemoglobin (Muir and Wellman 2003). The intravascular half-life of NO is approximately 2 ms in whole blood, but is more than 500 times shorter when exposed to HBOC solutions (Thomas et al. 2001). HBOC solutions can also generate the release of reactive oxygen species that can react with NO, inhibit NO synthase, and possibly increase the release of endothelin, another potent vasoconstrictor (Biro 2012). Ultimately, the administration of HBOC solutions can dramatically reduce NO concentrations, thereby reducing the vasodilation effects of NO and promoting vasoconstriction.
Differences exist between HBOC solutions and their vasoactive properties (Winslow 2000). Diaspirin crosslinked solutions have marked vasoactivity, whereas the polymerized formulations have mild to moderate vasoactivity. However, even among polymerized formulations, large differences in increases in arterial blood pressure exist, suggesting that other properties are contributing. Factors likely affecting the vasoconstriction that occurs with HBOC solution administration include the HBOC concentration and molecular dimension, rheologic properties (viscosity), COP, degree of penetration into the vessel wall or activation of HGB receptors in the vessel wall, amount of sensitization of adrenoreceptors, and extent of release of other vasoactive mediators such as thromboxane or endothelin (Muir and Wellman 2003).
Immunomodulation
Concerns exist regarding the potential for increased susceptibility to infection after administration of HBOC solutions due to free HGB causing impairment of neutrophil function. HGB also provides a readily available source of iron, which can theoretically sustain growth and multiplication of bacteria. There are two conflicting reports in the literature, including one report of increased survival of Escherichia coli after a sublethal challenge and administration of an HBOC solution in a mice model of peritonitis (Griffiths et al. 1995), as well as a second similar study that investigated a different HBOC solution, but found no difference in bacterial growth between HBOC-treated and human albumin-treated groups of mice (Langermans and Bleeker 1995). The difference in these findings is not easily explained, but clinical use of HBOC solutions in other species has not demonstrated concerning findings with regards to incidence of sepsis or infection.
The risk of immunosuppression with HBOC solutions might not be more significant than that with blood transfusions, given the recent literature supporting the occurrence of transfusion-related immunomodulation (see Chapter 11). HGB breakdown results in the liberation of free iron, which could theoretically catalyze the production of oxygen free radicals and increase cytokine release, including tumor necrosis factor (TNF) (McFaul et al. 1994). However, administration of a lyophilized liposome encapsulated HBOC solution prevented release of TNF in rats (Rabinovici et al. 1994). A more recent study investigating use of an HBOC solution for resuscitation in an uncontrolled hemorrhagic shock model in swine found an increase in TNF after HBOC solution administration (Malkevich et al. 2008). Evidently, the risk of immunomodulation with HBOC solution administration has not been extensively investigated and remains unclear.
Oxidation
In order for HGB molecules in HBOC solutions to bind and release oxygen and minimize methemoglobin production, the heme molecule must remain in the reduced state. Free HGB is susceptible to oxidation, which forms methemoglobin, thereby reducing oxygen to the superoxide anion. Superoxide is the precursor to hydrogen peroxide, a free radical that can lead to oxidative injury. Normally, several enzymes in the RBC and plasma such as ascorbic acid and glutathione inhibit this reaction. Because HBOC solutions contain free HGB, there is a theoretical concern that the risk of oxidative injury will be higher with administration of these solutions (Dorman et al. 2002). HBOC solutions have not been studied extensively to determine the production of oxidative damage. However, an experimental model demonstrated that HBOC solution perfusion of rat hearts was protective against ischemia-reperfusion injury, as demonstrated by improved cardiac function and decreased myocardial necrosis, apoptosis, and infarction (Li et al. 2010).
Development of HBOC solutions for human use
Dozens of HBOC solutions have been developed worldwide, but the only countries to approve the use of HBOC solutions in people are Russia and South Africa. Many companies that were investigating the use of HBOC solutions in the 1990s have since abandoned any research or product development in the area and some have even filed for bankruptcy. The physical and chemical properties of previously investigated HBOC solutions are summarized in Table 6.1.
Table 6.1 Characteristics of hemoglobin-based oxygen carrier solutions investigated for use in people and animals (Spahn and Kocian 2005; Day 2003)
Product | Type | HGB source | HGB concentration | P50 (mmHg) | COP (mmHg) | Current status of development |
Hemassist® (Baxter Corporation, Deerfield, IL) | Diaspirin crosslinked hemoglobin | Human | 10 g/dL (100 g/L) | 32 | 42 | Stopped |
PolyHeme® (Northfield Labs, Evanston, IL) | Polymerized pyridoxylated hemoglobin | Human | 10 g/dL (100 g/L) | 28–30 | 20–25 | Stopped |
Hemopure® (HGB O2 Therapeutics, Souderton, PA) | Gluteraldehyde polymerized hemoglobin | Bovine | 13 g/dL (130 g/L) | 36–68 | 25 | Approved in South Africa and Russia |
Hemolink® (Hemosol, Mississauga, ON) | O-raffinose polymerized hemoglobin | Human | 10 g/dL (100 g/L) | 39 | 26 | Stopped |
Hemospan® (Sangart, San Diego, CA) | Polyethylene glycol conjugation | Human | 4 g/dL (40 g/L) | 6 | 55 | Phase II outside of United States |
Oxyglobin® (HGB O2 Therapeutics, Souderton, PA) | Gluteraldehyde polymerized hemoglobin | Bovine | 13 g/dL (130 g/L) | 34 | 42 | Approved in the United States and European Union for use in dogs |
COP, colloid osmotic pressure; HGB, hemoglobin; P50, the PO2 at which the HGB is 50% saturated.
Diaspirin crosslinked hemoglobin
The crosslinking of HGB tetramers with bis-(3,5-dibromaslicyl) fumarate produced the first-generation HBOC solutions including Hemassist® (Baxter Corporation, Deerfield, IL), which was composed of diaspirin crosslinked human HGB from outdated human blood products. It underwent extensive pre-clinical testing in animals before a multicenter randomized trial in the United States that enrolled 112 patients with traumatic hemorrhagic shock. The control group received 0.9% NaCl as their treatment; unfortunately, the Hemassist® group had a 72% higher mortality rate, which resulted in premature termination of the study (Sloan et al. 1999). Another multicenter trial was conducted in Europe and enrolled 121 trauma patients with severe hemorrhagic shock, but was also terminated prematurely when Hemassist® did not improve morbidity or mortality despite reducing the use of allogenic blood transfusions compared to standard fluid resuscitation (Kerner et al
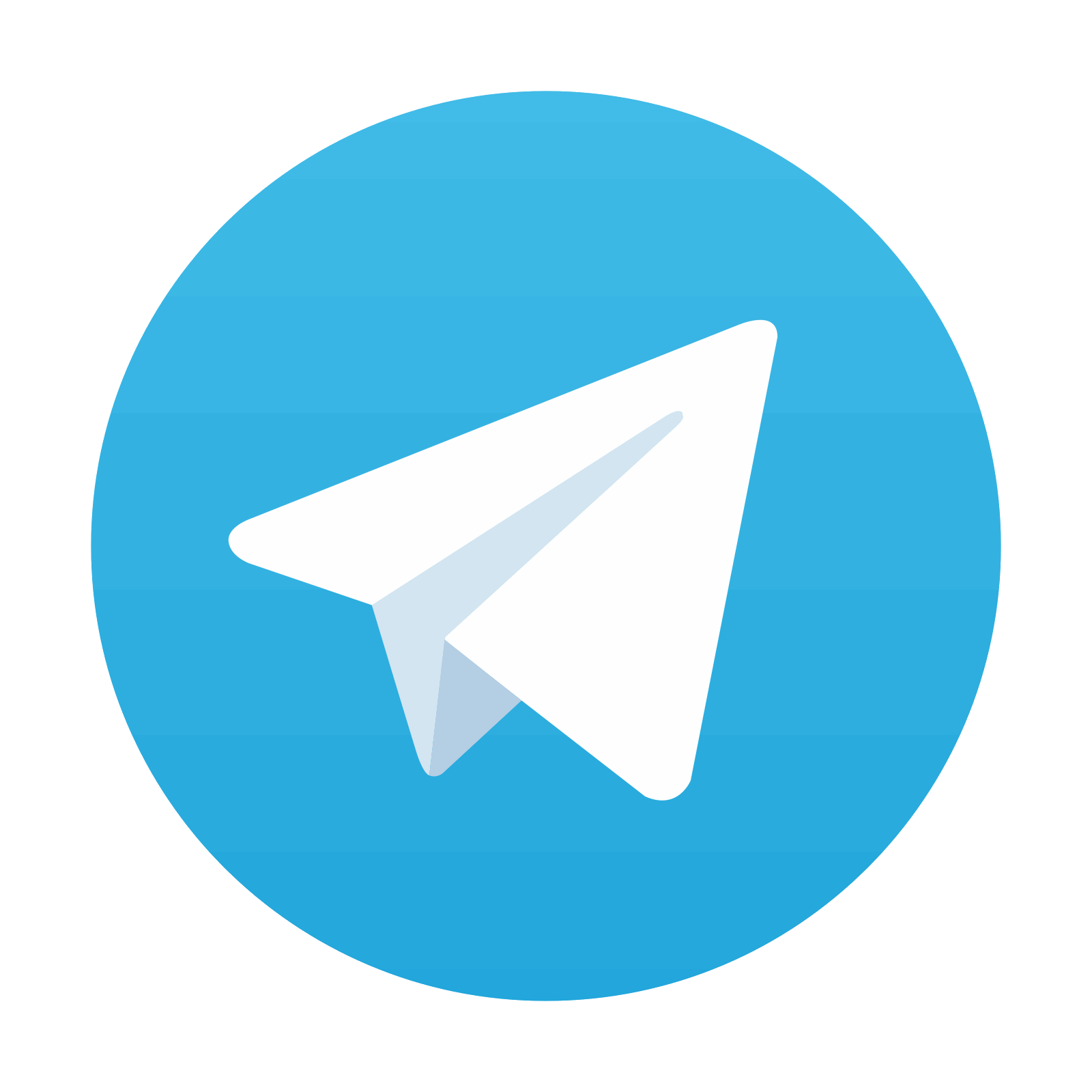
Stay updated, free articles. Join our Telegram channel

Full access? Get Clinical Tree
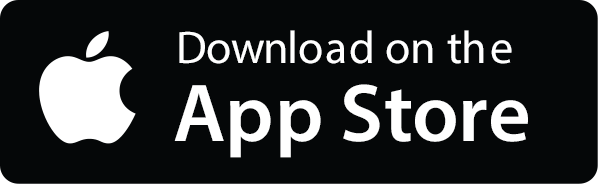
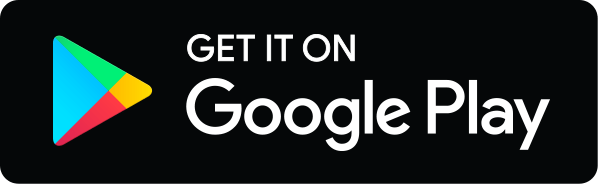