There is an association with a vitamin E deficiency in cases of NAD/EDM, although low vitamin E levels are not present consistently in all cases. It appears that vitamin E is a factor in the development of NAD/EDM in the first year of life in genetically predisposed foals (Blythe et al., 1991b). A study of risk factors associated with the development of EDM found that foals from dams that had previously produced an EDM-affected foal were at a significantly higher risk (25 times more likely) of developing EDM than were foals from other dams, whereas low serum vitamin E concentrations were not found to be a significant risk factor in disease development (Dill et al., 1990). In the family of Quarter Horses with NAD/EDM, we performed quantitative Real-Time PCR for alpha-tocopherol transfer protein (TTPA) mRNA to determine if the correlation between low vitamin E and NAD could be due to decreased or absent absorption of vitamin E, and there was no significant difference in expression between NAD-affected and unaffected horses (Aleman et al., 2011). In addition, we have performed serial measurements of serum, CSF and muscle vitamin E concentrations in affected horses before and after supplementation and found that vitamin E concentrations could be increased in serum, CSF, and muscle post-supplementation (unpublished results). Overall, there is very strong evidence that NAD/EDM is an inherited disorder, and it may be that vitamin E concentrations act as environmental modifiers to determine the overall severity of the phenotype of horses affected with NAD/EDM – a theory that has been supported by the research to date (Aleman et al., 2011; Blythe et al., 1991a).
An ante-mortem diagnosis of NAD/EDM is based solely on clinical signs, the elimination of other causes of neurologic disease, and possible association with a low serum vitamin E concentration. Definitive diagnosis requires histopathological evaluation of brainstem and spinal cord tissue at post-mortem. Histological lesions in NAD/EDM consist of dystrophic neurons and axons, vacuolization and spheroid formation (Beech, 1984). The distinction between NAD and EDM is made based on location of the lesions. Lesions in NAD are confined strictly and bilaterally to the lateral (accessory) cuneate, medial cuneate, and gracilis nuclei of the caudal myeloencephalon and the nucleus thoracicus (Clarke’s nucleus) of the spinal cord from the first thoracic vertebrae (T1) to the third lumbar vertebrae (L3) (Beech, 1984; Miller & Collatos, 1997). With EDM, there is additional bilateral axonal degeneration of the dorsal spinocerebellar and ventromedial tracts. Transport disruption of axonal proteins has been recognized in affected nuclear regions (Siso et al., 2003). Currently, there is no treatment for NAD/EDM, although vitamin E supplementation may stabilize clinical cases in addition to preventing severe signs in predisposed individuals (Mayhew et al., 1987; Dill et al., 1990; Aleman et al., 2011).
NAD has been associated with neurologic disease in humans, sheep, cats, and dogs; however, the clinical and specific histopathological findings vary. In the majority of NAD cases in various species, dystrophic neurons and axons are most commonly found in specific nuclear areas of the gray matter in brain and spinal cord (Siso et al., 2006). In canine and feline NAD, there is involvement of the cerebellum, which is not observed in horses (Chrisman, Cork, & Gamble, 1984; Carmichael et al., 1983; Cork et al., 1983). In humans, an underlying genetic basis for the disease has been identified in two of the five NAD disorders: infantile NAD (mutations in PLA2G6) and Juvenile/Adult Hallervorden-Spatz disease (mutations in PANK2 gene) (Zhou et al., 2001; Khateeb et al., 2006; Morgan et al., 2006). Equine NAD/EDM varies considerably from human NAD in both clinical signs and affected neuroanatomical pathways, and therefore a candidate gene approach is unlikely to identify a putative mutation for the disease in horses.
The prospective breeding trial in Morgan horses with NAD performed by Beech and Haskins (1987) demonstrated either an autosomal dominant mode of inheritance with variable expression or a polygenic mode of inheritance. Pedigree analysis performed on a group of Quarter Horses affected with NAD support an incompletely penetrant autosomal dominant mode of inheritance. Alpha-tocopherol transfer protein (TTPA) has been definitively excluded as a candidate gene through sequencing and expression studies (Finno, et al. 2012). At this time, genotyping of 133 horses has been performed using the EquineSNP50 Beadchip (Illumina, San Diego, CA); a genome-wide association study with family data (GWAF) is in progress.
Shivers
Shivers (shivering) is a chronic nervous or neuromuscular syndrome in horses that has been recognized since 1886 (Williams, 1886) and affects primarily draft-horse breeds, but has also been reported in Warmbloods, Warmblood crosses, and, less commonly, Quarter Horses and Thoroughbreds (Deen, 1984; Valentine et al., 1999; Davies, 2000; Firshman, Baird, & Valberg, 2005; Hunt et al., 2005). Although it has been reported that there is no sex predilection (Firshman et al., 2005), evidence from the University of Minnesota (UMN) Neuromuscular Laboratory reveals that shivers is predominantly diagnosed in male horses (80%) as compared to females (20%) (Valberg, 2009). There is a variable age of onset, with the mean age reported from UMN Neuromuscular laboratory of 6.4 years and a range from 1 to 18 years of age (Valberg, 2009). The disease is characterized by “shivering” and hyperflexion of the pelvic limbs and an elevated tail head caused by involuntary spasms of the muscles in the pelvic region and tail. The muscles of the thigh and quarters appear to quiver when the leg is hyperflexed. These signs are often most evident when the horse is moving backward (Baird, Firshman, & Valberg, 2006). Clinical signs are often exacerbated by excitement. Less commonly, there may be involvement of the muscles of the thoracic limbs, neck, or face. Pelvic limb weakness was evident in 58% (11/19) of Belgian horses with shivers (Firshman et al., 2005).
Diagnosis is primarily made based on clinical observation. There is no significant difference in muscle enzyme concentrations (creatine kinase and aspartate aminotransferase) or vitamin E concentrations in horses with shivers compared to control horses (Firshman et al., 2005). Although hypothesized for some time that shivers was a manifestation of polysaccharide storage myopathy (PSSM), no significant association was found between a diagnosis of PSSM and a diagnosis of shivers (Firshman et al., 2005). In most cases, shivers is progressive and gradually debilitating. Various treatments have been attempted, with no success, including acupuncture, chiropractic adjustment, and supplementation with magnesium or vitamin E. Recommendations include maintaining the horse in fit condition and providing frequent turnout in an effort to stabilize clinical signs (Valberg, 2009). Post-mortem examination is unrewarding in these cases, with no documented histopathological lesions observed despite extensive examination of the central and peripheral nervous systems (Mayhew, 2005; Valentine et al., 1999).
An underlying familial tendency to shivers is suspected, and it has been suggested that shivers is inherited or has a hereditary predisposition (Green, Davies, & Doucet, 1995). Shivering has been classified as a hereditary disease in the United Kingdom (Tutt, 1964). Currently, a genome-wide association study is being performed utilizing the EquineSNP50 (Illumina, San Diego, CA) (Valberg, personal communication).
Cervical vertebral malformation and malarticulation
Cervical vertebral compressive myelopathy (CVCM) – also known as cervical vertebral stenotic myelopathy, cervical vertebral malformation and malarticulation (CVM), spinal ataxia, cervical stenotic myelopathy, and “wobbler’s” syndrome – is one of the most common causes of neurologic disease in horses worldwide. CVCM can be divided into two subsets of disease manifestation. The first (type I) is a developmental disease, caused by vertebral malformation or malarticulation that leads to a dynamic instability of the vertebral canal and is most common in young horses. There is a higher incidence of type I CVCM in Thoroughbred horses, and an inherited basis has been suggested. It has been demonstrated that young male horses are at risk and dietary factors, such as a high carbohydrate ration, low dietary copper and excess dietary zinc may predispose individuals to type I CVCM (Kronfeld DS & Donoghue, 1990). Type II CVCM occurs more commonly in older horses and results from osteoarthritic enlargement of the articular processes, typically within the caudal cervical vertebrae (C5, C6, C7). It does not appear to be a breed predisposition for type II CVCM, but it has been suggested that individuals are predisposed to develop type II CVCM due to a relatively narrow vertebral canal (Van Biervliet, 2007).
Clinical signs of CVCM include usually symmetric upper motor neuron dysfunction, leading to spastic paresis; and sensory dysfunction resulting in ataxia and proprioceptive deficits of all limbs. All limbs could be equally affected or more typically the pelvic limbs more affected than the thoracic limbs. Ante-mortem diagnosis has been supported by plain cervical radiography, myelography, computed tomography, and exclusion of other causes. Pathologic changes, including mild subluxation of the vertebrae, physeal enlargement and dorsal projection of the caudal physis of the vertebral body, osteoarthritis of the articular processes, osteochondrotic changes at the articular processes, and caudal extension of the dorsal vertebral arch over the cranial physis of the next caudal body may be evident (Mayhew et al., 1993). A definitive ante-mortem diagnosis of CVCM is challenging and therefore accurate phenotyping of affected cases can be difficult. In an attempt to determine whether cervical vertebral canal stenosis is present, radiographic measurements have been used as common denominators for correcting the absolute mean sagittal diameter measurements into correct value. Both intravertebral and intervertebral ratios have been established (Moore et al., 1994; Hahn et al., 2008a). Cervical myelography may be useful in confirming a diagnosis of CVCM and is required to define the site of spinal cord compression if surgical intervention is to be performed. There are no established diagnostic criteria for compression based on myelographic observations, and false-positive diagnoses may occur (van Biervliet et al., 2004). If available, contrast-enhanced computed tomography (CECT) can accurately diagnose the location and severity of compressive lesions in cases of CVCM (Reed, 2007). Although CECT is promising as a diagnostic option, most CT units at veterinary practices and universities are unable to image adult equine cervical vertebrae; therefore, in most cases, post-mortem examination remains the only way to achieve a definitive diagnosis of CVCM.
Medical therapy in horses with CVCM involves reducing inflammation and edema in an attempt to reduce the compression on the cervical spinal cord. Use of nonsteroidal anti-inflammatory agents and dimethyl sulfoxide are common. In young horses (less than 1 year of age), restricted exercise and diet are recommended, with particular attention to meeting the minimum requirements of essential nutrients. In adult horses with compressive lesions that are graded as 1 to 3 ataxic based on the scale proposed by Lunn and Mayhew (1989) and are compressed at a maximum of two sites, ventral interbody fusion can be used to stabilize the affected joint(s) (Reed, 2007).
It has been suggested that inheritance plays a role in the etiology of CVCM. In 1950, Dimock proposed, based on post-mortem findings and neurological examinations in 121 Thoroughbreds with CVCM and known pedigree information, that the disease was hereditary (Dimock, 1950). Dimock observed that foals that developed CVCM were frequently by the same or closely related sires, with 43% of the affected cases appearing in one line and some mares producing two or three cases. Conversely, in 1976, an investigation into the genetics of CVCM in Thoroughbreds was performed in Great Britain, but no conclusive evidence was found to support a direct role of inheritance in cases of CVCM (Falco, Whitwell, & Palmer, 1976). Supporting this finding, no evidence was found to suggest that CVCM is directly heritable by simple Mendelian dominant or recessive patterns (Wagner et al., 1985). Interestingly, in that same study, although the breeding of an affected CVCM stallion to an affected CVCM mare did not produce a foal with CVCM, there was a high incidence of osteochondrosis (OC) (10 of 22 foals) and physitis (9 of 22) found in the offspring of the crosses. Osteochondrosis has been postulated to have a substantial heritable component (Grondahl & Dolvik, 1993). Subsequent work supported an association between CVCM and osteochondrosis, with the frequency and severity of OC lesions in articular cartilage of the appendicular skeleton (patella, distal humerus, femur, third metacarpal/metatarsal bone) greater in CVCM horses as compared to normal controls (Stewart, Reed, & Weisbrode, 1991). As nutritional factors and rate of growth appear to be factors in both the development of CVCM and OC, it may be that there is a genetic predisposition to develop either or both conditions as underlying inabilities to develop normal mature bone and cartilage. Efforts to investigate the genetic contribution to CVCM are currently under way.
From a comparative perspective, cervical spinal cord compression has been described in Great Danes and Doberman pinchers, with males more frequently affected than females, in a similar ratio to that of horses (approximately 2:1) (Trotter, 1985). Clinical presentation is similar to CVCM in horses. Breeding affected dogs results in a higher incidence of the syndrome in the progeny (Selcer & Oliver, 1975); however, the determination of a genetic predisposition to cervical myelopathy due to an inherited spinal canal stenosis awaits confirmation.
Sensorineural deafness in American Paint horses
There have been isolated case reports of American Paint horses suffering from sensorineural deafness, which was confirmed by brainstem auditory-evoked responses (BAER) (Harland et al., 2006). There is a small population of melanocytes within the inner ear that is essential for the development of the stria vascularis, a blood-vessel-rich zone of the cochlea that plays a role in modulating the chemical composition of endolymph and the resultant production of an endocochlear potential. In mice that have been genetically engineered to have no melanocytes in the stria (viable dominant spotting mice), the endocochlear potential is close to zero (wild type +100 mV) at all stages of development and the mice are profoundly hearing impaired (Steel & Barkway, 1989). In many species, congenital deafness is associated with abnormal migration of these melanocytes from the neural crest and poor survival within the inner ears of some animals with coat and iris pigmentation alterations (Steel & Barkway, 1989; Price & Fisher, 2001).
Recently, a case-control series evaluating 14 confirmed deaf, 20 suspected deaf, and 13 non-deaf American Paint horses was performed to further evaluate deafness in the American Paint horse by phenotype, clinical findings, BAERs, and endothelin B receptor (EDNBR) genotype (Magdesian et al., 2009). Horses were confirmed to be bilaterally deaf through BAER testing. In both the confirmed and suspected deaf groups, the most common coat color patterns were splashed white overo and frame-splashed white overo blends (Figure 14.2). All confirmed deaf horses had extensive white facial markings, one or more blue irides, partially pigmented palpebral skin, and limbs with white markings. Otoscopic examination, routine clinicopathological analysis of blood and serum, skull radiographs, and neurologic examinations revealed no abnormalities in the confirmed deaf horses. There is currently no treatment available for equine deafness; however, it is important to recognize the condition in order to counsel owners about management and handling of deaf horses.
When evaluating the confirmed deaf horses for the EDNRB mutation that is causative for LWFS (I118K), 7/8 confirmed deaf horses were carriers for the mutation and 1/8 was not (Magdesian et al., 2009). Therefore, a relationship between the mutation and deafness was established; however, this could represent two independent mutations (i.e., EDNRB and the true causative mutation for deafness in the American Paint horse) that are prevalent in certain overo horses.
In humans, there are several conditions that cause hypopigmentation and deafness. Type II Waardenburg syndrome is limited to hypopigmentation and deafness without any craniofacial deficits and is caused by a mutation in the Mitf gene, which is a central mediator of melanocyte development (Price & Fisher, 2001). Type IV Waardenburg syndrome includes deafness and hypopigmentation in conjunction with Hirschsprung’s disease and is associated with a mutation of the SOX10, EDN3, or EDNRB genes (Price & Fisher, 2001). It appears that the other genes involved in Waardenburg syndrome, namely Pax3, Kit, Sox10, EDN3, and EDNRB, mechanistically converge on Mitf, suggesting that Mitf plays a pivotal role during melanocytes development (Price & Fisher, 2001). Based on likely candidate genes, additional investigation into the genetics of deafness in horses is currently being pursued.
Equine inherited myoclonus
Suspected inherited myoclonus has been documented in Peruvian Paso horses. Three foals (aged 12 hours, 9 days, and 18 days at presentation) were evaluated at the University of California at Davis between 1988 and 1991. In the two older foals, weakness and lethargy were noted at birth and, in all three cases, the foals were hyper-responsive to auditory and tactile stimuli, demonstrating myoclonic contractions upon stimulation. Gait abnormalities were demonstrated, including a “rabbit hopping” of the pelvic limbs in one foal and an inability to stand without assistance in the second foal.
Diagnostics included a complete blood count, serum biochemistry, cerebrospinal fluid (CSF) analysis, pelvic radiographs, muscle biopsies, electrophysiological testing, and complete post-mortem examinations. Clinicopathological values for blood and CSF were within normal ranges, other than mild elevations in creatine kinase and aspartate aminotransferase. Pelvic radiographs, muscle biopsies and electrodiagnostic testing were unremarkable. On post-mortem examination, there were no gross or histopathological alterations noted in the central nervous system
Spinal tissue from two of these three cases was further evaluated by Gundlach et al. (1993). In calves with inherited myoclonus, there is a marked loss of spinal strychnine-sensitive glycine receptors (Gundlach et al., 1988; Gundlach, 1990). By using a [3H] strychnine-binding assay, it was demonstrated that [3H] binding was significantly reduced (by 40%) in cervical and lumbar spinal regions in the myoclonic horses when compared to control levels (Gundlach et al., 1993).
Glycine is a major inhibitory transmitter in the spinal cord and brainstem and acts by binding its receptor, transiently forming an anion-selective chloride transmembrane channel that increases chloride conductance and results in hyperpolarization, thereby inhibiting neuronal firing. In calves with myotonia, it has been demonstrated that there is an 80–90% loss of glycine receptors and the resultant phenotype is more severe than that observed in these two reported horses (Gundlach et al., 1993). It was confirmed in the two equine cases that there was only the loss of the glycine receptor and, in all other binding sites evaluated (muscarinic, β-adrenergic, GABAA/benzodiazepine and peripheral benzodiazepine receptor binding sites, and cholecystokinin receptor binding site), densities were normal when compared to control levels.
Inherited startle syndromes are recognized in humans and mice and are caused by defects in inhibitory glycinergic pathways. In humans, missense mutations in the glycine receptor α-1 subunit gene have been identified as molecular causes of inherited startle syndromes (Shiang et al., 1993). In mice, two autosomal recessive mutations have been described that resemble the startle-disease phenotype in humans: spasmodic and spastic. The spasmodic mutation (missense mutation in the receptor α-1 subunit gene) is similar to the human mutation and impairs the function of the glycine receptors by reducing the receptor’s sensitivity to agonists. The spastic mutation (intronic insertion of a LINE-1 transposable element (Kingsmore et al., 1994) or aberrant splicing of β-subunit mRNA (Mulhardt et al., 1994) prevents or diminishes the expression of glycine receptors, similar to what is observed in bovine and equine myoclonus. Both mechanisms result in reduced glycinergic inhibition in the spinal cord, resulting in a startle syndrome or myoclonus. Currently, there is ongoing research to definitively define the underlying molecular mechanism of equine myoclonus.
Juvenile idiopathic epilepsy
Juvenile idiopathic Epilepsy is a syndrome in Arabian foals of Egyptian lineage that is characterized by recurrent generalized seizures that are manageable and appear to be self-limiting, disappearing by 1 to 2 years of age (Mittel, 1987; Aleman et al., 2006). This form of epilepsy appears to be inherited; however, a proposed mode of inheritance had not been established. There appears to be no sex predilection (Aleman et al., 2006).
Clinical manifestations in affected cases include a history of multiple generalized or partial seizures, a decreased to absent menace response, blindness, and abnormal mentation ranging from lethargy to obtundation. In a review of 22 cases (Aleman et al., 2006), the median age at onset was 2 months (range of 2 days to 6 months). Clinical signs of seizure disorders can be divided into pre-ictal, ictal, and post-ictal signs. Pre-ictally, most foals exhibit either no clinical signs or mild behavioral changes. During the ictal phase, tonic seizures are observed followed by clonic motor activity in severe cases or focal head twitches and nystagmus in milder cases. These seizure episodes usually last for less than 1 minute and are responsive to treatment with benzodiazepines (diazepam, midazolam) (Mittel, 1987; Aleman et al., 2006). Post-ictally, blindness, lasting from a few minutes up to three weeks, was noted in 100% of the 22 cases reported by Aleman and colleagues. Other post-ictal signs included lethargy, disorientation, and obtundation. Injuries are common following seizure episodes, with corneal abrasions frequently noted (Mittel, 1987).
Diagnostics in suspect cases include routine clinicopathological evaluation of blood, serum, and CSF. An increase in serum creatine kinase and glucose may be noted on the serum biochemistry. The remainder of the clinicopathological evaluation is within reference ranges. Imaging of the head with plain radiographs and computed tomography reveal no abnormalities. The diagnosis of epilepsy is made primarily on clinical grounds and exclusion of other causes of seizures. Electroencephalography has been useful to document and characterize the presence of abnormal brain electrical activity in affected foals. In 9/13 foals, electrophysiological abnormalities, including spikes, sharp waves, spike and wave discharges, or multiple spike complexes were observed (Aleman et al., 2006). The majority of these changes were noted in the central region and the voltage maximum was often on midline. There were, however, times where the voltage maximum was localized to the left or right sides, indicating multiform events in some foals. In human cases of idiopathic generalized epilepsies, EEG findings should be abnormal even during the inter-ictal phase in untreated patients (Panayiotopoulos, 2005). If inter-ictal awake EEG findings in an untreated suspect equine juvenile idiopathic epilepsy case are unremarkable, it may be advisable to repeat the EEG while the patient is sleeping and during awakening as this may reveal abnormal activity. Intermittent photic stimulation has been demonstrated to induce seizures in humans with idiopathic generalized epilepsies (Panayiotopoulos, 2005); however, in the two foals where photic stimulation was performed during EEG readings, no epileptiform activity was noted (Aleman et al., 2006).
Therapeutic management of juvenile idiopathic epilepsy is generally successful. Phenobarbital is the main antiepileptic medication that has been used for the long-term control of seizures. Dose regimens vary among individuals and adjustments may be necessary. Phenobarbital concentrations should be considered therapeutic on an individual basis when seizures are controlled without causing excessive sedation (Aleman et al., 2006). Potassium bromide (KBr) may be added in some cases. Generally, affected foals will require anywhere from 2 to 9 months of treatment with antiepileptic drugs. Additional therapies include vitamin E and selenium supplementation and supportive care while adjusting the antiepileptic drug dosage as seizure-induced trauma may lead to abrasions, contusions, or fractures. Pneumonia was present in 50% of the cases diagnosed with juvenile idiopathic epilepsy and should be managed with appropriate antimicrobial therapy (Aleman et al., 2006). The prognosis for juvenile idiopathic epilepsy is excellent when the seizures are appropriately managed. The disease appears to be self-limiting, disappearing by 1 to 2 years of age.
There appears to be a strong hereditary component to juvenile idiopathic epilepsy, as all reported cases are in Arabian foals from Egyptian lineage. Many of the cases reported in the case series by Aleman et al. (2006) had siblings or parents that appeared to be affected with the same condition. In humans, many of the rare Mendelian idiopathic generalized epilepsies are ion voltage- and receptor-mediated channelpathies (Panayiotopoulos, 2005). There are three types of neonatal and infancy epilepsy syndromes in humans that are characterized by afebrile focal motor seizures that remit after weeks or months with administration of standard anti-epileptic drugs. All three syndromes have an autosomal dominant mode of inheritance with high penetrance (Baulac & Baulac, 2009).
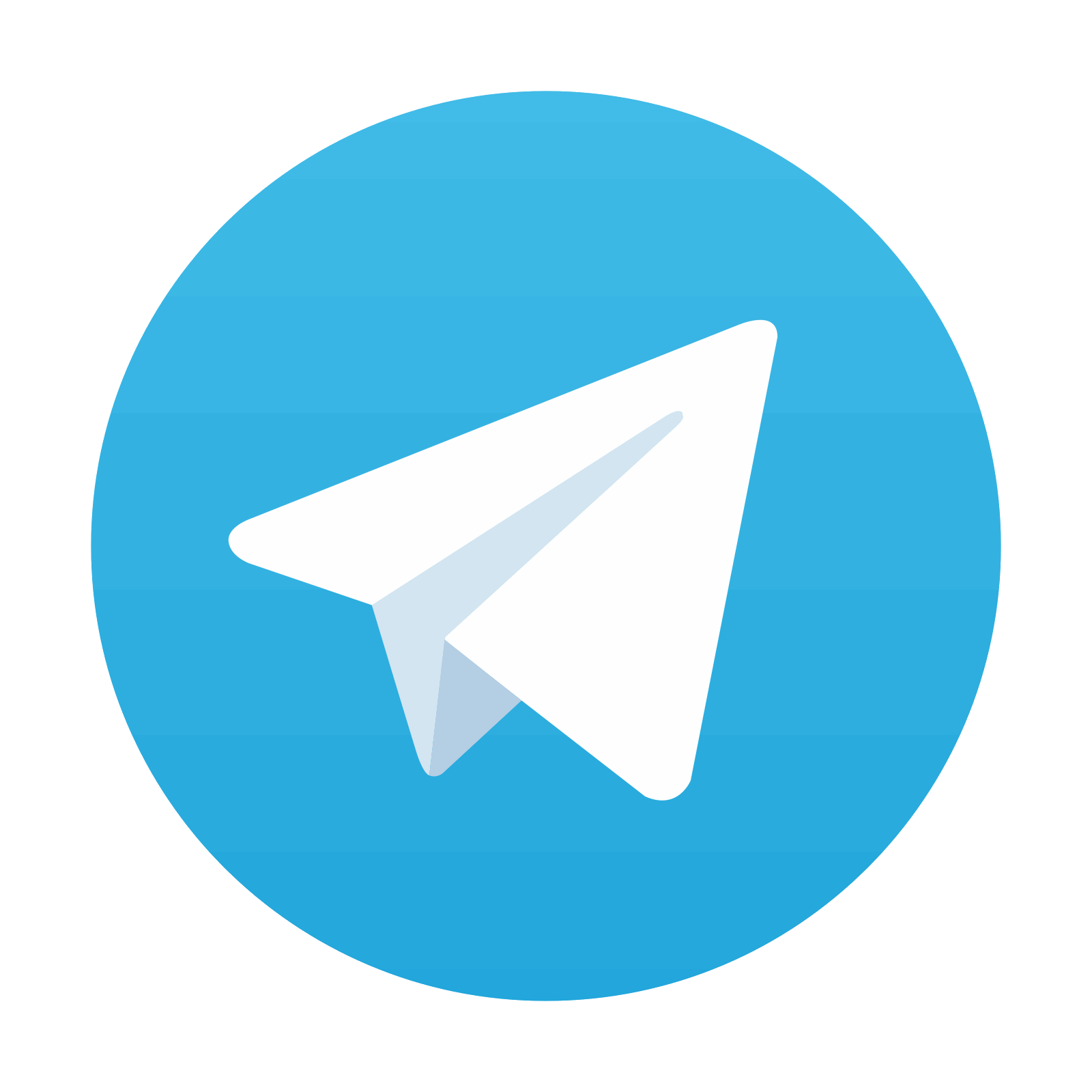
Stay updated, free articles. Join our Telegram channel

Full access? Get Clinical Tree
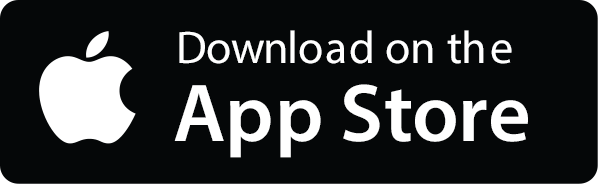
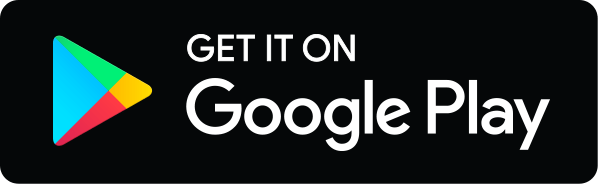