Systemic NMDAR antagonist administration
Global NMDAR genetic manipulation
Cell type-specific/brain region-restricted genetic manipulation of NMDAR
Molecular specificity
Variable (low PCP, high MK-801)
High
High
Cellular/regional specificity
No
No
Yes
Pros
Simple, fast, cheap, can be compared with equivalent studies in humans
Very reliable, gene specific
Very reliable, gene specific, area specific, cell type specific. Potentially inducible
Cons
Large methodological variations, multiple administration schemes. Potential off-target effects
Potential temporal and/or spatial compensatory mechanisms. Not inducible
Expensive and hard to develop. Time consuming for initial characterization.
Access
Widely available through commercial suppliers
Collaboration New initiative
Collaboration New initiative
Best use
Hypothesis-driven approach with a specific gene of interest
Hypothesis-driven approach with a specific gene and cell type/brain area
References
(45)
Among many brain areas/cell types that may potentially serve as a target of NMDAR hypofunction, we have focused on the cortical and hippocampal GABAergic interneurons. Basically, three lines of evidence suggest the idea that NMDAR hypofunction occurs preferentially in cortical GABAergic neurons in schizophrenia; first, acute systemic administration of NMDAR antagonists results in hyperactivity of cortical pyramidal neurons (30), and spillover of cortical acetylcholine (31) and glutamate (32). These paradoxical cellular changes concur with brain imaging data, showing net cortical excitation after NMDAR antagonist treatment in human subjects (33–35). Second, GABAergic interneurons are disproportionally more sensitive to NMDAR antagonists than are pyramidal neurons (36, 37), although the precise mechanism for this difference is unresolved. NMDAR antagonist-induced cortical excitation may be due to a preferential reduction in the firing of fast-spiking interneurons and resultant disinhibition of cortical excitatory neurons. Third, repeated administration of NMDAR antagonists reduces the levels of GAD67 and parvalbumin expression in cortical GABAergic neurons (38–42), directly linking NMDAR hypofunction to dysfunction of GABAergic neurons.
To directly test the role of NMDARs in GABAergic neurons in the development of schizophrenia-like phenotypes, we created a conditional NMDAR knockout mouse strain in which early postnatal ablation of NR1 was targeted to corticolimbic GABAergic neurons using the Cre/loxP system. At first glance, the approach may appear inappropriate because genetic predispositions of human schizophrenia are not manifested solely in corticolimbic GABAergic neurons. Nonetheless, we expected that by bypassing the temporal and spatial compensations inherent to the global knockout approach, the conditional transgenic approach may evoke the selective and characteristic impairments, thereby providing insights into the mechanisms of cellular and behavioral manifestation of schizophrenia-related phenotypes.
4 Methodological Challenge in Targeting Transgene Expression to Certain Cell Types of the Brains
The ability to target genetic manipulations to specific brain cell types is essential for analysis of genes, cells, and circuits that play key roles in behavior and disease. In particular, Cre recombinase driver transgenic mouse lines, which target the Cre expression to particular brain areas/cell types of interest, would be extremely useful by crossing them to a loxP-flanked transgenic line (“floxed”) of the targeted gene. Unfortunately, only a few Cre lines are available in which Cre expression is confined to particular cell types of brain areas and certain brain areas (e.g., 43, 44). As such, no Cre driver lines had been previously reported where the recombination is restricted to cortical and hippocampal GABAergic neurons. This is because no endogenous gene promoters have been discovered to drive the transgene expression to cortical and hippocampal GABAergic neurons throughout the development. Therefore, lack of appropriate promoter prevented our attempt from generating Cre lines using ES cell-derived homologous recombination approach (knock-in strategy). Alternatively, it is possible to use conventional transgenic approach of Cre recombinase over-expression by oocyte injection. This approach is to aim at screening out a particular line of desired Cre expression pattern, which may be determined by the combinatory effects of short transcriptional regulatory elements injected and positional effect at the transgene integration site in the genome. In particular, bacterial artificial chromosome (BAC) transgenic approach appears to be more feasible because the transcriptional enhancer/promoter elements contained in the BAC fragment would direct the transgene expression faithfully mimicking to the endogenous pattern. Note that this method would also allow us to pick the mouse line with slightly different expression pattern, due to the positional effect at the transgene integration site. Thus, it seems ideal to utilize this BAC transgenic approach if endogenous expression pattern is close to what you wish to obtain but not completely the same.
Here we adopted the BAC transgenic approach to target the Cre expression to corticolimbic interneurons. We utilized a BAC fragment carrying Ppp1r2 gene [protein phosphatase 1, regulatory (inhibitor) subunit 2] (45). Endogenous Ppp1r2 is expressed in GABAergic neurons in the striatum, the cortex, and the hippocampus and, to a lesser degree, pyramidal neurons in hippocampal CA1 throughout the development. Using this promoter for BAC-transgenic approach, we tried to obtain the Cre line, in which Cre is not expressed in the striatum. To the end, we inserted the Cre cDNA prior to the initial ATG of the promoter region of the gene by homologous recombination method in BAC (46) and injected the BAC fragment carrying Cre cDNA to mouse-fertilized oocytes to generate several transgenic lines. After screening by intercrossing with loxP-flanked Rosa26LacZ reporter line, we chose one line showing the desired pattern of Cre expression.
5 A Postnatal NMDA Receptor Ablation in Corticolimbic Interneurons Confers Schizophrenia-Like Phenotypes
Using the new generated Cre line, Ppp1r2-Cre, we successfully deleted NR1 subunit (GluN1) in 40–50% of cortical and hippocampal interneurons, predominantly parvalbumin-positive ones, in early postnatal development. This was confirmed by double in situ hybridization histochemistry and patch clamp recordings of NMDA-mediated currents. Consistent with the NMDAR hypofunction theory of schizophrenia, distinct schizophrenia-like symptoms emerged after adolescence in mutants. These included novelty-induced hyperlocomotion, which may reflect psychomotor agitation, a reduced preference for sweet solution (anhedonia), deficits in nesting and mating that suggest social withdrawal, and deficits in spatial working and short-term social memory that echo cognitive impairments; taken together, these deficits are reminiscent of the positive, negative, and cognitive symptoms of human schizophrenia. Impaired sensorimotor gating, as assessed by the PPI of the startle reflex, was also observed in these mutants. In addition, the mutants exhibited reduced GAD67 and parvalbumin levels in the NR1-deleted cortical GABAergic neurons, which concur with postmortem studies in the cortex of schizophrenia individuals (47, 48). Notably, deficits in social nest building, anxiety-like behaviors, and anhedonia-like behaviors were exacerbated by social isolation stress resembling stress precipitation of psychotic states in schizophrenia. Furthermore, the phenotypes of disrupted nest building and mating, as well as the anhedonic and anxiety-like behaviors, were most prominent after 12 weeks of age, suggesting the existence of a latency period before their emergence. This latency period is reminiscent of the premorbid stage before the emergence of symptoms characteristic of major psychiatric disorders (49). Spatial working memory and PPI deficits were ameliorated by the antipsychotic risperidone, conferring some degree of predictive validity to the model. Strikingly, post-adolescent deletion of NR1 subunit in the same interneuron population did not result in such abnormalities, demonstrating a fundamental role of NMDAR during early postnatal stages in the development of schizophrenia-like phenotypes later in adulthood.
In summary, our model not only reproduces positive, negative, and cognitive aspects of schizophrenia but also mirrors three additional features of human schizophrenia: stress-dependent precipitation of symptoms, a latency period before the development of symptoms, and a critical period for disease acquisition. It also exhibits non-behavioral features compatible with schizophrenia, like GAD67/parvalbumin decrease increasing the face validity of the model. All these features give our animal model unique properties, making it suitable for the study of schizophrenia pathophysiology and the testing of new approaches for treating human psychiatric illnesses and schizophrenia in particular.
6 Future Directions
While a number of schizophrenia susceptibility genes have been implicated in the modulation of NMDAR activity (50), genetic linkage studies have not directly associated polymorphisms in the NR1 subunit with schizophrenia (51). It is also unlikely that genetic predispositions of human schizophrenia are manifested solely in corticolimbic GABAergic neurons. Nevertheless, we expect that our strategy to spatially and temporally restrict NMDAR hypofunction will provide insights into the mechanisms of cellular and behavioral manifestation of schizophrenia-related phenotypes. Indeed, restricting the genetic deletion of NR1 to corticolimbic GABAergic neurons during early postnatal development was sufficient to trigger the development of schizophrenia-related phenotypes after adolescence in mice.
Future studies will address what could cause reduced NMDAR function in people with schizophrenia; how disrupted regulation of this receptor causes symptoms and how stress exacerbates them; and how currently used medications work to alleviate symptoms. Ultimately, understanding these processes will provide the basis for developing new targeted medications.
Acknowledgments
We would like to thank Veronika Zsiros, Elyse R. Sklar, Zhihong Jiang, Gu Yu, Yuqing Li, and Elizabeth M. Quinlan for their significant contributions as co-authors to our work cited in Ref. 45. We also acknowledge the colleagues for suggesting improvements to aspects of this review.
References
1.
Swartz, M. S., Perkins, D. O., Stroup, T. S., Davis, S. M., Capuano, G., Rosenheck, R. A., Reimherr, F., McGee, M. F., Keefe, R. S., McEvoy, J. P., Hsiao, J. K., and Lieberman, J. A. (2007) Effects of antipsychotic medications on psychosocial functioning in patients with chronic schizophrenia: findings from the NIMH CATIE study. Am J Psychiatry 164, 428–436.PubMedCrossRef
2.
Dossenbach, M., Erol, A., el Mahfoud, K. M., Shaheen, M. O., Sunbol, M. M., Boland, J., Hodge, A., O’Halloran, R. A., and Bitter, I. (2004) Effectiveness of antipsychotic treatments for schizophrenia: interim 6-month analysis from a prospective observational study (IC-SOHO) comparing olanzapine, quetiapine, risperidone, and haloperidol. J Clin Psychiatry 65, 312–321.PubMedCrossRef
3.
4.
5.
Javitt, D. C. and Zukin, S. R. (1991) Recent advances in the phencyclidine model of schizophrenia. Am J Psychiatry 148, 1301–1308.PubMed
< div class='tao-gold-member'>
Only gold members can continue reading. Log In or Register a > to continue
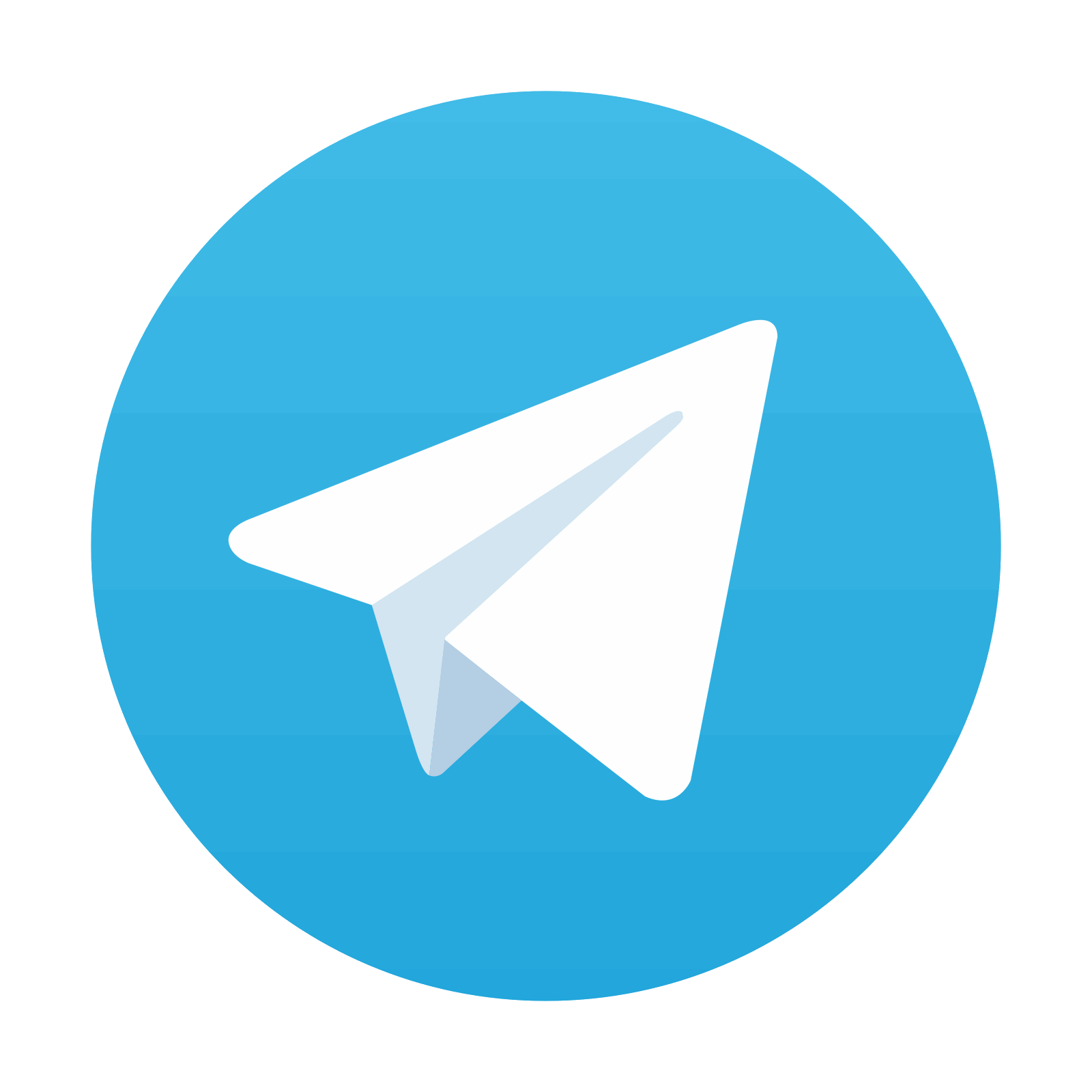
Stay updated, free articles. Join our Telegram channel

Full access? Get Clinical Tree
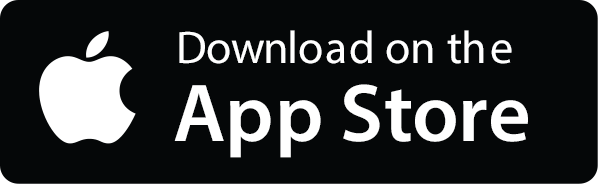
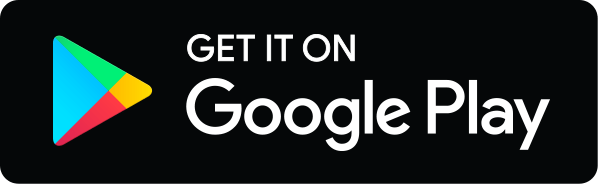