Raquel M. Walton IDEXX Laboratories, Inc., Langhorne, PA, USA Acronyms and abbreviations that appear in this chapter include: Hb, hemoglobin; MCH, mean cell Hb; MCHC, mean cell Hb concentration; MCV, mean cell volume; PCV, packed cell volume; POC, point of care; POCT, point‐of‐care testing; RBC, red blood cells; TP, total protein; TPRef, refractometer total protein; TS, total solids. Laboratory medicine, more commonly referred to as clinical pathology (or bioanalytical pathology), is a distinct specialty that overlaps other medicine specialties such as internal medicine and oncology in the area of diagnostics. In contrast to internists, clinical pathologists practice a systems‐based rather than problem‐based approach when interpreting hematological and biochemical results. However, in addition to recognizing disease‐associated changes, two other phenomena contribute to test interpretation: how test results are generated and how “normal” is defined. Artifacts due to sample preparation, sample condition or disease processes need to be identified and distinguished from true disease‐associated changes. Similarly, test interpretation is always performed in context – the context of health. The accuracy and sensitivity of tests and the use of appropriately established reference intervals are essential to the ability to diagnose disease. This chapter will provide selected information on hematological and biochemical test methodologies and validation, and will discuss the basic knowledge needed for generating and/or using reference intervals. The remainder of the book will address test interpretation using a systems‐based approach. Preanalytical factors that may affect test results should be minimized in order to ensure result accuracy [1]. Specimens should be collected according to standard practices and transported to the laboratory in a timely manner under conditions appropriate for the type of specimen and its stability. The minimum information on a specimen label for laboratory evaluation should include the full name of the patient (animal and owner), the patient signalment, and the specimen type (e.g., whole blood, serum or plasma). Especially for hematological evaluation, it is important that the patient’s signalment be correct as analyzer settings vary with respect to species. Anticoagulated specimens for hematology that have visible macroclots in the tube will produce variably erroneous results. Because the degree of inaccuracy cannot be predicted, clotted specimens are unsuitable for analyzis and these specimens should not be analyzed or submitted for analysis. Blood films and cytology smears should not be refrigerated and should be protected from condensation and freezing during transport to the laboratory to avoid condensation artifact (Figure 1.1). Failure to fully dry blood films or cytology preparations before placing them into slide holders can also result in moisture artefact. Figure 1.1 Condensation artifact caused by exposure of unfixed slides to moisture. The nucleated cells are lyzed and many hemoglobin crystals are present, formed from erythrocytes. Measurement of the percentage of red blood cells in whole blood can provide more information than simply the packed cell volume (PCV). In addition to the packed erythrocytes at the bottom of a microhematocrit tube, there is the white buffy coat layer and a plasma layer. The size of the buffy coat is related to the white blood cell (WBC) (and platelet) count; a thick buffy coat would indicate a high leukocyte (and/or platelet) count, whereas a scant buffy coat suggests leukopenia. The character of the plasma can also yield valuable information pertaining to a disease process, as well as contributing to spurious results. The plasma can appear hemolyzed, icteric or lipemic (Figure 1.2). Hemolysis in samples from horses usually indicates an in vivo phenomenon due to toxins or immune‐mediated disease (see Chapter 4). However, hemolysis can also occur during blood collection if excessive force or too small needle gauge is used in phlebotomy. Whether in vivo or in vitro, hemolysis produces a color change that can make refractometer readings difficult or interfere with spectrophotometric tests. Icterus indicates hyperbilirubinemia that usually exceeds 1.5 mg/dL (see Chapter 5). However, in herbivorous animals yellow‐colored plasma is not a reliable indicator of hyperbilirubinemia due to the presence of diet‐associated carotene pigments, which impart a yellow color to plasma. Icterus has not been demonstrated to interfere with refractometer readings [2]. Depending upon the chemistry analyzer, icterus can cause interference with some serum chemistry tests. Figure 1.2 Evaluation of plasma. From left to right: normal plasma color and consistency; lipemic and slightly hemolyzed plasma; hemolyzed plasma; icteric plasma. Lipemia is visible to the eye as increased turbidity in plasma or serum at triglyceride concentrations >300 mg/dL. Whether physiological (postprandial) or pathological (see Chapter 9), lipemia can cause spuriously high refractometer readings and will interfere with many chemistry tests. Protein can be rapidly and accurately measured by hand‐held refractometers. Because refractometers measure protein via a total solids‐based technique, the total dissolved solids in the sample affect light refraction. In addition to protein, total solids include electrolytes, glucose, urea, and lipids. The term “total solids” has caused much confusion in the reporting of refractometric protein results. Total protein (TP) and total solids (TS) are not synonymous. Currently, the vast majority of refractometers incorporate a conversion factor in their design so that the scales report TP and not TS. Contributing to the confusion is the fact that at least one refractometer is named the “TS meter” (AO Corporation) when it is in fact calibrated to report TP. While the altered refraction of plasma is mostly due to protein content, increases in lipid, glucose or urea content interfere with refractometric protein measurements. However, marked increases in urea or glucose (273 and 649 mg/dL, respectively) are needed to increase protein measurement by 0.4–0.5 g/dL. Increases in plasma cholesterol of 39 mg/dL are shown to increase the refractometer TP (TPRef) by 0.14 g/dL [2]. Another potential cause of erroneous refractometer readings is the addition of EDTA from K3EDTA anticoagulant tubes. At the standard concentration of EDTA (5 μmol/mL), K3EDTA by itself has minimal effect on the plasma’s refraction (≤0.1 g/dL increase). This is not true for peritoneal fluid, however, where overestimation of TPRef by 0.7 +/− 0.1 g/dL was reported in one study (see Chapter 18). At higher concentrations of EDTA (10 and 20 μmol/mL), EDTA can increase TPRef by 0.9–1.0 g/dL. Underfilling of EDTA tubes has the effect of increasing the EDTA concentration and will cause spurious increases in the TPRef [3]. Some commercial tubes with K3EDTA anticoagulant may also contain additives to prevent crystallization of the EDTA. Tubes that contain the additive may increase TPRef readings by up to 0.9 g/dL, even when properly filled. In general, polypropylene (plastic) tubes are more likely to include additives to prevent evaporation than glass tubes [4]. While sodium heparin anticoagulant has no effect on TPRef, heparin has deleterious effects on cellular morphology and is not recommended for samples that will be evaluated cytologically. Point‐of‐care testing (POCT) is defined as testing done at or near the patient with the expectation that results will be available quickly to facilitate immediate diagnosis and/or clinical intervention [5]. Whilst POCT provides quick, relatively inexpensive results with small volumes of blood, it also comes with its own set of risks. The major sources of error associated with POCT were categorized in one study as most often due to operator incompetence, nonadherence to test procedures, and the use of uncontrolled reagents and testing equipment [6]. Instrument calibrations and quality control measures may be omitted due to ignorance or the need for fast results. And, in veterinary medicine, analyzers may be used with species for which the instrument has not been validated. It should also be noted that diagnostic instruments for veterinary use are not subject to government regulations as they are for human use, which means that devices may not have been independently evaluated or tested [7]. Finally, poorly maintained instruments that are carried from one area to another may be a source of nosocomial infection or may transmit antibiotic‐resistant bacterial strains [5]. As part of the process of ensuring accuracy in an analytical method, calibrators and controls are used. A calibrator is a material of known or assigned characteristics that is used to correlate instrument readings with the expected results from the calibrator (or standard). A control is a preparation of human or animal origin intended for use in assuring the quality control of the measurement procedure, not for calibration. Controls usually represent abnormal and normal concentrations of the measured analyte. Currently, there are some POC analyzers marketed as “maintenance free” that do not come with controls and some that do not have calibrators. These instruments should be used with caution as there is no way to verify assay accuracy. Many POC hematology analyzers are based upon impedance methodology. Examples include the HM series (Abaxis, Union City, CA), the HemaVet 950 (Drew Scientific, Oxford, CT), the HemaTrue® (Heska, Loveland, CO), and the scil Vet abc™ (Scil, Gurnee, IL). Impedance technology employs an electric current that flows through a conductive liquid. When cells, which are nonconductive, pass through an aperture containing this fluid, there is an electrical impedance created for each cell that is proportional to the size of the cell. The impedance method facilitates measurement of the mean RBC and platelet volumes, as well as enumeration of WBCs, RBCs, and platelets. The WBCs (and any nucleated red blood cells) are counted separately from RBCs and platelets after cell lysis. Hemoglobin (Hb) concentration is also measured after RBC lysis. In the isotonic solution, nucleated cells are prevented from being counted along with RBCs and platelets because they are too big to pass through the aperture (Figure 1.3). Figure 1.3 Schematic representing standard impedance methodology. Blood is directed into two chambers. In one chamber, a lytic solution is used to obtain the WBC count by evaluating bare nuclei and measuring the hemoglobin released from erythrocytes. The second chamber contains isotonic solution and an aperture of limited size through which erythrocytes and platelets are enumerated. Failure of RBCs to lyze may result in their being counted as WBCs, thereby falsely increasing the WBC count. Similarly, large platelet aggregates may be erroneously counted as WBCs, resulting in spuriously low platelet and high WBC counts. Very large platelets may be miscounted as erythrocytes. Centrifugal analyzers operate by taking quantitative measurements on the cell layers below and within the buffy coat. The quantitative buffy coat (QBC) VetAutoread™ (IDEXX Laboratories Inc., Westbrook, ME) is an example of a centrifugal hematology analyzer. Granulocytes, mononuclear cells (monocytes and lymphocytes), erythrocytes, and platelets are separated into layers in an enlarged microhematocrit‐like tube using a cylindrical float to further expand the buffy coat layer. Cells separate into layers upon centrifugation according to relative density and fluorescent staining differentiates layers. Centrifugal analyzers can also provide fibrinogen concentrations by re‐reading the sample after incubating in a precipitator. Only the spun hematocrit is measured with centrifugal analyzers. Since erythrocyte counts are not determined, the MCV cannot be calculated. The Hb can be estimated assuming a constant relationship between hematocrit and Hb. From Hb and hematocrit, MCHC can be calculated. Estimated WBC counts are obtained from the thickness of layers by assuming an average cell size. Laser hematology analyzers generate both cell counts and differentials using light scatter. Single cells pass through a laser beam and scatter light at forward and side angles from the cell, which is picked up by photoreceptors (Figure 1.4). Forward, right‐angle, and side light scatter represent cell size and complexity. While this technology affords the opportunity to generate leukocyte differentials, in general there is not good precision with differential leukocyte counts [7, 8]. The presence of band neutrophils, toxic change or reactive lymphocytes can result in poor separation between leukocyte groups, adversely affecting the instrument differential (Figure 1.5). A manual differential from a blood film is still recommended to verify instrument differentials. Examples of POC hematology analyzers using light scatter are the ProCyte® and LaserCyte® (IDEXX) and ElementHT5® (Heska). The majority of in‐clinic chemistry analyzers are based upon dry reagent technology, which uses reflectance photometry. Similar to absorbance photometry, a chemical reaction (occurring within a dry fiber pad or multilayer film) results in a product that absorbs a portion of the light that illuminates it. The remaining reflected light reaches a photodetector that measures its intensity relative to the original illuminating light or a reference surface. There is an inverse relationship between reflected light (transmittance) and absorbance, where T is the percent transmittance (Equation 1.1). Analyzers will convert transmittance into absorbance because of the linear relationship between concentration and absorbance. Thus, concentration can be directly calculated from the absorbance. Figure 1.4 Schematic representing the principle of hematological analysis using laser methodology. Light passing directly through the cells (forward scatter; FSC) and light deflected 90° (side scatter; SSC) is captured by detectors. FSC and SSC correspond to cell size and complexity, respectively. Complexity refers to the character of the cytoplasm (e.g., presence or absence of granules). Fluorescence detectors capture fluorescence from dyes that stain RNA, myeloperoxidase or reticulum to differentiate leukocytes or to count reticulocytes. Figure 1.5 Laser‐generated leukocyte differentials from the ProCyte Dx POC hematology analyzer (Idexx). The scatterplot is based upon side scatter (granularity) and fluorescence from a fluorescent polymethine dye that stains nucleic acids. (a) Scatterplot from a healthy horse. Neutrophils have the least amount of cytoplasmic RNA, thus are located at the base of the y‐axis. (b) Scatterplot from a horse with toxic change in neutrophils and a left shift to band neutrophils. Neutrophils with toxic change and bands both have increased RNA content relative to normal mature neutrophils. Note how the increased RNA staining causes the neutrophil plot area to move upwards on the y‐axis, blending into the lymphocyte region.
1
General Laboratory Medicine
1.1 Introduction to Laboratory Medicine
1.2 Preanalytical Factors
1.3 Basic Hematological Techniques
1.3.1 Packed Cell Volume and Plasma Evaluation: Disease and Artifacts
1.3.2 Protein Measurement by Refractometer
1.4 Point‐of‐Care Testing
1.4.1 Hematology Analyzers
1.4.1.1 Impedance Technology
1.4.1.2 Centrifugal Hematology Analyzers
1.4.1.3 Laser Technology
1.4.2 Clinical Chemistry Analyzers
1.4.2.1 Dry Reagent Analyzers
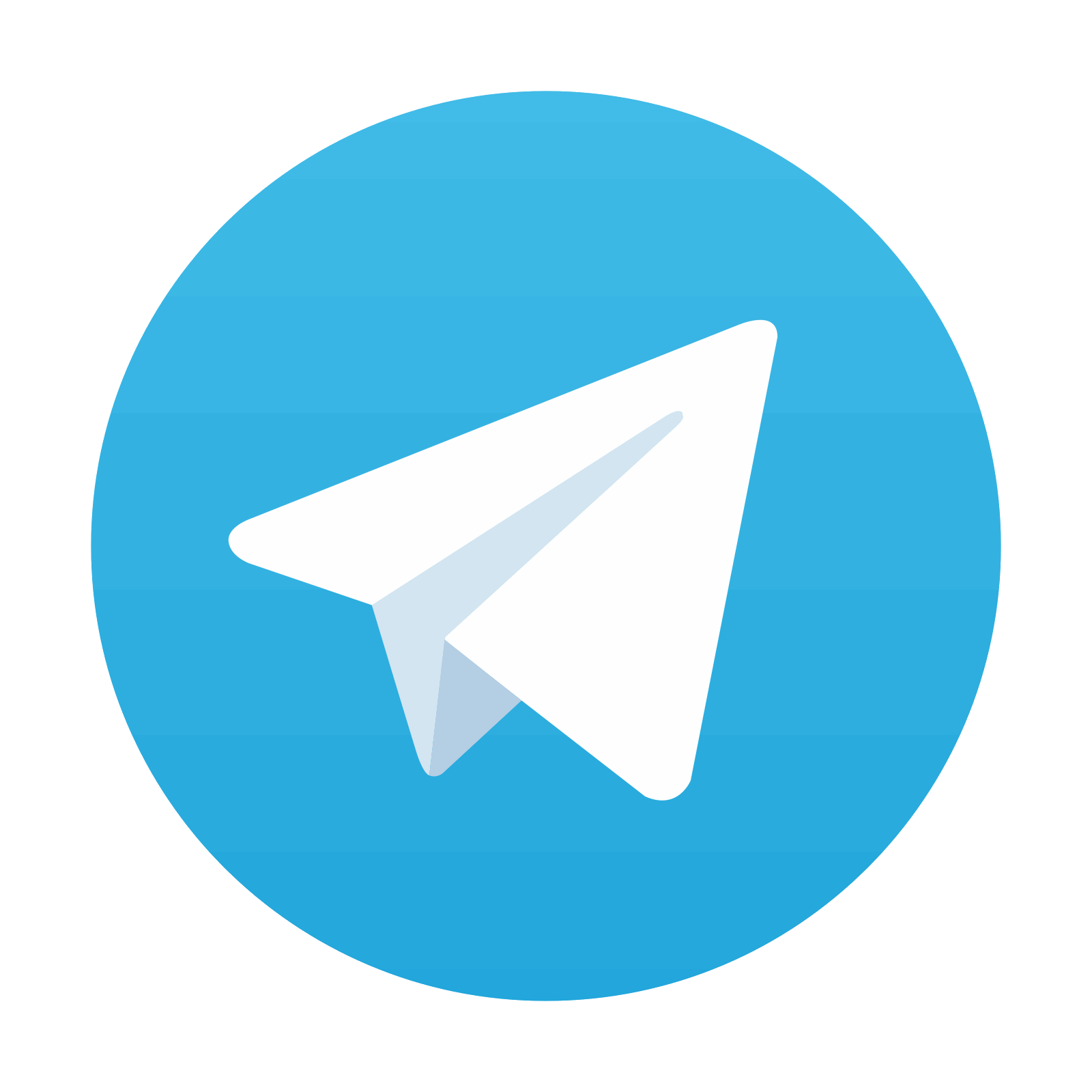
Stay updated, free articles. Join our Telegram channel

Full access? Get Clinical Tree
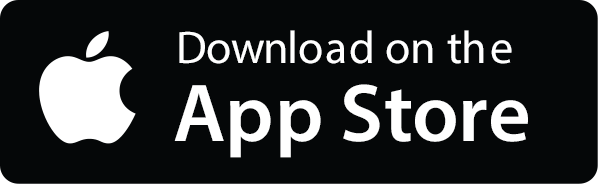
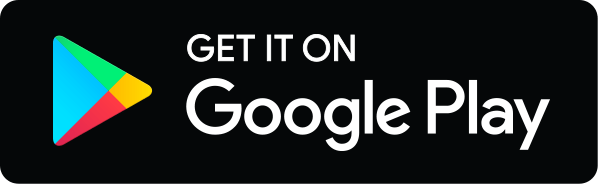